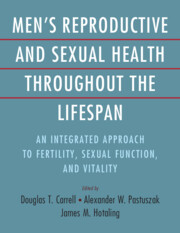
Book contents
- Men’s Reproductive and Sexual Health throughout the Lifespan
- Men’s Reproductive and Sexual Health throughout the Lifespan
- Copyright page
- Contents
- Contributors
- Preface
- Section 1 An Introduction to Men’s Health Care
- Section 2 The Biology of Male Reproduction and Infertility
- Section 3 Clinical Evaluation and Treatment of Male Infertility
- Section 4 Laboratory Evaluation and Treatment of Male Infertility
- Section 5 Medical and Surgical Management of Issues of Male Health
- Index
- References
Section 2 - The Biology of Male Reproduction and Infertility
Published online by Cambridge University Press: 06 December 2023
- Men’s Reproductive and Sexual Health throughout the Lifespan
- Men’s Reproductive and Sexual Health throughout the Lifespan
- Copyright page
- Contents
- Contributors
- Preface
- Section 1 An Introduction to Men’s Health Care
- Section 2 The Biology of Male Reproduction and Infertility
- Section 3 Clinical Evaluation and Treatment of Male Infertility
- Section 4 Laboratory Evaluation and Treatment of Male Infertility
- Section 5 Medical and Surgical Management of Issues of Male Health
- Index
- References
- Type
- Chapter
- Information
- Men's Reproductive and Sexual Health Throughout the LifespanAn Integrated Approach to Fertility, Sexual Function, and Vitality, pp. 23 - 96Publisher: Cambridge University PressPrint publication year: 2023