The terminology “Specific Language Impairment” (SLI), referring to children with oral language impairment for no obvious reason, was recently replaced by the term “Developmental Language Disorder” (DLD) (Bishop et al., Reference Bishop, Snowling, Thompson and Greenhalgh2017). This diagnosis, observed in 5–10% of the population (Law et al., Reference Law, McKean, Murphy and Thordardottir2019), includes children with severe and persistent language disorders that interfere with their communication in daily life and/or affect their schooling in the absence of a specific biomedical condition (such as Down syndrome). These two inclusion factors (severity and persistence) were already present in the original SLI terminology (Leonard, Reference Leonard2014), but it was the exclusion criterion referring to the specificity of the disorder that was problematic. Indeed, the new terminology better reflects the clinical reality of comorbidity associated with DLD, as language deficits observed in DLD are often associated with difficulties in other realms, including executive functions such as sustained attention, working memory (WM), inhibition, and attention shifting (Ebert & Kohnert, Reference Ebert and Kohnert2011; Kapa & Plante, Reference Kapa and Plante2015; Kapa et al., Reference Kapa, Plante and Doubleday2017). Considering the various interconnections between language and other higher cognitive functions, Tomas and Vissers (Reference Tomas and Vissers2019) suggest that DLD be treated as a complex neuropsychological syndrome in which language delays would be explained by other neuropsychological deficits.
Among the deficits reported for this population are impairments in WMFootnote 1 . These weaknesses can be revealed via simple-span tasks that require the maintenance and recall of verbal information, such as nonword repetition, forward digit span, and serial word order span (Archibald & Harder Griebeling, Reference Archibald and Harder Griebeling2016; Ellis Weismer et al., Reference Weismer, Evans and Hesketh1999; Gathercole & Baddeley, Reference Gathercole and Baddeley1990; Hick et al., Reference Hick, Botting and Conti-Ramsden2005; Montgomery & Evans, Reference Montgomery and Evans2009), or via complex-span tasks that require processing while maintaining stored information, such as listening span, counting span, or backward digit span (Archibald & Gathercole, Reference Archibald and Gathercole2006; Delage & Frauenfelder, Reference Delage and Frauenfelder2020; Hoffman & Gillam, Reference Hoffman and Gillam2004; Montgomery, Reference Montgomery2000). These well-known WM deficits in DLD have been integrated into various theories accounting for the condition (Conti-Ramsden et al., Reference Conti-Ramsden, Ullman and Lum2015; Evans & Brown, Reference Evans, Brown, Small and Hickok2016; Gillam & Hoffman, Reference Gillam, Hoffman, Verhoeven and van Bolkom2003; Kail, Reference Kail1994; Nicolson & Fawcett Reference Nicolson and Fawcett2007; Ullman & Pierpont, Reference Ullman and Pierpont2005).
Archibald and Gathercole (Reference Archibald and Gathercole2006) revealed that impairments in WM observed in children with DLD (aged 7 to 11 years) in both simple and complex span tasks not only cooccur but even exceed the criterial language deficits that are characteristic of the disorder. Performance in visuospatial WM, on the other hand, gives more mixed results, with difficulties either not emerging at all (Baird et al., Reference Baird, Dworzynski, Slonims and Simonoff2010; Ellis Weismer et al., Reference Weismer, Davidson, Gangopadhyay, Sindberg, Roebuck and Kaushanskaya2017; Petruccelli et al., Reference Petruccelli, Bavin and Bretherton2012) or being restricted to only subgroups of children with DLD (Archibald & Gathercole, Reference Archibald and Gathercole2006; Henry & Botting, Reference Henry and Botting2017; Vugs et al., Reference Vugs, Hendriks, Cuperus and Verhoeven2014). The observations that limitations in verbal (but not necessarily visual) WM are common to the majority of children with DLD could help to explain their language deficits. In line with this view, Baird and colleagues (Reference Baird, Dworzynski, Slonims and Simonoff2010) assessed the WM performance of three groups of children aged 6 to 16 years and revealed significant verbal WM deficits in the first group with DLD (N = 51), more subtle WM deficits in the second group with a history of resolved language impairment (N = 13), and an absence of WM deficits in the third group with no history of language impairment (N = 26).
Working memory and language in TD and DLD
The relation between WM and language has been extensively studied, both in typical and atypical language development, as well as in second language acquisition (e.g., Mackey and Sachs, Reference Mackey and Sachs2012). In typical development (TD), research has established clear links between WM and lexical acquisition (Leclercq & Majerus, Reference Leclercq and Majerus2010; Majerus et al., Reference Majerus, Poncelet, Greffe and Van der Linden2006) as well as between WM and syntax in school-aged children (Adams & Gathercole, Reference Adams and Gathercole2000; De Abreu et al., Reference De Abreu, Gathercole and Martin2011; Delage & Frauenfelder, Reference Delage and Frauenfelder2019; Ellis Weismer et al., Reference Weismer, Davidson, Gangopadhyay, Sindberg, Roebuck and Kaushanskaya2017; Finney et al., Reference Finney, Montgomery, Gillam and Evans2014; Montgomery et al., Reference Montgomery, Magimairaj and O’Malley2008; Poll et al., Reference Poll, Miller, Mainela-Arnold, Adams, Misra and Park2013; Willis & Gathercole, Reference Willis and Gathercole2001). These associations have been highlighted with simple span tasks (e.g., nonword repetition tasks and forward digit span in children aged 4–5 years, Willis & Gathercole Reference Willis and Gathercole2001) as well as with complex span tasks (e.g., listening span task in children aged 6–13 years, Poll et al., Reference Poll, Miller, Mainela-Arnold, Adams, Misra and Park2013). In a study combining the two types of span, Delage and Frauenfelder (Reference Delage and Frauenfelder2019) found a predictive relation between the two composite WM scores (simple and complex spans) and different measures of syntax: repetition, comprehension, and spontaneous production of complex sentences, in 48 TD children aged 6–12 years.
The significant difficulties with WM experienced by children with DLD persist into adolescence (Ellis Weismer et al., Reference Weismer, Plante, Jones and Tomblin2005), as do their language deficits (see Conti-Ramsden & Durkin, Reference Conti-Ramsden and Durkin2008), particularly for complex syntax (Nippold et al., Reference Nippold, Mansfield, Billow and Tomblin2009; Tuller et al., Reference Tuller, Delage, Monjauze, Piller and Barthez2011, Reference Tuller, Henry, Sizaret and Bathez2012). Such syntactic difficulties are accounted for by the Derivational Complexity Hypothesis (DCH, Jakubowicz, Reference Jakubowicz2011; Jakubowicz & Strik, Reference Jakubowicz and Strik2008; Jakubowicz & Tuller, Reference Jakubowicz, Tuller and Ayoun2008)Footnote 2 . This metric predicts that children with DLD should encounter difficulties with noncanonical sentences, which have been borne out by studies on object clitic pronouns (see, for example, Tuller et al., Reference Tuller, Delage, Monjauze, Piller and Barthez2011), object relatives (1) (Adani et al., Reference Adani, Stegenwallner-Schütz, Haendler and Zukowski2016; Delage & Frauenfelder, Reference Delage and Frauenfelder2020), object questions (2) (Friedmann & Novogrodsky, Reference Friedmann and Novogrodsky2011; Jakubowicz, Reference Jakubowicz2011), and passive sentences (3) (Marinis & Saddy, Reference Marinis and Saddy2013; Montgomery & Evans, Reference Montgomery and Evans2009). All these different constructions share the underlying property of implying a noncanonical word order instead of the French default order: subject–verb–object. More specifically, in all examples, the (logical) object moves to a higher position, a syntactic movement symbolized by the arrows and resulting in different word orders.
The DCH also links mastery of grammatical structures to cognitive factors: “Jakubowicz (2004, 2005) proposed that (ab)normal language build up is affected by developmental constraints such as the capacity of working memory, that are sensitive to the computational complexity of the derivation” (Jakubowicz & Strik, Reference Jakubowicz and Strik2008: 106). More computational complexity would be involved in the structures above, where the movement operation would lead to an overload of WM capabilities. The immature WM systems of young TD children would thus yield a grammar characterized by the presence of short and simple, canonical sentences. In children and adolescents with DLD, limitations in WM persist, potentially explaining reports of their avoidance of noncanonical structures (see above) and links between performance on these complex structures with WM.
To take the concrete example of an object relative clause, its mastery requires 1) remembering the object until reaching the position of gap, i.e., the trace/copy left by the moved element, 2) while processing the new verbal stimuli (= words) to be integrated into the sentence, and then 3) proceeding to the correct interpretation of the sentence by linking the verb to its different arguments (Gibson, Reference Gibson1998). WM resources are thus plausibly solicited by such structures, which would therefore pose challenges to children with a condition displaying memory deficits. This reasoning has found empirical support from a series of studies. For instance, in young TD children, Arosio and colleagues (Reference Arosio, Guasti and Stucchi2011) showed that digit span performance relates to the comprehension of object relative clauses. Similarly, Bentea and colleagues (Reference Bentea, Durrleman and Rizzi2016) report that WM measures (assessed by forward and backward digit spans) correlated with the comprehension of object Wh-questions and object relative clauses in French-speaking children aged 5 years. In older children (aged 7 to 9 years), this link was limited to the most complex constructions (involving intervention effects in the presence of two NPsFootnote 3 ). Still on object questions and object relative clauses, Stanford and Delage (Reference Stanford and Delage2019) found that comprehension of English-speaking children with specific learning disorder (mainly with dyslexia) aged 7 to 11 years correlated with their performance on backward digit span. Finally, Frizelle and Fletcher (Reference Frizelle and Fletcher2015) have also identified a strong relationship between the repetition of relative clauses, varying in complexity, and WM measures in 35 English-speaking children with DLD aged 6–7 years. More precisely, complex-span tasks (including listening recall, counting recall, and backward digit span) were particularly associated with the more complex relative clauses, like object relatives. In a similar study, Riches and colleagues (Reference Riches, Loucas, Baird, Charman and Simonoff2010) examined several WM measures (nonword repetition, digit span, and listening span) as well as the capacity to repeat relative clauses in adolescents with DLD aged 14 to 16 years. Their results reveal that performance on repetition of such complex sentences was significantly correlated to their scores obtained on WM tasks.
Other constructions involving syntactic movement, such as passives, were also found to be linked to WM in 25 English-speaking TD children aged 7 years with a listening recall task (Marinis & Saddy, Reference Marinis and Saddy2013) and in adults with a composite measure of WM-capacity index (Sung et al., Reference Sung, Yoo, Lee and Eom2017). Mastery of such complex grammatical constructions arguably requires storing and manipulating verbal sequences since “these structures require storing of the NPs of the sentence in memory before syntactically and semantically integrating with the verb phrase thanks to the cue provided by the passive morphology” (Durrleman et al., Reference Durrleman, Delage, Prévost and Tuller2017: 8). Montgomery and colleagues (Reference Montgomery, Magimairaj and O’Malley2008) compared the role of simple spans (assessed via nonword repetition) and complex spans (assessed via listening span) in the comprehension of a variety of both simple and complex sentences in children aged from 6 to 12 years. Complex structures assessed were passives or sentences involving binding dependencies (with either reflexive or accusative pronouns), while simple sentences contained no movement or dependency. A correlation emerged between the comprehension of complex sentences and performance on complex spans, but no correlation was observed between the comprehension of simple sentences and simple spans. Furthermore, regression analyses showed that complex-span results explained 30% of the variance in the comprehension of complex sentences. These findings were replicated for children with DLD (Montgomery & Evans, Reference Montgomery and Evans2009).
Syntactic movement is not the only source of syntactic complexity for children with DLD. These children also have difficulty with complex sentences that include embedding (Delage et al., Reference Delage, Monjauze, Hamann and Tuller2008; Hamann & Tuller, Reference Hamann and Tuller2014; Scheidnes & Tuller, Reference Scheidnes and Tuller2014). For example, Tuller et al. (Reference Tuller, Henry, Sizaret and Bathez2012) show, through a spontaneous language analysis conducted in 18 adolescents with DLD aged 11 to 16 years, that they produced shorter sentences with fewer embedded sentences than 8-year-old TD children. Few studies have focused on this aspect with respect to WM. However, in young TD children aged 3–5 years, Adams and Gathercole (Reference Adams and Gathercole2000) and Willis and Gathercole (Reference Willis and Gathercole2001) have shown that children with better WM skills (assessed by word, nonword, and number repetition tasks) produced and repeated longer and more complex sentences than children with poorer memory skills. Mastering the production of complex sentences requires dealing with structures in which the different verbs must be related to several arguments and which include tense concordance phenomena, while keeping in mind the previous words of the sentence. In the case of multiple embedding as in (4), the implication of WM capabilities appears even more obvious, as Kimball (Reference Kimball1973) postulated that the number of incomplete clauses that must be stored in memory in these contexts crucially influences processing load.

Tuller et al. (Reference Tuller, Henry, Sizaret and Bathez2012: 165) explicitly linked both the level of embedding and the number of operations involved in a derivation to WM: “These two properties both appear to be related to demands on memory: building syntactic structure while keeping in mind already built syntactic structure (particularly when a new clause is involved), linking antecedents and gaps, comparing features for agreement”. This approach is in line with the Jakubowics’s DCH approach previously mentioned which aims to account for the syntactic impairment in DLD by linking domain-specific syntactic principles with domain-general behavioral variables. Even if these approaches are firmly grounded in the nativist tradition, they acknowledge that cognitive capacities, and especially WM, play a role in language acquisition, and more precisely that they are recruited to help develop domain-specific language predispositions. Chomsky himself acknowledged this (Reference Chomsky2005: 12): “It could be that unbounded Merge, and whatever else is involved in [Universal Grammar], is present at once, but only manifested in limited ways for extraneous reasons (memory and attention limitations and the like).” In more purely cognitive approaches to language acquisition, such as usage-based models (e.g., Tomasello, Reference Tomasello2000, Reference Tomasello2005, Reference Tomasello2009), language acquisition reflects the maturation of cognitive capacities and processes. From this point of view, children are seen as using their general and social-cognitive skills to build up an inventory of linguistic constructions through imitation of the language(s) they hear around them (Tomasello, Reference Tomasello2000). Since language acquisition is assumed to interact closely with the gradual development of other cognitive processes within this framework, limitations in cognitive skills, such as WM, should play a nonnegligible role in language outcomes.
Tuller and colleagues’ hypothesis was addressed by Zebib et al. (Reference Zebib, Tuller, Hamann, Abed Ibrahim and Prévost2019) who explored the performance of 23 bilingual children (6–8 years old) with DLD and 53 bilingual TD children of the same age in sentence repetition (with complex sentences varying in embedding and syntactic movement) and WM, using classical tasks of forward and backward digit spans. Results showed that sentence repetition was mainly linked to complex spans in DLD children, whereas it was predicted by simple spans in TD children, suggesting that the former rely more on their general processing abilities to repeat complex sentences that they do not yet master. In previous work that provided the basis for the current study, Delage and Frauenfelder (Reference Delage and Frauenfelder2020) also addressed these two complexity factors of embeddedness and syntactic movement by using more diverse syntax and memory tasks. Twenty-eight children with DLD (5–14 years old) and 48 TD children of the same age, all monolingual and francophone, were assessed for verbal WM skills through simple span tests (forward digit-span, serial memory, and nonword repetition) and complex span ones (backward digit span, counting span and running span), and for syntax via tests of comprehension and repetition of complex sentences, as well as via spontaneous language samples. Results confirmed the persistent deficits of the children with DLD in all tests of WM and complex syntax and, most importantly, a strong relationship between WM and syntactic complexity, even though differences in age and nonverbal intelligence were controlled for. More specifically, results for the children with DLD revealed a strong predictive link between the serial component of verbal WM and syntactic abilities, a relation that is not mediated by more general intellectual skills. In TD children, the involvement of complex spans (including counting span and running span) appeared stronger than that of simple spans to explain the variance of the results in complex syntax. The clinical implications of these results have given rise to the current investigation, which aims to evaluate the specific effects of training WM to enhance grammatical abilities in DLD. Indeed, given that the WM limitations observed in children with DLD predict their difficulties in complex syntax, integrating WM training in speech and language therapy for this population may be promising.
Working memory training
In Reference Morrison and Chein2011, Morrison and Chein showed that WM capacity can be expanded through targeted training, involving the repetition of tasks on specific mechanisms. Several studies have since examined the effects of WM training, most often with healthy adults, yielding variable results: they generally show an overall improvement in WM performance without necessarily long-term maintenance and very little transfer to other cognitive abilities (verbal abilities, identification of written words, etc., see Melby-Lervåg & Hulme, Reference Melby-Lervag and Hulme2013). The internal validity of these studies has been called into question by numerous scholars, who have thus encouraged the scientific community to conduct future research using more appropriate experimental designs and measures adapted to large cohorts of participants (Majerus, Reference Majerus2016; Melby-Lervåg & Hulme, Reference Melby-Lervag and Hulme2013, Reference Melby-Lervag, Redick and Hulme2016). Interestingly, when WM training focuses on populations with learning disabilities, results seem more promising, with short and long-term effects on WM and word decoding, as shown by the meta-analysis of Peijnenborgh and colleagues (Reference Peijnenborgh, Hurksc, Aldenkamp and Vlesabd2015). However, while WM training has been offered to various populations such as children with attention deficit hyperactivity disorder (ADHD) or learning disorders (Majerus, Reference Majerus2016), very few studies have focused on children with oral language difficulties. One that did is Holmes and colleagues (Reference Holmes, Butterfield, Cormack, van Loenhoud, Ruggero, Kashikar and Gathercole2015) which reported improved visuospatial skills in 12 children with poor language skills aged 8 to 11 years, following WM training by means of the Cogmed program. Vugs and colleagues (Reference Vugs, Knoors, Cuperus, Hendriks and Verhoeven2017) also proposed executive function training (including visuospatial WM, inhibition, and cognitive flexibility tasks) to 10 children with DLD aged 8 to 12 years. The authors observed a gain that seemed to be maintained on executive functions but did not test for possible language progression, while recognizing that: “If it is proven possible to improve [executive functions] in children with SLI by computerized training, this might also have an (indirect) effect on the linguistic skills of these children” (p. 16). Ebert and Kohnert (Reference Ebert and Kohnert2009) examined the language effects of processing speed and auditory memory training in two children with DLD aged 7 and 8 years. Results indicated that the participants made gains in processing speed and language abilities, including sentence formulation and grammatical morpheme production. Note, however, that such case studies do not allow the results to be generalized to a population as heterogeneous as DLD.
Aim and prediction of the present study
The current study focused on children with DLD and proposed a targeted training of verbal WM. The intensive training programs for WM that already exist on the market (e.g., the Cogmed or Jungle Memory programs) seem too broad to enhance the specific skills identified as underlying linguistic complexity, as they include a number of unrelated activities such as visuo-spatial memory. We thus developed Magic Memory (Delage et al., Reference Delage, Da Costa and Durrleman2017), a program aimed at training those precise aspects of WM that have been shown to predict performance in complex syntax, i.e., complex spans and serial memory (Delage & Frauenfelder, Reference Delage and Frauenfelder2019, Reference Delage and Frauenfelder2020). This program has shown its effectiveness on the ability of children with DLD to produce accusative clitic pronouns (Stanford et al., Reference Stanford, Durrleman and Delage2019), structures that, it should be recalled, include syntactic movement. Specifically, 26 children with DLD aged 5 to 12 years who had completed the WM training program were compared to 17 children with DLD receiving an alternative (scholastic) training. The results showed that after 12 hours of effective training in WM, the first group made significant progress in the production of object clitics, while the performance of the control group remained stable. Furthermore, the program had direct effects with an improvement in WM capacities in children with DLD. These direct effects were also observed in 16 children of the same age with typical language development.
The objective of the present research is to extend the cohort of DLD and TD children benefiting from intensive WM training and to explore whether transfer effects can be found for syntactic structures varying in the number of (i) syntactic derivations and (ii) embedded clauses. Whereas our previous study aimed at eliciting a single syntactic structure (i.e., accusative clitics), we propose here to explore the repetition of varied syntactic structures, consisting of simple sentences and relative clauses, for which the factors “syntactic movement” and “embedding” have been conscientiously manipulatedFootnote 4 . As it was the case for elicitation tasks of accusative clitics in romance language, sentence repetition tasks have been shown to be good clinical markers of DLD (e.g., Archibald & Joanisse, Reference Archibald and Joanisse2009; Armon-Lotem & Meir, Reference Armon-Lotem and Meir2016; Zebib et al., Reference Zebib, Tuller, Hamann, Abed Ibrahim and Prévost2019), even in young children (Everitt et al., Reference Everitt, Hannaford and Conti-Ramsden2013). This type of task assesses multiple components such as expressive phonology, lexical knowledge, morphosyntactic skills, as well as verbal WM (Alloway & Gathercole, Reference Alloway and Gathercole2005; Polišenská et al., Reference Polišenská, Chiat and Roy2015; Tuller et al., Reference Tuller, Hamann, Chilla, Ferré, Morin, Prevost and Zebib2018). To focus on syntax and then to minimize the impact of the other skills, we proceeded to different adjustments:
-
– We contrasted simple and complex sentences that contained the same number of syllables, to counterbalance the effects of verbal WM;
-
– We considered measures for which children respect or not the expected structure and level of embedding, whatever the lexicon they used, to neutralize effects of lexical selection/retrieval;
-
– We did not penalize any phonological deformations, to overcome the effects of imprecise phonological representations or articulatory deformations.
More precisely, our predictions are as follows:
-
1. As in our previous study, we expect that children (both TD and DLD) following the WM training will improve their capacities in WM, i.e., direct effects, and these improvements will be observed in tasks beyond those trained by the program. As for children following the control training, we predict no significant improvement in WM, which would prove the specificity of our WM training. These effects have already been found in our earlier study, but we think that replicating them with a larger cohort of participants would strengthen our conclusions and confirm the effectiveness of our program. This seems to be of particular importance as such effectiveness of WM training is still being debated in the literature (Melby-Lervag & Hulme, Reference Melby-Lervag and Hulme2013; Melby-Lervag et al., Reference Melby-Lervag, Redick and Hulme2016). Moreover, we aim to explore another aspect not previously addressed, namely, whether the observed effects can be sustained over time through delayed posttest.
-
2. Next, and more importantly, we also expect to demonstrate the presence of transfer effects, with children trained in WM also improving their ability to accurately repeat complex sentences?. Here again, we predict that improvements in syntax will not be observed in children in the control (scholastic) group who would not have undergone any specific training to improve this area. As for differences between TD and DLD children in the WM training, we expect a modest progression in the former compared to the latter, since the syntactic level of TD children would be logically higher than that of DLD children, leaving a smaller margin for progression.
-
3. Finally, we will be able to explore to which extent transfer effects are modulated by syntactic complexity factors, given our manipulation of the degree of complexity of the structures to be produced. We predict that the performance of children in the WM training group will improve for the most complex structures, which are hypothesized to particularly tax WM resources, and that this effect will be less obvious for simpler syntactic structures.
Method
Participants and inclusion criteria
A total of 88 native French-speaking children (52 with DLD and 36 with TD) aged 6 to 12 years took part in this study. This age range was chosen since it corresponds to the ages of children for whom a predictive relationship between WM and syntax had previously been identified (Delage & Frauenfelder, Reference Delage and Frauenfelder2019, Reference Delage and Frauenfelder2020). Thirty-two children with DLD (‘DLDWM’, M age = 9;0) followed the WM training entitled Magic Memory (MM) and were compared to 20 children with DLD matched on age (‘DLDSQ’, M = 9;3) who followed an alternative, scholastic, training program called Squla. TD children were also divided between WM training (‘TDWM’, N = 18, M age = 8;5) and alternative training (‘TDSQ’, N = 18, M age = 8;4). Assignment to the different training groups was random. Even though age matching was only done for each cognitive group (DLD, TD), the four groups did not significantly differ for age, F(3, 84) = 1.3, p = .28. Table 1 details the general characteristics of the different groups (gender, age range, mean age, and bilingualism). As for inclusion criteria, we only recruited monolingual (N = 74) or simultaneous bilingual (N = 14) French-speaking children, hence only native speakers of French participated.
Table 1. Characteristics of experimental (WM) and control (Scholastic) groups
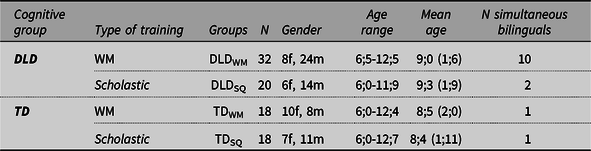
Cognitive groups were based on children having received a diagnosis of language impairment by speech and language therapists, hence being assigned to the DLD group, or an absence thereof for TD children. More specifically, children with DLD had been tested by clinicians with standardized tasks (e.g., the EXALANG 5-8, Thibaut et al., Reference Thibault, Helloin and Croteau2010), and we required that these demonstrate clear syntactic impairment, since DLD does not always include such deficits (Friedman & Novrogrodsky, Reference Friedmann, Novogrodsky, Gavarró and João Freitas2008). We also made sure with the speech-language therapists that these children did not present any known differentiating condition (as defined by the CATALISE group, Bishop et al., Reference Bishop, Snowling, Thompson and Greenhalgh2017), such as brain injury, aphasia, cerebral palsy, sensorineural hearing loss, autism spectrum disorder, or intellectual disability. TD children had normal levels of language (i.e., no language therapy) and were functioning without special difficulties in their age-appropriate classroom, according to their parents. Different standardized tests were also used to refine our inclusion criteria. First, we ensured that both groups performed above the 10th percentile in a nonverbal reasoning task (Raven’s Colored Progressive Matrices, Raven & Court, Reference Raven and Court1998), thereby excluding any risk of intellectual disability. Then, we conducted a standardized assessment of expressive grammar for all children with a subtest of the BILO-3C battery (Khomsi et al., Reference Khomsi, Khomsi, Parbeau-Guéno and Pasquet2007) as well as of WM via a battery which combined three simple-span tasks and three complex-span tasks (Boutard & Gatignol, Reference Boutard and Gatignol2015). To be definitely included in the study, children with DLD had to obtain a score of at least 1.25 SD below age-specific norms for the score in grammar as well as such delay for a minimum of three of the six WM tasksFootnote 5 . As for TD children, we only retained participants who scored with a score above −1 SD in expressive grammar and had no more than two of the six WM scores that were inferior to −1 SDFootnote 6 . In this manner, we ensure that children with DLD had clear deficits in WM and in syntax, whereas TD children showed preserved capacities. Table 2 presents the mean standard deviations obtained by the different groups for these standardized tasks. Two composite scores were calculated for WM tasks, grouping simple-span, and complex-span tasks. Using independent t-tests, the two groups of DLD children (DLDWM versus DLDSQ) did not significantly differ for nonverbal reasoning (p = .65), expressive grammar (p = .75) or WM, whether for simple (p = .11) or complex spans (p = .09)Footnote 7 . The same pattern was observed for TD children (respectively p = .35, p = .41, p = .77, p = .37). As expected, children with DLD, as a whole, differed significantly from TD children for expressive grammar, t(86) = −12.2, p < .001, d = 2.76, and for WM measures, for simple-span, t(86) = −11.3, p < .001, d = 2.52, and complex-span tasks, t(86) = −8.3, p < .001, d = 1.7. These two groups also differed for nonverbal reasoning, t(86) = −7.4, p < .001, d = 1.63, with DLD children scoring lower than TD ones. This difference can be explained by the fact that children with DLD, even though they display performance within the norm, typically perform lower than age-matched controls on nonverbal tasks (Leonard, Reference Leonard2014).
Table 2. Standardized assessments: nonverbal reasoning, expressive grammar, and working memory
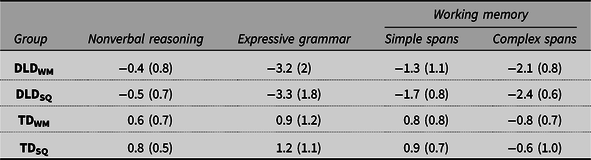
Of the 14 bilingual children, 12 have a diagnosis of DLD. We compared the performance of these 12 children to that of the other (monolingual) children with DLD (N = 40). These two groups did not significantly differ for age (p = .50), nor for expressive grammar (p = .08), nonverbal reasoning (p = .05) or complex-span tasks (p = .46). The only difference which emerged was in simple-span task where bilingual children outperformed monolinguals, t(50) = 3.0, p = .004, d = 1.03. Note, however, that the effect size is reduced, as is the sample of bilinguals, and that the difference seems to be due to the particularly high performance of some bilingual children (see also Marini et al., Reference Marini, Eliseeva and Fabbro2019 for similar effects in bilinguals). Ages and standardized scores of monolingual and bilingual children with DLD are provided in Appendix A.
Material and methodology
General procedure
For all of the trained participants, we established a baseline via pretests, conducted one week before the first training session. These tests evaluated various WM and syntactic skills. One week after the last training session, posttests evaluated these same skills using different (but matched) items, in order to avoid a learning effect. Hence, we obtained two different test versions, A and B, in which words were controlled and matched for length end frequency. The order of these versions was randomized between participants in order to have half of the participants passing version A in pretests and version B in posttests, and the opposite for the other half. These measures are used to identify potential progression of trained participants and thus to assess the immediate effects of training. Three months after the posttests, we conducted another session of posttests with DLD participants (N = 12) who had followed WM training, who were available at this moment and whose parents agreed to participate in this third testing phase. For these delayed posttests, participants were readministered the version (A or B) they had completed at the pretest, 5 to 6 months earlier, which was sufficient to avoid potential retest effects.
The training sessions consisted of two different training programs. As previously mentioned, the target “Magic Memory” (MM) training aimed to improve WM capacities with different exercises implying simple and complex verbal spans. The alternative training, entitled “Squla,” focused on scholastic skills with exercises adapted to the participants’ school level. The aim of this control training was to ensure that any potential gains in WM and/or syntax following the training period were indeed related specifically to the WM training program rather than to maturation or global cognitive stimulation. More specifically, we predicted that the progression of the group benefiting from WM training would be better, both in WM and syntax, than that of children who had followed the alternative training. Both training sessions were offered with the same duration and format: each participant completed three training sessions per week, with each session lasting 30 minutes, for 8 weeks, which constitutes a total of 12 hours per participant. Both programs were provided in computerized format, either on iPads or computers, depending on the material available at children’s homes. For each activity of both types of training, the task difficulty was adapted to match the child’s performance level. Frequent positive feedback was also included in the programs, in order to boost children’s motivation. Figure 1 outlines the overall experimental design of the study.
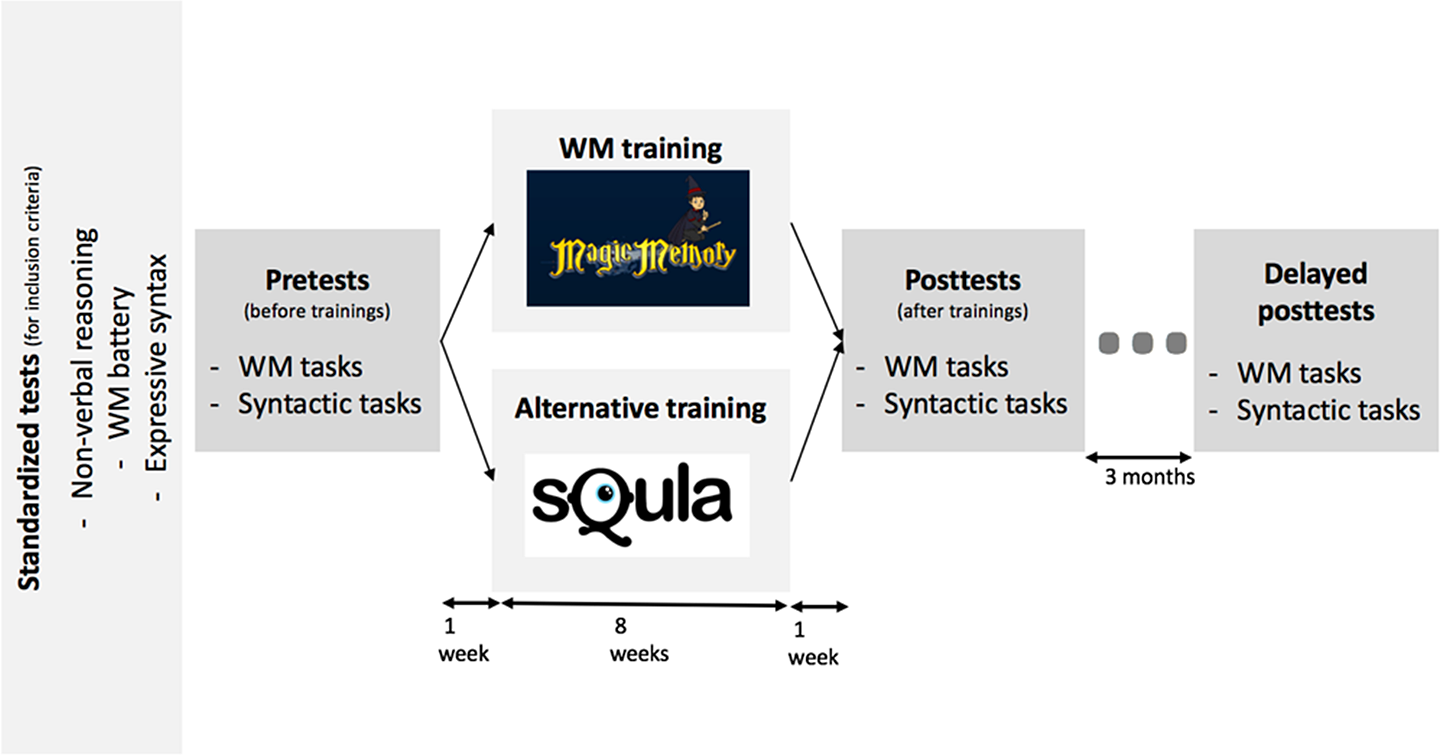
Figure 1. Experimental design.
The training sessions were carried out at the home of the participant under the supervision of parents and graduate students in French-speaking Switzerland, as well as in France. Students contacted parents on a weekly basis to ensure that the assigned training program was being appropriately followed and visited participants two to three times a month to track the progress of the training regime. Approval for the research was obtained from the Ethics Committee of the University of Geneva and was also declared at ‘La Commission Nationale de l’Informatique et des Libertés (CNIL)’ in France. All parents received detailed information on the study and signed the consent form to approve the participation of their child.
Pre- and post-training tests: Working memory
Before and after the training phase, children passed a series of tests assessing WM with three tasks for simple spans and two tasks for complex spans (Table 3). As said before, we used two paired versions (A and B) for each task. Standard scores were derived for the individual tests and composite scores were calculated by averaging standard scores for each set of tests (simple-span and complex-span tasks).
Table 3. Pre- and post-training tests: Working memory tasks

Pre- and post-training tests: Syntax
Sentence repetition task
To test the ability of our participants to repeat syntactically complex sentences, we adapted a sentence repetition task created by Delage and Frauenfelder (Reference Delage and Frauenfelder2019)Footnote 8 that required participants to immediately repeat sentences read to them by the experimenter. The task was composed of 23 sentences: 8 syntactically simple control sentences and 15 syntactically complex experimental sentences. All sentences, both control and experimental, contained 14 syllables. As for experimental sentences, syntactic complexity was measured by the number and type of syntactic movement operations required to derive the structure (short- and long-distance phrasal movement or head movement) and the number of embedded clauses within the structure (1, 2, or 3), as shown in Table 4. There were thus simple sentences, without any embedding, which were intercalated (one simple sentence after two complex sentences) with complex sentences. The first relative clauses only contained one degree of embedding, followed by sentences with two and three degrees of embedding. For sentences with one degree of embeddingFootnote 9 , there were three subject relatives and six object relatives (3 without subject−verb inversion, 3 with such inversion). For the second and third levels of embedding, there was one subject relative and two object relatives (1 without subject−verb inversion, 1 with such inversion) for each level. Appendix B presents the set of sentences for the two versions (A and B), which were matched for length, syntactic structure and frequency.
Table 4. Sentence repetition task
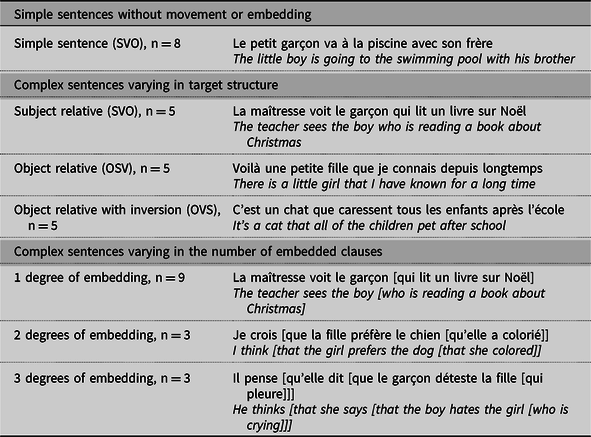
As the task progressed, the structures the children were asked to repeat became increasingly more complex. Three measures were considered: 1) the number of syllables that were correctly repeated, without considering the phonetic deformations, 2) the respect of the target structure (e.g., an object relative), and 3) the respect of the expected degree of embedding (with one, two, or three embeddings). For these two last measures, only the syntactic properties are considered; hence, the child obtained the point if s/he produced the correct structure and/or the expected level of embedding even if s/he changed the lexicon. Appendix C provides examples illustrating this scoring. After initial transcription and coding, all transcriptions were checked, and corrected if necessary, by two different experts.
Working memory training: Magic Memory
For the target WM training, we created a new training program called Magic Memory. This is an adaptive type of training where the level of difficulty gradually increases according to the progress made by the participants. Figure 2 (screenshots) illustrates the design of the program and the different types of feedback. In the Magic Memory training program, five activities were designed to train serial-order memory, WM updating, as well as dual-task processing. For the latter, the child must retain the order of familiar auditory stimuli while simultaneously performing a secondary task (a visual comparison of quantity task). The different activities, which were integrated and proposed in random order at each session, are detailed in Appendix D.

Figure 2. Magic Memory: Global design and examples of feedbacks.
Alternative scholastic training
The alternative training program was offered through the Squla online educational game platform (Squla Inc. 2017). This training program for scholastic skills was carefully selected from a variety of existing systems. The program targets scholastic skills, such as spelling, history-geography, English, or mathematical reasoning, using several educational games with multiple choice questions and playful reinforcers. We made sure that none of the proposed activities focused heavily on WM or complex syntax. In addition, we asked parents to vary the academic notions to be worked on. The activities were adapted to each child’s grade level. Trophies and positive feedback punctuated the different activities.
Results
Pretraining tests: Preliminary analyses
Before investigating whether or not our WM training program had been effective, we wanted to first ensure that there was a difference between our two cognitive groups (TD vs. DLD) but not between our two training groups (WM versus SQ) on our three main pretest measures: (i) composite simple span score, (ii) composite complex span score, and (iii) percentage of correctly repeated syllables in the sentence repetition task, see Table 5. Independent t-tests confirmed that the performance of our TD participants was indeed significantly better than that of our participants with DLD on our pretest measures (TDWM/SQ children outperformed DLDWM/SQ children) and that our two training groups contained participants with similar pretest abilities (DLD/TDWM children performed similarly to DLD/TDSQ children).
Table 5. Summary of composite WM scores and pretest syntax scores

*** p < .001
As for the sentence repetition task in particular, we proceeded to an examination of unexpected structures and error types which showed that 1) the dominant nonexpected structure consisted of producing simple sentences, without any embedding, instead of the target relative clause; 2) gender errors and omission/substitution of complementizers were the most frequent errors in both populations (TD/DLD). A more exhaustive description is provided in Appendix E.
Direct effects: Is there an improvement in WM tasks?
After verifying that variables met standard assumptions of normality and heterogeneity, we wanted to examine whether the individual training programs had differential effects on the WM tasks that had not been directly trainedFootnote 10 . In other words, was there an improvement from pre to posttest and if so, was this specific to the type of training program the participants had followed (WM versus SQ)? To begin with, repeated measures ANOVAs were run with time (pretest, posttest) as the within subjects variable and training type (WM, SQ) and cognitive group (DLD, TD) as the between subjects factors. Our first analyses used composite scores for simple and complex span and revealed a main effect of time (posttest > pretest) and a main effect of cognitive group (TD > DLD) for both measures, but no main effect of type of training for either simple or complex span, see Table 6. In other words, when composite scores were considered, the WM and SQ groups (TD + DLD) performed similarly, in both the pre and posttest phases.
Table 6. Summary of main effects and interactions for composite WM test measures

When the WM tests were analyzed individually, significant main effects of time (posttest > pretest) and cognitive group (TD > DLD) were observed for all five tests. In addition, for serial order word span there was a significant main effect of type of training, with children in the WM training group outperforming children in the SQ training group. For the other four tests, this effect was not significant, see Table 7.
Table 7. Summary of main effects and interactions for the individual WM test measures

Next, the repeated measures ANOVAs were used to investigate the effects that were of primary interest to us, mainly if the target training had a specific effect across the two cognitive groups (DLD, TD) on WM tasks when compared to the (SQ) scholastic training. In other words, did pre to posttest WM progress depend on training type? There was a statistically significant interaction effect of time by type of training for both composite simple and complex span (see Table 6). Post hoc Tukey HSD tests revealed that these effects were significant in both WM training groups, with composite posttest scores being significantly higher than composite pretest scores, as seen in Table 8. In the SQ training group, there was a significant difference between pre and posttest scores for the TD children for simple span, but not for complex span. While pre to posttest WM gains are unexpected for TDSQ children, it should be noted that the improvement for this particular group of participants is less statistically prominent than it is for DLD/TDWM children when the p-value and Cohen’s d are considered. No significant differences were found for DLDSQ children.
Table 8. Summary of pretest vs. posttest comparisons for the composite scores for simple and complex spans

*** p < .001; ** p < .01; * p < .05
Analyzing WM tests individually, a significant time by type of training interaction effect was observed for serial order word span, forward and backward digit spans (see Table 7). For counting span, this interaction was tendential and for nonword repetition it was not significant. Tukey HSD results confirmed that it was only the WM training groups that made significant pre to posttest progress on these individual WM measures, with DLDWM children demonstrating posttest performance that was significantly better for serial order word span, forward digit recall, nonword repetition, and backward digit recall. For TDWM children, there was significant improvement in serial order word spanFootnote 11 and forward digit recall. No significant progress was observed for any of the WM tests for the DLD/TDSQ children. These results are summarized in Appendix F.
The repeated measures ANOVAs were also used to provide insight about a potential two-way interaction between time (pretest/posttest) and cognitive group (DLD/TD) and a potential three-way interaction between time, cognitive group, and training type (WM/SQ), see Tables 6 and 7. With the exception of a significant time by cognitive group interaction for the serial order word span measure (TD group improving most), these analyses revealed no significant interaction effects. These results are understandable because pre to posttest improvements on the WM measures were not limited to DLDWM children and were also observed in TD children.
Direct effects: Did the training results persist beyond immediate posttests?
To investigate if the observed gains in WM capacity persisted beyond the immediate posttests, paired-sample t-tests were run comparing the immediate posttest scores to those from the delayed posttests for twelveFootnote 12 of the Magic Memory participants. Before comparing the immediate posttest scores to the delayed posttest scores for these participants, we first wanted to verify that the observed pre to posttest gains still held for this subset of participants. The results confirm that for composite simple span: posttest performance was better than pretest performance even if the difference is only tendential, t(22) = 2.08, p = .05, d = 0.85. For composite complex span, a significant difference between pre and posttest performance was not observed, although there was a slight tendency, despite the small sample size, for the children in this subgroup to have higher scores on the posttests than on the pretests, t(22) = 1.80, p = .08, d = 0.74. Next, we compared immediate posttest scores to those of the delayed posttests, and our results showed that these differences were not significant (p = .91 for simple span and p = .90 for complex span), indicating that the WM gains seem to be relatively maintained over time, which is illustrated in Figure 3.

Figure 3. Distribution of composite WM scores for the pretests, immediate posttests (POST 1), and delayed posttests (POST 2).
Transfer effects: Is there an improvement in syntactic tasks?
Analyses were then performed to examine whether training effects were limited to the domain of WM, or whether there was generalization to less directly related tasks, i.e., syntax, which was the primary aim of our study. For the sentence repetition task, the measures that were of interest were the percentage of correctly repeated syllables, the percentage of respected target structures (subject and object relatives combined), and the percentage of structures in which the degree of embedding was respected (one, two, and three degrees of embedding combined). A repeated measures ANOVA showed significant main effects of cognitive group (TD > DLD) and time (posttest > pretest) for all three of these measures, but no significant main effect of type of training (Table 9).
Table 9. Summary of main effects and interactions for the syntax measures
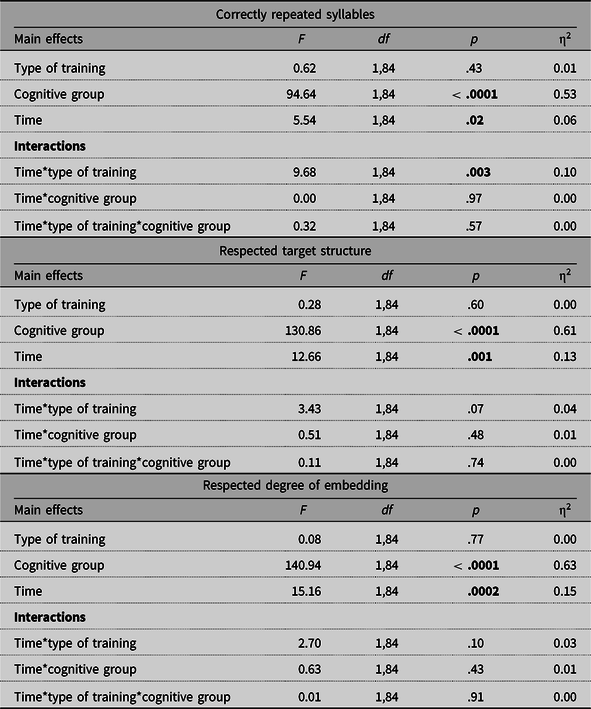
Post hoc Tukey HSD tests, summarized in Table 10, showed that only DLDWM children significantly improved on these syntactic measures: for syllables, for respected structures, and for embedding. There was no significant syntactic improvement for TDWM children or for the TD/DLDSQ children.
Table 10. Summary of pretest vs. posttest comparisons for complex sentence repetition

* p < .05
The results presented in Table 9 also showed a significant time by type of training interaction effect for the percentage of correctly repeated syllables, but as previously stated, post hoc Tukey HSD tests revealed that significant improvement occurred only in the DLDWM group. This interaction effect is likely explained by the significant main effect of time found in the children with DLDWM and by the more modest but consistent improvement of the TDWM children. For the other two variables of interest (percentage of respected structures and embedding), no significant time by type of training interaction effects were observed. There were also no significant time by cognitive group or time by type of training by cognitive group interactions.
Transfer effects: Did the training results persist beyond immediate posttests?
As with the WM tests, we wanted to investigate whether gains observed in the repetition task endured from the immediate posttest phase to the delayed posttest phase. To do this, we first wanted to ensure that the previously observed pre to immediate posttest gains were still present in our reduced sample of twelve participants, but paired-sample t-tests revealed that this was not the case (p = .55 for syllables, p = .49 for structure, and p = .28 for embedding). Consequently, in this subgroup of participants, we were unable to make a meaningful comparison between the immediate posttests and the delayed posttests for the repetition task.
Transfer effects: Are the effects modulated by syntactic complexity factors?
While our main results confirmed that our WM training regime was successful when simple and complex structures (i.e., subject and object relatives) were combined, the goal of our final analyses was to distinguish between more or less complex sentences within our task and to examine if training effects were different with respect to this distinction. To recall, we predicted that these effects would be less obvious for simpler syntactic structures that are hypothesized to be less taxing for WM. To investigate this, all of the items in the repetition task were split into groups by type of sentence (simple sentences containing no relative clause vs. complex sentences containing a relative clause), and complex sentences were split into groups by type of structure (subject vs. object) and degree of embedding (one degree vs. two-three degrees). Before analyzing pre to posttest performance in our participants, we first wanted to verify that complexity effects were in fact present in pretest performance, see Table 11. Paired-sample t-tests revealed that in the pretest phase, children with DLD correctly repeated significantly more syllables in simple sentences without a relative clause (−RC) than in ones containing a relative clause (+RC) which was not the case for TD children who were at ceiling for both measures. Similarly, for what concerns target structure, a complexity effect was only found for children with DLD, who correctly repeated significantly more subject relatives than object relatives in the pretest phase. For TD children, there was no significant difference between these two measures prior to training. However, for degree of embedding, both TD and DLD groups produced significantly more sentences containing one degree of embedding than those containing either two or three degrees in the pretest phase.
Table 11. Summary of comparisons made for pretest measures of differing syntactic complexity when the WM and scholastic training participants are put together in each cognitive group

*** p < .001
Next, we investigated if improvement in the repetition task in the posttest phase was related to degree of complexity of the sentence. We have already reported a significant main effect of time for sentences containing a relative clause in DLD children, but repeated measures ANOVA showed that no significant progress was made for simple sentences without a relative clause (−RC), F(1,84) = 0.58, p = .45, η2 =0.01. In other words, significant improvement only occurred for syntactically complex sentences either containing a subject or an object relative. As for different types of relatives, we found a significant main effect of time for the repetition of subject relatives, F(1,84) = 5.56, p = .02, η2 = 0.06, but with post hoc Tukey HSD analysis it was not possible to statistically distinguish pre and posttest performance in any of the groups, see Table 12. When the same analyses were run for pre and posttest performance of object relatives, a significant main effect of time was also observed F(1,84) = 9.78, p = .002, η2 = 0.10, as well as a significant time by type of training interaction, F(1,84) = 4.96, p = .03, η2 = 0.06. Post hoc Tukey HSD tests (Table 12) revealed a significant pre to posttest difference in only DLDWM children. Finally, for degree of embedding, there was a significant main effect of time for sentences containing a relative clause and one degree of embedding, F(1,84) = 11.76, p = .001, η2 = 0.12, and post hoc Tukey HSD tests confirmed that the pre to posttest improvement was significant only for DLDWM children. For items containing two-three degrees of embedding, no significant effects were observed. For the most part, these results are in line with our predictions, with the exception of degree of embedding. For DLDWM children, significant improvement was observed for more complex sentences containing a relative clause but not for less complex ones without a relative clause, and for more complex object relatives but not for less complex subject relatives.
Table 12. Summary of additional pretest vs. posttest comparisons on measures of differing degrees of syntactic complexity

** p < .01; * p < .05
Discussion
Our study explored the effects on a new intensive WM training, Magic Memory, on WM (assessed via untrained tasks) and mastery of complex syntax (assessed via repetition of relative clauses). This training was administered to 18 TD children aged 6 to 12 years and to 32 children with DLD of the same age. Strong predictive links between WM and syntax have been repeatedly demonstrated, for TD children (Delage & Frauenfelder, Reference Delage and Frauenfelder2019; Marinis & Saddy, Reference Marinis and Saddy2013; Montgomery et al., Reference Montgomery, Magimairaj and O’Malley2008) and also for children with DLD (Durrleman & Delage, Reference Durrleman and Delage2016; Frizelle & Fletcher, Reference Frizelle and Fletcher2015; Zebib et al., Reference Zebib, Tuller, Hamann, Abed Ibrahim and Prévost2019). In the latter case, predictive links between WM and complex syntax (Delage & Frauenfelder, Reference Delage and Frauenfelder2020) may be suggestive of novel therapeutic avenues, in which case we expect improvements in memory abilities to minimize the persistent syntactic deficits associated with DLD. Finally, we compared the effect of WM training to that of age-matched children (18 TD, 20 DLD) who followed an alternative, scholastic training, for whom no syntactic improvement was expected, which would allow us to conclude that the potential positive effects observed in WM trained children stemmed specifically from our WM program. Our original, computerized intervention targeted the aspects of WM shown to predict complex syntax processing in both TD and DLD children, namely serial order memory and dual-task processing (Delage & Frauenfelder, Reference Delage and Frauenfelder2019, Reference Delage and Frauenfelder2020).
Direct effects
Our results confirm our main predictions, specifically that Magic Memory improves WM performance in children with TD and DLD and leads to better repetition of complex sentences in children with DLD. More precisely, a positive direct effect of this program on WM performance was observed for untrained tasks in both TDWM and DLDWM children. Furthermore, a time by training group interaction was found for both simple and complex-span composite scores, which shows that the observed effects are specific to the training program and cannot be attributed to external factors such as maturation or motivation. It is nevertheless true that the WM tasks for which we showed direct effects resemble the activities trained by the WM program, although the visual and verbal material differ, as well as the test modality (paper versus computer-based). However, it is important to note that such direct effects have not always been found in the literature (see Melby-Lervag & Hulme, Reference Melby-Lervag and Hulme2013; Melby-Lervag et al., Reference Melby-Lervag, Redick and Hulme2016), and it is thus noteworthy that children seem to have progressed sufficiently to transfer skills from one support to another. That previous studies did not detect the benefits observed here could be due to the fact that these studies have been mainly conducted with healthy adults and moreover involved generic WM training programs with many visuospatial activities, such as is the case for Jungle Memory and Cogmed. Put differently, our more convincing results may stem from our program’s focus on the specific underlying components of WM found to establish a predictive relationship with syntax in children with difficulties both in WM and syntax. That we do not find an interaction between training type and the cognitive group (TD/DLD) suggests that the two groups respond similarly to training. However, an unexpected result is that TDSQ children also progressed for the simple composite span, which was not observed in DLDSQ children who followed the same alternative training. This progression in TD children could be explained by their normal maturation of simple spans. However, when considering progression in each WM task separately, no significant progression is observed in children who followed the scholastic training, whereas children with DLDWM showed such a progression for 4/5 tasks and TDWM progressed on 2/5 tasks. Finally, we were also able to test for long-term effects of the WM training in evaluating WM performance three months after the end of the training. Even though we could test only 12 of the 32 DLDWM children, these delayed posttests showed that gains in WM seem to be maintained. These results are encouraging regarding the program’s long-term effectiveness, particularly since such effects are generally absent from the literature (Melby-Lervag & Hulme, Reference Melby-Lervag and Hulme2013). However, analyses conducted with a larger cohort of participants that compare their long-term performance with that of control participants are needed to validate these initial observations.
Transfer effects
As for indirect effects on syntactic capacities, we showed a significant effect of time for the measures analyzed in sentence repetition, with posttest performance being better than pretest performance in children with DLDWM, whereas no improvement was observed in children following the alternative training. Amongst these measures, a progression in the percentage of correctly repeated syllables can be explained by the progression of WM abilities, particularly the phonological loop, as these children managed to repeat more digits or nonwords in posttest than pretest. However, DLDWM children also improved their capacity to reproduce the target structure and the expected level of embedding (without considering lexical substitutions or omissions), which is clearly less related to the simple maintenance and recall of the phonological loop, and more to the information processing required for complex WM tasks. Furthermore, that our transfer effects show a progression on sentence repetition cannot be attributed to similarities between the material of Magic Memory and this syntactic test, as may be claimed to be the case for WM tests, since Magic Memory only includes isolated words as verbal material. It should be noted, however, that despite the progress of children with DLDWM, they still perform at a much lower level than TD children at posttests, with scores of 45% for the target structures and 37 % for the degree of embedding, compared to 80% and above for TD children. As for TD children, we were not able to show significant transfer effects in the group who followed the WM training, probably because their performance level was already high in the pretest phase. However, close inspection of Table 8 reveals that this TDWM group still progressed more than the TDSQ children. The fact that numbers of participants vary between TD (18WM, 18SQ) and DLD (32WM, 20SQ) groups can help to explain why more differences are significant in the DLD group than the TD one, although there may be more to the story. Indeed, the finding that transfer effects are only significant in children with DLD and not in TD is consistent with the literature, which has tended to report the absence of such effects in participants without language impairment (Melby-Lervag et al., Reference Melby-Lervag, Redick and Hulme2016). It would seem then that WM training might increase syntactic abilities most when there is a specific deficit in this realm.
Modulation of training effects
We predicted that the more complex sentences were particularly taxing for WM and thus should be the ones that improve the most through WM training. In line with this prediction, we found a significant progression in DLDWM children in repetition of complex sentences, while such a progression is absent for the repetition of simple sentences (without embedding), which were crucially of the same length as the complex ones. Moreover, we found an interaction of time by type of training for object relatives and not for subject relatives. It thus seems that more complex sentences, with a disruption of the canonical word order as it is the case for object relatives, may be more sensitive to WM training than simpler structures such as subject relatives. Here again, this effect is essentially due to DLDWM children, the only group to demonstrate a significant progression for object relatives between pre- and posttest. Note that we consider the disruption of the canonical order attested in object relative clauses to be a factor of complexity, in line with the DCH approach sketched by Jakubowicz (Reference Jakubowicz2005, Reference Jakubowicz2011). However, as mentioned by an anonymous reviewer, the complexity of object relatives can also be linked to the intervention of a similar argument, along the lines of Featural Relativized Minimality (fRM, Rizzi, Reference Rizzi and Belletti2004; Friedman et al., Reference Friedmann, Belletti and Rizzi2009). Future studies should go one step further by examining the effect of our WM training on different types of object structures, namely those with and without syntactic intervention as described by fRM, to see if the training boosts all object structures similarly, or if the nature of syntactic intervention (i.e., featural similarity) modulates this effect.
As for the correct repetition of the target level of embedding, it is interesting that the DLDWM group significantly progressed only for the sentences containing one level of embedding (39% at pretest, 50% at posttest), and not for additional levels of embedding which can be explained by floor effects on these measures (with percentages inferior to 17% for 2–3 levels of embedding). Although not significant, the pattern seems different in the TDWM group: they were at ceiling at pretest for level 1 (89%), which leaves too little room for improvement, yet they showed an improvement of 10% at posttest for sentences with 2–3 levels of embedding for which they were not initially at ceiling (55% at pretest, 65% at posttest). It is clear that our TD groups are too small in number for such differences to be significant, which is a limitation of our study. For future studies, it also seems important to test the effect of WM training on comprehension of such complex structures, since children with DLD also show difficulties in this realm (as in Delage & Frauenfelder, Reference Delage and Frauenfelder2020 or Friedman & Novrogrodsky, Reference Friedmann and Novogrodsky2004). The gains may be subtler in comprehension, as an asymmetry between comprehension and production has been reported in this direction for children with DLD (Jakubowicz & Tuller, Reference Jakubowicz, Tuller and Ayoun2008; Contemori & Garrafa, Reference Contemori and Garrafa2010). Still, if our WM training can improve performance in both modalities, i.e., by giving rise not only to gains in production but also to (possibly subtler) gains in comprehension, this would argue more forcefully for the role of executive functions on the language capacities in DLD.
Overall, our results are consistent with those obtained in previous studies, which highlighted the modulation between the degree of syntactic complexity and WM capacities. In 2019, Delage and Frauenfelder specifically demonstrated that WM evaluated in 48 TD children aged 6–12 years explained a greater part of the variance for relatives with higher levels of embedding (54%) than for relatives with lower level of embedding (38%). In the same vein, the production of subject relatives was not predicted by WM, whereas both simple and complex spans explained a significant part of the variance of results in repetition of object relative clauses. While this prior study was a cross-sectional one, the current study is the first, to our knowledge, to demonstrate this link using a longitudinal, training paradigm. One should ask, however, if a targeted training focusing specifically on complex syntax would produce the same effects. In that sense, it would be interesting to compare the effect of systematic training in WM with that of direct syntactic training (with, for example, the metalinguistic approach of Shape Coding, Ebbels, Reference Ebbels2007). There is still little evidence in the scientific literature regarding the effectiveness of syntax-based speech-language pathology therapy (Ebbels, Reference Ebbels2014; Law et al., Reference Law, Garett and Nye2003), but such trainings, including explicit grammatical interventions, seem to be efficient (Balthazar & Scott, Reference Balthazar and Scott2018; Calder et al., Reference Calder, Claessen, Ebbels and Leitão2020; Zwitserlood et al., Reference Zwitserlood, Wijnen, van Weerdenburg and Verhoeven2015).
Long-term effects
Our findings also suggest potential long-term effects of WM training, given that direct effects seem to be maintained after three months, although this needs to be interpreted with extreme caution given that only 12 participants were retested with delayed posttests. If future studies confirm that children retain the benefits of the training and continue to progress in syntax, the training could be seen as a cognitive “boost” allowing the children to free up resources to process complex syntax. Future work should explore precisely what underlying cognitive processes are enhanced as a result of the training program. Indeed, WM is underpinned by a whole set of attentional and executive processes and more specifically by selective attention (Majerus et al, Reference Majerus, Heiligenstein, Gautherot, Poncelet and Van der Linden2009; Veer et al., Reference Veer, Luyten, Mulder, van Tuijl and Sleegers2017). It is therefore possible that with a training program as intensive as ours, requiring sustained attention for 30 minutes per session, it is the attentional component, included in all of the WM models (Baddeley, Reference Baddeley2003; Barrouillet et al., Reference Barrouillet, Bernardin and Camos2004; Cowan, Reference Cowan, Miyake and Shah1999), that has been particularly called upon. Although selective attention is less studied than WM in its relationship to language development, some studies have already shown a link between attentional abilities and relative sentence comprehension in preschool children (Finney et al., Reference Finney, Montgomery, Gillam and Evans2014). With respect to children with DLD, it is known that a significant proportion of these children suffer from attentional difficulties (Ebert & Kohnert, Reference Ebert and Kohnert2011), suggesting that there is a significant interaction between language and attention disorders. It would therefore be interesting to use pure training of the attentional component as an alternative training, which would make it possible to determine more precisely whether the underlying component necessary for this cognitive boost is indeed the attentional component or, on the contrary, whether it is not sufficient to improve language. Additionally, it seems important to include measures of attention in future work in order to understand how intersubject variability in this domain can affect the progression of trained children. This is also the case for other co-occurring problems frequently found in children with DLD such as speech sound disorders (Pennington & Bishop, Reference Pennington and Bishop2009).
Clinical applications
Finally, this work presents potential clinical implications. Indeed, if future work can replicate our results, and notably can demonstrate robust long-term effects, the logical next step would be to make the training material available to clinicians, which would provide them with innovative and scientifically validated material for the therapy of children with syntactic disorders. The idea here would not be to replace conventional therapy with a computer-based WM protocol, but rather to use it as a complement to this treatment, in the vein of an Evidence-Based Practice approach, consisting of applying the results of research to clinical decision making (Sackett et al., Reference Sackett, Straus, Richardson, Rosenberg and Haynes2000).
Acknowledgments
The authors are grateful to Julien Da Costa for the time he devoted to developing Magic Memory and the various students of the University of Geneva, Paris, and Tours. Finally, we extend our thanks to the team from Squla in Amsterdam, the Netherlands.
Conflict of Interest
There are no real or potential conflicts of interest related to the manuscript.
Financial Support
This study was supported by Swiss National Science Foundation, Grant 100014_159606 (awarded to H. Delage).
Supplementary material
To view supplementary material for this article, please visit https://doi.org/10.1017/S0142716421000369