Skeletal muscle is the largest organ in the body and significantly affects locomotion and the maintenance of posture in both adults and children(Reference Biolo, Cederholm and Muscaritoli1). Skeletal muscle also plays a crucial role in whole-body protein metabolism and systemic glucose and energy homeostasis, thus modulating the risks of certain diseases like obesity, CVD, insulin resistance, diabetes, sarcopenia and osteoporosis(Reference Wolfe2). Therefore, adequate skeletal muscle quantity and quality are essential for maintaining optimal health throughout life. Skeletal muscle mass increases throughout childhood and adolescence before it starts to decrease over time about mid-life(Reference Chen, Li and Chen3). Muscle mass and strength in later life are a reflection of both the rate of muscle loss and the peak muscle mass attained earlier in life(Reference Wilkinson, Piasecki and Atherton4). Therefore, it is necessary to focus on the determinants of peak muscle mass and strength attained in early adulthood.
Skeletal muscle shows high plasticity in response to environmental cues such as exercise and nutrition(Reference Carbone, Mcclung and Pasiakos5–Reference Landi, Marzetti and Martone7). There has been significant interest in the role of protein in muscle health(Reference Gielen, Beckwée and Delaere8). However, evidence concerning several mechanistic pathways, including inflammation and oxidative stress(Reference Cruz-Jentoft and Sayer9), points to the importance of micronutrients. The high level of metabolic activity in skeletal muscle generates reactive oxygen species, and the accumulation of excess reactive oxygen species results in damage to biomolecules in muscle (DNA and proteins). Through their effects on signalling pathways, reactive oxygen species also play a role in inflammation(Reference Damiano, Muscariello and La Rosa10,Reference Foreman, Hesse and Ji11) . Previous studies have shown that inflammatory markers such as plasma levels of IL-6 and tumour necrosis factor-α are negatively correlated with skeletal muscle mass and strength(Reference Fan, Yang and Guo12–Reference Schaap, Pluijm and Deeg14). Reactive oxygen species production is normally balanced by the actions of endogenous antioxidant defense systems such as the enzymes superoxide dismutase and glutathione peroxidase and by exogenous antioxidants, which prevent excess accumulation(Reference Mukund and Subramaniam15). This has focused attention on a range of dietary components with antioxidant effects, namely dietary antioxidants such as vitamin A and vitamin E(Reference Kim, Wilson and Lee16). Vitamin A is a fat-soluble vitamin that involves retinol and retinol derivatives (retinoic acid, retinyl esters and retinaldehyde)(Reference Han, Zhao and Wang17). Vitamin E is also known as tocopherol which includes α, β, γ, δ-l tocopherol. α-Tocopherol is the most active form of vitamin E(Reference Meulmeester, Luo and Martens18). Cross-sectional studies have shown that good antioxidant status is inversely associated with relevant lipid and oxidative stress markers(Reference Cocate, Natali and Alfenas19), muscle strength(Reference Welch, Jennings and Kelaiditi20,Reference Semba, Blaum and Guralnik21) and the risk of frailty(Reference Michelon, Blaum and Semba22,Reference Kochlik, Stuetz and Pérès23) . Prospective cohort studies have shown that people with high serum retinol or α-tocopherol concentrations at baseline exhibit a lower rate of muscle decline and risk of frailty(Reference Alipanah, Varadhan and Sun24–Reference Sahni, Dufour and Fielding28). However, the participants included in the above studies were middle-aged and elderly people. Few studies have focused on the associations of retinol and α-tocopherol levels with skeletal muscle health in children.
With the above in mind, we explored the associations of plasma retinol and α-tocopherol with skeletal muscle mass and strength in Chinese children aged 6–9 years.
Methods
Participants
This cross-sectional study was performed in Guangdong province in the south of China in 2015–2017(Reference Liang, Chen and Fang29). The investigators recruited healthy children aged 6–9 years from several kindergartens and primary schools. The recruitment strategy involved giving out leaflets to primary schools and contacting the mutual acquaintances of parents through public WeChat accounts. A total of 1600 children were invited to participate in the study, 521 of whom responded and agreed to participate in the study. We excluded ninety-five children based on the following criteria: The following exclusion criteria were applied: (1) twins and preterm births; (2) incomplete general data or skeletal muscle testing or hand grip strength testing data and (3) history of serious disease or disability. Finally, 426 participants (243 boys and 183 girls) were included. We obtained written informed consent from the parents of all enrolled children.
The study was conducted in accordance with the Declaration of Helsinki and approved by the Ethics Committee of the School of Public Health at Sun Yat-sen University (No. 201549).
Anthropometric measurements
Weight and height were measured with the participant’s barefoot and wearing light clothing. BMI was then calculated. Whole-body dual X-ray absorptiometry scans were using a Hologic Discovery W System (Discovery W; Hologic Inc.) to determine the appendicular skeletal muscle mass (ASM). Thirty-three randomly selected subjects were replicating the measurements on the same day after repositioning to evaluate the reproducibility and the in vivo reproducibility of the ASM was 1·56 %. ASM index (ASMI) was calculated as follows:
BMI = weight (kg))/height (m)2,
ASM index (ASMI) = ASM (kg)/height (m)2,
ASMI Z-scores were calculated with respect to the ASMI value provided by Liu et al. (Reference Liu, Yan and Xi30). As such, children were classified as having high muscle mass (Z-score ≥ 1, n 234), medium muscle mass (Z-score 0–1, n 139) or low muscle mass (Z-score < 0, n 53).
Hand grip strength measurement
HGS was measured using a Jamar® Plus+ Hand Dynamometer (JAMAR® Hydraulic Hand Dynamometer, Sammons Preston) as previously described(Reference Chen, Li and Chen3). All participants sat in a straight-backed chair with their feet flat on the floor. The participants were placed in a standardised position with their shoulders adducted and neutrally rotated, elbows flexed at 90°, forearms in neutral rotation and wrists between 0° and 30° of extension and between 0° and 15° of ulnar deviation. The handle of the device was set to the second knuckle. The children were instructed to squeeze the handle of the dynamometer as hard as possible during a 5-s period with both hands while exhaling. HGS was recorded in kilograms (kg). The mean of the three trials was calculated and used in subsequent analysis.
Laboratory examination
Venous blood samples were obtained from the children in the morning after 10 h of fasting. More than 90 % of blood samples are collected by investigators on the scene. The plasma was separated from blood within 2–4 h and setted up parallel samples to test the stability of the monitoring. Samples were stored at −80°C unexposed to light until assayed in 2022. Quantitative analysis of plasma retinol and α-tocopherol concentrations was performed using a Qlife Lab 9000 triple quadrupole mass spectrometer testing system under research protocols including quality assurance measures(Reference Lin, Zhou and Li31). A pooled serum sample was stored in separate EP tubes to prevent repeated freeze–thaw and analysed alongside batches of study samples to monitor analytic precision, with approximately coefficient of variation values of 7·7 %.
Covariates
The interviewers collected data concerning mode of delivery, household income and parental educational level through face-to-face interviews. Parental education level was classified into three categories: primary or lower, secondary and graduate or above. Household income per month was classified into four categories: ≤ 8000 yuan, 8000–15 000 yuan, > 15 000 yuan and no response. The delivery mode was defined as a binary variable: cesarean or vaginal. Dietary intake over the prior year was assessed using a seventy-nine-item FFQ. During the interviews, the investigators checked the questionnaires for potentially incorrect responses and made clarifications when necessary. The reproducibility and validity of the FFQ were described previously(Reference Chen, Li and Liang32). Energy and nutrient intakes were calculated based on the Chinese food composition tables (2018)(Reference Yang, Wang and Pang33). Each nutrient intake value was adjusted using the energy-residual method. Information on physical activity was obtained using a 3-day physical activity questionnaire. Physical activity was calculated by combining the metabolic equivalent score for each type of physical activity after multiplying it by its duration (hours) per day(Reference Ainsworth, Haskell and Herrmann34).
Statistical analysis
Normally distributed continuous variables are expressed as means ± standard deviations, while medians and interquartile ranges are used to describe non-normal distributions. Categorical variables are presented as percentages. Descriptive statistics of sex differences in subject characteristics were tested for significance using analysis of variance for continuous data, while the χ 2 test was used for categorical data. Multiple linear regression and ANCOVA were applied to examine the association of plasma retinol and α-tocopherol concentrations with muscle mass indicators. We constructed three models with minimum and maximum adjustments. In model 1, adjustments were made for age; in model 2, we added delivery mode, parental education, household income, physical activity, use of Ca and multivitamin supplements and energy-adjusted dietary intakes of total energy and protein. Whole-body fat was further adjusted for in model 3. All analyses were conducted using R version 4.1.2. The significance level was set at 0·05.
The sample size was estimated based on a similar study that the relationship between protein and skeletal muscle. We assumed that retinol and α-tocopherol have a similar or slightly lower magnitude of association with skeletal muscle than protein(Reference Asp, Richardson and Collene35). Setting a two-tailed α of 0·05 and a power of 0·80, the current study initially required a sample size of 173 to detect the association between skeletal muscle status and the level of plasma retinol and α-tocopherol as low as R 2 = 0·05 adjusting for eleven covariates in multiple linear regression models. Power analysis was performed using PASS software (version 15; Jerry Hintze, Kayville, UT).
Results
Participant characteristics
A total of 243 boys and 183 girls were included in this study. Table 1 shows the characteristics of caregivers and the differences between boys and girls. The mean age of the study population was 8·04 years (Q1–Q3:7·30–8·80). The boys tended to be older and had higher body weight, BMI, physical activity level, daily energy and protein intake, ASM, ASMI, left HGS and right HGS than the girls (P < 0·001–0·016). However, there was no significant difference in ASMI Z-score between the boys and the girls (P = 0·210). The boys had similar plasma retinol concentrations (312 ng/ml v. 326, ng/ml, P = 0·087) but lower plasma α-tocopherol concentrations than the girls (5·42 μg/ml v. 5·75 μg/ml, P = 0·023).
Table 1. Baseline characteristics of participants

Continuous variables are presented as mean (sd) or median (Q1–Q3); ASM, appendicular skeletal muscle mass; ASMI, the ratio of appendicular skeletal mass to height2; Left HGSm left handgrip strength, Right HGSm right handgrip strength; ASMI-Zm the Z score of ASMI.
Relationship between plasma retinol and muscle mass
As shown in Table 2, after adjustment for potential confounders, multiple linear regression revealed that a per-unit increase in the plasma concentration of retinol led to a 2·43 × 10−3 kg, 1·33 × 10−3 kg/m2, 3·72 × 10−3 kg and 2·45 × 10−3 increase in ASM, ASMI, left HGS and ASMI Z-score in the girls (P < 0·001–0·050). In the boys, no significant association was detected between any of the muscle mass indicators and the plasma retinol concentration.
Table 2. Multiplelinear regression between plasma retinol and α-tocopherol concentration and skeletal muscle indexes
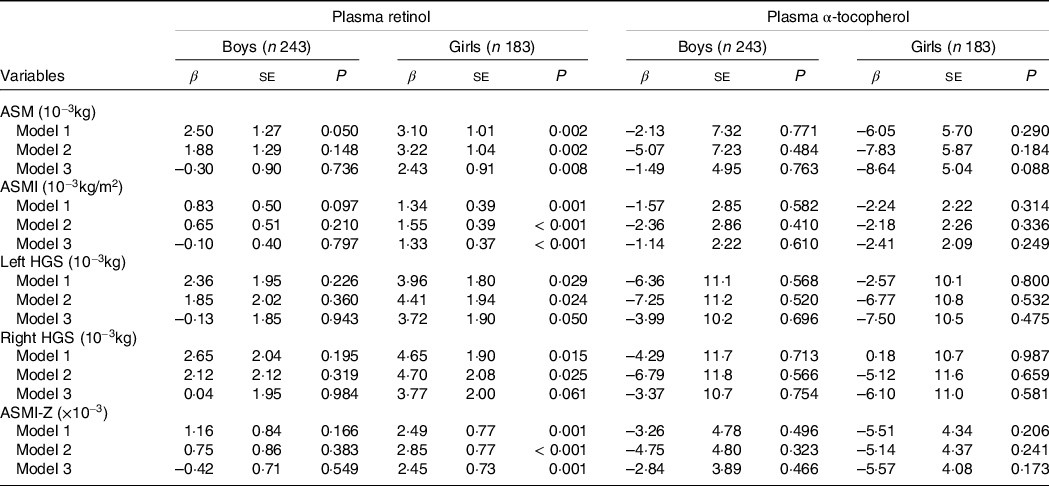
ASM, appendicular skeletal mass; ASMI, the ratio of appendicular skeletal mass to height2; AFM, appendicular fat mass; Left HGS, left handgrip strength, Right HGS, right handgrip strength; ASMI-Z, the Z score of ASMI.
Model 1: adjustment for age, model 2: adjustment for age, delivery mode, parental education, physical activity, use of Ca and multivitamin supplements, energy adjusted dietary intakes of total energy and protein and household income; model 3: adjustment for age, delivery mode, parental education, physical activity, use of Ca and multivitamin supplements, energy adjusted dietary intakes of total energy and protein, household income and whole-body fat.
Figure 1 shows the results of ANCOVA. For the boys, none of the muscle mass indicators were associated with the plasma retinol concentration. In the girls, most of the muscle mass indices increased significantly with the tertile of the plasma retinol concentration, and the adjusted percentage mean differences for tertile 3 v. tertile 1 were 8·38 %, 6·26 %, 12·1 %, 13·2 %, and 116 % for ASM, ASMI, left HGS, right HGS and ASMI Z-score, respectively (P trend: 0·001–0·007, P- diff: 0·005–0·020).
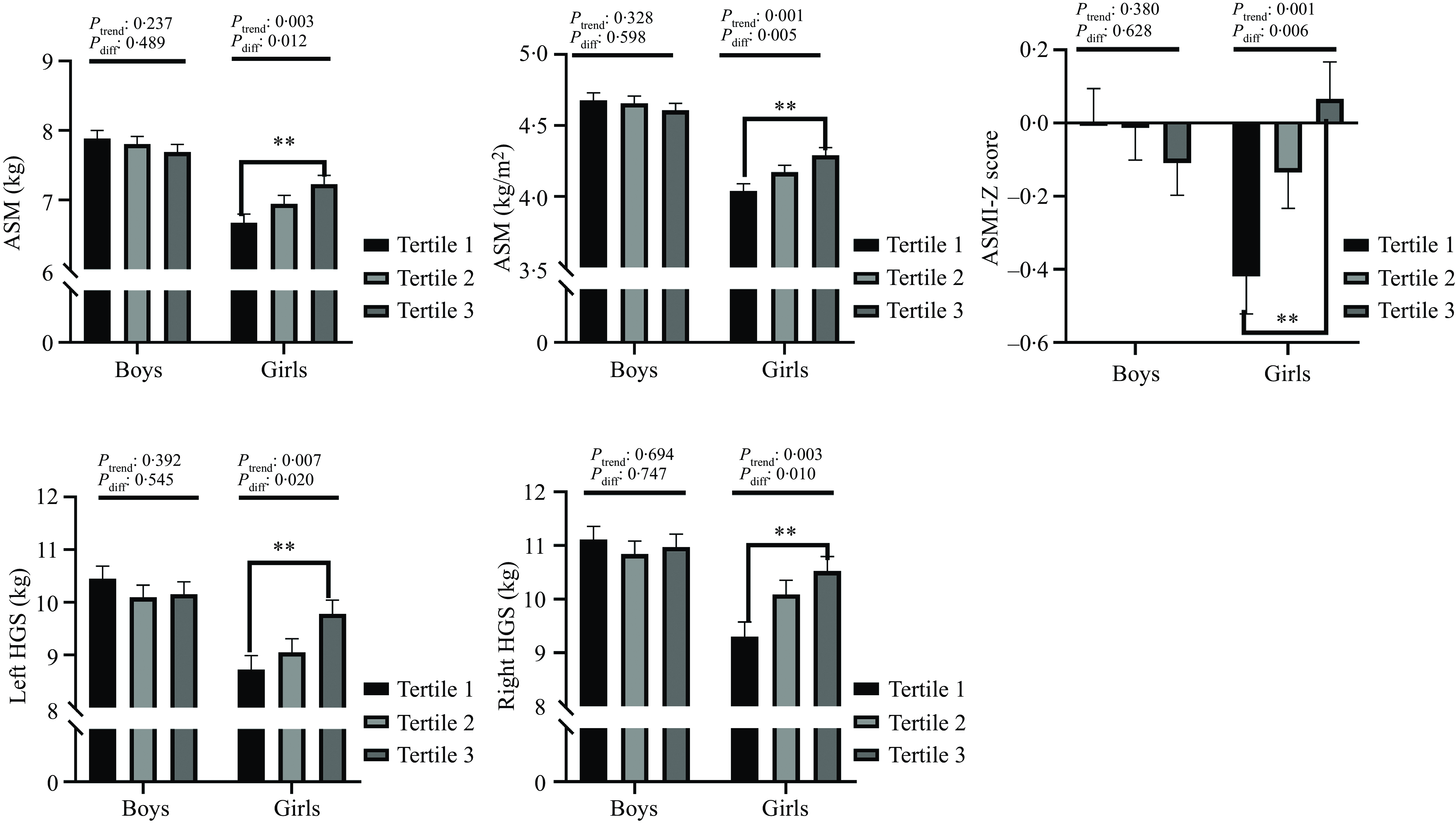
Fig. 1. Analysis of covariances for the association between plasma retinol concentration tertiles and muscle mass and muscle strength. Results were adjusted for age, delivery method, household income, parental education, physical activity, use of Ca and multivitamin supplements, energy adjusted dietary intakes of total energy and protein and whole-body fat. ASM, appendicular skeletal mass; ASMI, the ratio of appendicular skeletal mass to height2; Left HGS, left handgrip strength, Right HGS, right handgrip strength; ASMI-Z score, the Z score of ASMI.
Relationship between plasma α-tocopherol and muscle mass
As shown in Table 2, no statistically significant associations between the plasma concentrations of α-tocopherol and muscle mass indicators were detected either in the boys or the girls using the three multiple linear regression models. Similar results were obtained using ANCOVA (Fig. 2).

Fig. 2. Analysis of covariances for the association between plasma α-tocopherol concentration tertiles and muscle mass and muscle strength. Results were adjusted for age, delivery method, household income, parental education, physical activity, use of Ca and multivitamin supplements, energy adjusted dietary intakes of total energy and protein and whole-body fat. ASM, appendicular skeletal mass; ASMI, the ratio of appendicular skeletal mass to height2; Left HGS, left handgrip strength, Right HGS, right handgrip strength; ASMI-Z score, the Z score of ASMI.
Discussion
In this observational study, we demonstrated that girls with higher circulating levels of retinol had higher skeletal muscle mass and strength than girls with lower retinol levels, whereas no such relationship was present in boys. Furthermore, we detected no significant association between the circulating levels of α-tocopherol and skeletal muscle status in either sex.
Many studies in adults have evaluated the impacts of dietary antioxidants on skeletal muscle health. In the Invecchiare in Chianti study, which included 986 men and women aged 63–73 years, higher antioxidant concentrations (e.g. vitamin C, vitamin E, beta-carotene and retinol) were associated with greater knee extension strength and better physical performance metrics such as walking speed, ability to stand from a chair and ability to maintain balance in progressively more challenging positions(Reference Cesari, Pahor and Bartali36). Another study in elderly people aged 60–85 years confirmed that the combined supplementation of whey protein, vitamin D and vitamin E can significantly improve relative skeletal muscle mass index (mean difference: 0·18 kg/m2, 95 % CI: 0·01, 0·35, P = 0·040) and muscle strength (mean difference: 2·68 kg, 95 % CI: 0·71, 4·65, P = 0·009) in older adults when compared against a placebo group(Reference Bo, Liu and Ji37). Pilleron et al. reported that a greater prevalence of frailty was associated with lower concentrations of either carotenoids, retinol, or α-tocopherol(Reference Pilleron, Weber and Pérès38). However, the findings obtained in the elderly have not always been confirmed in other populations. In young athletes, Teixeira et al. reported that antioxidant supplementation (e.g. α-tocopherol, vitamin C, lutein, Se, Zn and Mg) did not offer protection against exercise-induced lipid peroxidation and inflammation and might hinder recovery from muscle damage(Reference Teixeira, Valente and Casal39). Importantly, some studies have highlighted that prolonged antioxidant supplementation can lead to undesirable effects, such as the disruption of endogenous antioxidant levels leading to failure to counteract exercise-induced oxidative stress(Reference Rowlands, Pearce and Aboud40,Reference Peternelj and Coombes41) . To date, most studies have focused on adults and the elderly, and comparatively little research has investigated how antioxidant vitamin status correlates with skeletal muscle quality in children. In our study, we measured the concentrations of retinol and α-tocopherol in plasma and found that concentrations were significantly different between the sexes. This result was in line with other studies in the literature(Reference de Dios, Navarro and Ortega-Senovilla42–Reference Borel, Moussa and Reboul46). In addition, the findings of the present study revealed that plasma retinol levels were positively correlated with skeletal muscle health in girls but not in boys. Furthermore, the plasma concentration of α-tocopherol was not correlated with skeletal muscle mass and strength in either sex.
Increasing evidence has shown that aging-mediated oxidative stress and inflammation are the main pathological characteristics of skeletal muscle(Reference Chen, Wang and Deng47). Therefore, antioxidants derived from food may have beneficial effects on skeletal muscle health. The antioxidant and anti-inflammatory properties of vitamin A and vitamin E have been demonstrated in a multitude of studies in cells, animal models and humans(Reference Liao, Omage and Bormel48,Reference Palace, Khaper and Qin49) . However, increasing evidence suggests that the predominant effects of vitamin A within the body stem not directly from its antioxidant action but its metabolite all-trans retinoic acid, a potent transcriptional regulator that affects the expression levels of hundreds of distinct genes involved in responses to oxidative stress. In contrast, vitamin E supposedly acts as a direct antioxidant. Very little compelling evidence demonstrates that vitamin E has a direct effect on gene expression as vitamin A does(Reference Blaner, Shmarakov and Traber50). Vitamin A also plays a vital role in helping cells and tissues to grow and develop(Reference Carazo, Macakova and Matousova51). These differences may collectively contribute to the different actions of vitamin A and vitamin E detected in the present study.
The mechanisms that mediate the sex-specific differences detected in the present study remain unclear. However, the considerable number of animal studies performed to date suggest a metabolic basis for the difference in response to changes in vitamin A status between the sexes. During experimental vitamin A depletion, male animals exhaust their liver stores more rapidly than females(Reference Brenner, Brookes and Roberts52), which may predispose males to earlier developed severe manifestations of hypovitaminosis A(Reference Klein-Szanto, Clark and Martin53,Reference McLaren54) . In breast cancer cells, estrogen receptor status was proven to sensitise the antiproliferative effects of retinoids(Reference Rousseau, Nichol and Pettersson55). In males, on the other hand, androgens retard RA function by repressing RAR-α and RAR-γ (Reference Huang, Li and Von Hagen56). Previous researchers found that sex hormone concentrations are significantly higher in girls compared with boys in prepuberty, and the sex differences in terms of those hormones were more robust for 17β-estradiol(Reference Courant, Aksglaede and Antignac57). These studies suggest that a sex difference may exist in vitamin A storage, utilisation, or function mediated by sex hormones.
This research explored the association of retinol and α-tocopherol with skeletal muscle mass and strength in a relatively large sample of children, and all of the measurements were under strict quality control. However, there are some notable limitations. First, this study involved a cross-sectional design. As such, the longitudinal changes in the plasma concentrations of retinol and α-tocopherol were not evaluated. Conventional observational studies cannot completely rule out reverse causality and residual confounding, which makes it hard to infer causality. Future studies should collect data from the study population over time to evaluate longitudinal changes. Second, the plasma sample was assayed for retinol and α-tocopherol levels six years after collection. But at −70°C or colder, retinol and α-tocopherol are stable for 15 years(Reference Comstock, Alberg and Helzlsouer58). Third, this study focused on urban children from a narrow age range, which limits the generalisability of the findings. Finally, although the findings were adjusted for a range of dietary and lifestyle confounders, residual or unmeasured confounding factors inevitably remain unaccounted for.
In conclusion, the present study revealed that plasma retinol concentration is positively correlated with skeletal muscle mass and strength in girls. An improved understanding of the effects of increasing dietary intake of vitamin A may inform future strategies to improve muscle quality.
Acknowledgements
We thank the research team members from the Department of Nutrition and Food Hygiene and Food Safety and Health Research Center of Southern Medical University for their help in sample collection. We also thank the families who participated in this research. This work was supported by National Natural Science Foundation of China (No. 81973045) and Guangzhou Science and Technology Planning Project (No. 202102020967).
This work received support from the National Natural Science Foundation of China (No. 81973045) and Guangzhou Science and Technology Planning Project (No. 202102020967). The funders had no role in the study design, data collection, data analysis, writing of this article or in interpreting the results.
Conceptualization: Z. Z. and H. D.; Investigation: X. Z., J. H., F. C., Z. H., X. L. and J. L.; Writing – Original Draft: J. H. and X. Z.; Writing – Review & Editing: Z. Z. and D. H. . All authors have read and agreed to the published version of the manuscript.
The authors declare no conflict of interest.