The essential role of iodine in thyroid hormone production and in the regulation of metabolism, growth and development has been extensively documented( Reference Zimmermann 1 – Reference Gunnarsdottir and Dahl 3 ). In most industrialised countries, the main dietary sources of iodine are iodised salt (approximately 50 %) and animal food products; of which, dairy products are the largest contributors, accounting for up to 40 % of total iodine intake as has been shown and discussed by different authors( Reference Johner, Gunther and Remer 4 – Reference Haldimann, Alt and Blanc 8 ). Despite the global WHOUniversal Salt Iodisation Programme( 9 ), and regional strategies for iodine deficiency prevention and control, which in Europe included initiatives of legislation in the use of iodised salt in industrial food production( Reference Remer and Neubert 10 ), and more recently in bread( Reference Rasmussen, Ovesen and Christensen 11 , Reference Vandevijvere, Mourri and Amsalkhir 12 ), to mention some; iodine deficiencies in the European region still persist( Reference Zimmermann and Andersson 13 – Reference Zimmermann 15 ).
Evidence from studies on the effect of diets containing little to no animal food products on iodine status is limited, but overall, the literature on this topic suggests that lower intakes of animal food can contribute to inadequate iodine intakes( Reference Krajcovicová-Kudlácková, Bucková and Klimes 16 – Reference Lightowler and Davies 18 ). Although there is still controversy about the impact of reducing dietary protein intake in children and potential health outcomes( Reference Hörnell, Lagström and Lande 19 ), existing dietary guidelines for healthy eating, for example, the ‘New American Plate’ from the World Cancer Research Fund/American Institute for Cancer Research, advise the limitation in the intake of animal protein and to increase the intake of plant-based foods to prevent chronic diseases( 20 , 21 ). In addition, reducing Na intake in children is also part of the global health initiatives in the prevention of chronic diseases( Reference Campbell, Dary and Cappuccio 22 – 25 ). The existing typical Western diets, in contrast, are characterised by high intakes of processed foods, animal food products and salt( Reference Cordain, Eaton and Sebastian 26 ). As studies suggest an association between processed foods as well as meat and increased salt intake( Reference Alexy, Cheng and Libuda 27 – Reference Thomson 29 ), the question arises whether, diets with lower animal foods (e.g. meats) and thereby possibly also decreased iodised salt intake may contribute to depleted iodine status as reflected in lower iodine excretion. To our knowledge, to date, no studies have assessed the association of animal- and plant-based food sources with urinary iodine status in children consuming typical Western diets. To specifically examine the long-term effect of diets predominantly animal or plant based on iodine status, we studied the 24-h urinary iodine excretion (24-h UI) in a sample of German schoolchildren providing multiple collections of both 24-h urine samples (with available Na excretion measurements) and parallel 3 d weighed food records, during the years 1993–2010. In Germany, a particularly improved iodine status in the population has been observed since 1993 parallel to legislation amendment, facilitating the use of iodised salt (NaCl) in all processed foods( Reference Remer and Neubert 10 , Reference Remer, Fonteyn and Alexy 30 ). However, the iodisation of salt used in households or for food production is still on a voluntary basis. During the last years (starting in 2004), the use of iodised salt by the food industry began to decrease in Germany, and by now encompasses only < 30 % of total added salt, probably contributing to a negative trend in iodine status( Reference Johner, Gunther and Remer 4 , Reference Johner, Thamm and Nöthlings 5 ).
We used the ‘animal:plant protein ratio’ of the children's habitual diet as an approach to characterise predominantly animal- or plant-based diets. We hypothesised that those children with healthier food patterns (low animal:plant protein ratio) have lower iodine excretion than children with high animal:plant protein ratio; the individual change in dietary sources of protein (animal-related to plant-based) and 24-h UI was evaluated using repeatedly collected dietary records and 24-h urines. As a second aspect, we aimed to investigate whether the potential association between animal:plant protein ratio and urinary iodine excretion may be mediated by an increase in salt consumption (namely iodised salt) with high animal protein diets.
Subjects and methods
Design of the study
The Dortmund Nutritional and Anthropometric Longitudinally Designed (DONALD) Study is an ongoing, observational open cohort study conducted at the DONALD Study Centre of the University of Bonn (formerly Research Institute of Child Nutrition) in Dortmund, Germany. Details on the study protocol have been published elsewhere( Reference Buyken, Alexy and Kersting 31 ). Briefly, since 1985, detailed data on growth, diet and metabolism have been collected from healthy subjects between infancy and adulthood. In general, healthy infants are newly recruited each year and are first examined at the age of 3–6 months with continuing period follow-up examinations until reaching adulthood. The regular, non-invasive assessments (until the age of 18 years), that take place at intervals of about 1 year, include 3 d weighed food records, anthropometric measurements, collections of 24-h urine samples and interviews on lifestyle and medical assessments. The study was approved by the Ethics Committee of the Rheinische Friedrich-Wilhelms-University of Bonn (Germany). All examinations are performed with parental written consent and children's assent.
Study sample
For the purpose of this analysis, we used data from children aged 6–12 years participating in the DONALD Study from January 1993 to December 2010 (n 675 and n 2781 measurements). To be eligible for the present analysis, children had to be within the age range, and providing at least two complete 24-h urine samples, each with concurrent 3 d weighed food record and anthropometrical data during the observational period. The individual number of 24-h urine samples and corresponding dietary records ranged from two to a maximum of seven possible measurements during the 17-year time period (for a 6–12 years age range). We excluded those observations that met one or more of the following criteria: (1) incompletely collected urine sample, i.e. when body weight-related 24-h creatinine excretion rate was below the cut-off value of 0·1 mm/kg body weight per d( Reference Remer, Neubert and Maser-Gluth 32 ); (2) implausible dietary records, i.e. when the total recorded energy intake was inadequate in relation to the BMR( Reference Schofield 33 ); (3) estimated total protein intake < 0·66 g/kg of body weight, corresponding to the minimum maintenance requirement of protein( Reference Garlick 34 ); or (4) use or intake of medication or supplements containing iodine. Hence, the final study sample for the present analysis consisted of 516 children (n 159 excluded), 51·4 % male and 48·6 % female, providing 1959 collected 24 h urines and related dietary and anthropometrical records (mean 3·8 measurements per subject over the total study period).
Urinary assessment
Annual 24-h urine collections for children older than 3 years were routinely performed in the DONALD Study usually on the third day of the 3 d weighed food record under standardised conditions. All urine samples collected during the 24-h collection period were immediately stored frozen at − 20°C in Extran (Extran, MA03; Merck) cleaned, preservative-free one litre plastic containers before being transported to the DONALD Study Centre (formerly, Research Institute of Child Nutrition) where they were stored at − 22°C until analysis( Reference Remer, Montenegro-Bethancourt and Shi 35 ). Iodine concentration was determined in the 24-h urine samples by a modified Sandell–Kolthoff method after acidic wet-ashing of the samples( Reference Lorenz-Wawschinek, Tiran and Eber 36 ). Twenty-four hour Na excretion was measured by flame atomic absorption spectrometry with a Perkin Elmer 1100 Spectrometer (Perkin Elmer).
Nutritional assessment
Food consumption in the DONALD Study was assessed using 3 d weighed food records( Reference Buyken, Alexy and Kersting 31 ). Energy and nutrient intakes were calculated from the nutrient values of the food ingredients using recipes available in the in-house database LEBTAB, which incorporates information from standard nutrient tables, product labels or recipe simulation based on the labelled ingredients and nutrients( Reference Sichert-Hellert, Kersting and Chahda 37 ). For the present analysis, the data were derived for each participant from the mean of the three dietary recording days. Composite dishes were disaggregated into their corresponding components, e.g. ‘pizza’ into wheat, cheese and water, and assigned to a food group category (e.g. cereals, legumes, fruits, vegetables, milk, cheese, meat and fish). The calculated protein intake was divided into (1) dairy protein including protein content from milk and whey-based milk products (yoghurt, buttermilk, cream, condensed milk and quark) and all varieties of cheese; (2) animal protein including protein content from eggs; all type of meats and chicken; fish and seafood and (3) plant protein including protein content from wheat and all wheat products, cereals (breakfast cereals, oats, sprouts); rice, potatoes, fruits, vegetables, mushrooms, soya products (only solid fraction of soya milk), legumes, nuts and pulses. Total protein was estimated as the sum of protein from all sources (dairy+animal protein+plant protein). Based on this classification, dietary animal:plant protein ratio were calculated. A priori, we decided to exclude dairy protein from the ratios for the following reasons: dairy products are often important constituents of the vegetarian food pattern, not just in Germany but also in other industrialised countries such as the USA, as literature suggests( Reference Haddad and Tanzman 38 , 39 ). Milk has a relatively high iodine content( Reference Bath, Button and Rayman 40 – Reference Köhler, Fechner and Leiterer 43 ), and recent studies in German children suggest milk to be an important iodine source in the children's diet( Reference Johner, Gunther and Remer 4 , Reference Johner, Thamm and Nöthlings 5 ). Due to these reasons, the inclusion of dairy in the animal:plant protein ratio may have introduced a correlation bias, which led to an overestimation of the association between the animal:plant protein ratio and 24-h UI. Milk and milk products whatsoever were included as separate covariate in the PROC MIXED model (see statistical analyses).
Regular use of iodised salt was reported in dietary records or parental interviews by nearly 100 % of the participating children. Na (mg) and salt intake (g) were estimated from the urinary Na excretion values (1 g NaCl = 400 mg Na).
Anthropometry and parental data
Anthropometric measurements of the DONALD participants were performed at the time of dietary recording and urine collection by trained personnel following standard procedures. Body weight was measured using an electronic scale (Seca 753 E; Seca Weighing and Measuring System) to the nearest 0·1 kg. Height was measured in a standing position to the nearest 0·1 cm using a digital telescopic stadiometer (Harpenden), the children were dressed in underwear only and barefoot. BMI was calculated as body weight (kg) divided by height (m2). Sex- and age-independent BMI standard deviation scores were calculated using German National Reference data( Reference Neuhauser, Schienkiewitz and Schaffrath Rosario 44 ). Body Surface Area was estimated using the formula of Du Bois & Du Bois( Reference Du Bois and Du Bois 45 ).
At their child's admission to the study, parents provided information on educational background and they were also weighed and measured by the same personnel and equipment as the participating children. The weight was taken with light clothes and without shoes. Maternal education and maternal overweight (BMI ≥ 25 kg/m2) were tested additionally as parameters that can potentially influence the dietary preferences in children( Reference Lin, Bolca and Vandevijvere 46 ).
Statistical analyses
All statistical analyses were carried out by using SAS® (version 9.1.3; SAS, Inc.). In all statistical tests, a P-value < 0·05 was considered as significant. Descriptive data for anthropometric, dietary and urinary parameters are presented as medians and interquartile ranges stratified by (1) sex; and (2) quartiles of animal:plant protein ratio. Quartiles of animal:plant protein ratio (excluding dairy protein) were grouped as low (lowest quartile), medium (middle two quartiles) and high (highest quartile). All observations (i.e. the repeated measurements at different time points from the same individual) were included for the calculations of medians. Sex differences and differences between animal:plant protein ratio quartiles were tested by repeated measures regression models (PROC MIXED, also linear mixed regression model in SAS) to account for the lack of independence that exists between repeated observations on the same person (comparable to a cross-sectional analysis).
PROC MIXED, including both fixed and random effects, was also used for testing the longitudinal association of animal:plant protein ratio with 24-h UI (μg/d). An advantage of the MIXED procedure is that it does not exclude children from the analysis if they have missing data for a particular time point, but it analyses all variables on the assumption that any missing data are missing at random. The random component of the PROC MIXED model accounts among other for the lack of independence that exists between repeated observations on the same person.
The basic longitudinally regression model included animal:plant protein ratio, age, time and time × time as fixed effects. The variable ‘time’ (continuous, 1993–2010) was included as time, and time × time in the model because of the suggested variation of the 24-h UI through the time period( Reference Johner, Gunther and Remer 4 , Reference Remer, Fonteyn and Alexy 30 ). Potentially confounding factors influencing 24-h UI were considered as covariates. Only variables that substantially modified the coefficient of animal:plant protein ratio by >10 % in the basic models, or significantly predicted the outcome variable (24-h UI), or improved the fit statistic (Akaike's information criterion) were included in the subsequent multivariate analyses. All final multivariable models (model 1) included animal:plant protein ratio, age (years), time (chronological time of study years 1993–2010 and time × time), energy intake (MJ/d), 24-h urine volume (ml/d) and milk and milk products (g/d) as independent fixed effects. Diets with high animal protein intake may also be characterised by higher salt intake( Reference Alexy, Cheng and Libuda 27 ), which is one of the most important iodine sources. Because salt intake might mediate the association between animal:plant protein ratio and iodine excretion, 24-h urinary Na excretion (as marker for salt intake) was tested in final multivariable models (model 2). To allow for the distinction between within-person and between-person effects of animal:plant protein ratio on 24-h UI, the data were first centred on the respective person-specific means of the animal:plant protein ratio over time (i.e. individual's mean), and these means were used to predict the between-person effects on 24-h UI (i.e. the associations of between-person differences in protein consumption with differences in mean 24-h UI between the subjects). After that, time-specific deviations from the person-specific means were calculated (i.e. individual value − the individual's mean) and used to test whether within-person changes on protein intakes were associated with within-person changes in 24-h UI( Reference Curran and Bauer 47 , Reference Hoffman and Stawski 48 ).
To estimate the prevalence of children at risk of inadequate iodine intake, 24-h UI excretion levels (μg/d) were compared to the estimated average requirements (EAR, i.e. 55 μg/d for 4–8 years old; 62 μg/d for 9–13 years old)( 49 ), after considering a mean non-urinary iodine loss of 15 %( Reference Johner, Gunther and Remer 4 ).
Results
A general description of the study sample with respect to anthropometry, dietary intakes and 24-h urine parameters is shown in Table 1. In total, median (25th–75th interquartile range) of urinary iodine excretion (24-h UI) among these children was 75 (56–98) μg/d. Both iodine excretion (μg/d) and iodine concentration (μg/l) were significantly higher in boys than in girls, but no significance remained when corrected by individual energy intake. Regarding dietary intakes, median estimated protein intake was 1·7 (25th–75th interquartile range 1·4–2·0) g/kg for all children. Animal protein, including dairy, contributed 65·7 % to the total protein intake, ranging from 17·3 to 88·8 %.
Table 1 Anthropometric, nutritional and urinary characteristics of 6- to -12-year-old participants of the Dortmund Nutritional and Anthropometric Longitudinally Designed Study (1959 measurements) (Median values, 25th–75th percentiles)

BMI-SDS, sex- and age-independent BMI standard deviation scores.
* Sex differences were tested with a linear mixed-effects regression model (PROC MIXED). PROC MIXED was used to account for the dependence of multiple measurements within the same child.
† Estimated from urinary Na (1 g of NaCl = 0·4 g Na).
‡ Animal protein includes protein from fish, poultry and all meat products.
§ Dairy protein includes protein from milk and all milk products.
Cross-sectional comparison of anthropometrical, urinary and dietary variables by quartiles of animal:plant protein ratio is shown in Table 2. Both urinary iodine excretion (μg/d) and iodine concentration (μg/l) were lower in the low animal protein diet than in the protein-rich diet (high ratio) in boys and girls; however, they were not significant. Overall, iodine excretion values below the EAR were observed in 14·3 % (n 280) of the measurements. The proportion of observations below the EAR was higher in the lower animal protein eaters (12·8 % in boys and 19·2 % in girls) than in the higher animal protein consumers (10·3 % in boys and 14·5 % in girls) but statistically was not significant. Children in the high ratio category consumed more protein than children in the lower ratio category (differing by approximately 6 g/d). In the expected direction, Na excretion was higher when more animal protein was consumed (high ratio) but differed significantly only in boys. Proportion of mothers with lower education level was significantly higher in the high animal:plant protein ratio in boys and girls (P< 0·0001). Proportion of maternal overweight was higher in the high-ratio group, but was significant only in girls (P= 0·0002) (data no shown).
Table 2 Comparison of anthropometric, nutritional and urinary characteristics between quartiles (Q1 and Q4) of animal:plant protein ratio of 6- to 12-year-old participants of the Dortmund Nutritional and Anthropometric Longitudinally Designed Study (Median values, 25th–75th percentiles)

BSA, Body Surface Area; 24-h UI, 24-h urinary iodine excretion; EAR, estimated average requirements.
* P trend was tested with PROC MIXED using the data of the total sample of 516 subjects with 1959 measurements. PROC MIXED was used to account for the dependence of multiple measurements within the same child.
† Ratios estimated as animal:plant protein (excluding dairy).
‡ Defined by using the EAR( 49 ) corrected for 15 % non-urinary losses. Differences were tested with χ2 test.
§ Animal protein, include fish, poultry and all meat products.
∥ Dairy protein, include milk and all milk products.
Results of the longitudinal linear mixed effects regression analyses (PROC MIXED) with urinary iodine excretion (24-h UI, μg/d) as dependent variable are shown in Table 3. β-Values for the individual time invariant mean values of animal:plant protein ratios represent the difference of 24-h UI (μg/d) when the dietary animal:plant protein ratio differs by one unit between the individuals across time. β-Values for intra-individual change indicate the within-person changes in the 24-h UI (μg/d) for a unit of change in animal:plant protein ratio across time. A higher animal:plant protein ratio was significantly associated with higher iodine excretion, before (model 1), and after adjustment for Na excretion (model 2); however, β-values diminished after adjustment for Na excretion. Overall, the effect strength was greater between individuals than within individuals (different β-values and significance). Fig. 1 graphically illustrates the proportions of protein sources by animal:plant protein ratio category (low, middle and high) and its effect on 24-h UI, obtained from adjusted means of the 24-h UI from the PROC MIXED model.
Table 3 Results of the linear mixed effects regression models (PROC MIXED) for the association between ratios of animal:plant protein intake and 24-h urinary iodine excretion (24-h UI, μg/d) in 516 6- to 12-year-old schoolchildren participants of the Dortmund Nutritional and Anthropometric Longitudinally Designed Study. Longitudinal analysis of 1959 measurements collected repeatedly between 1993 and 2010 stratified by sex (β-Values and 95 % confidence intervals)
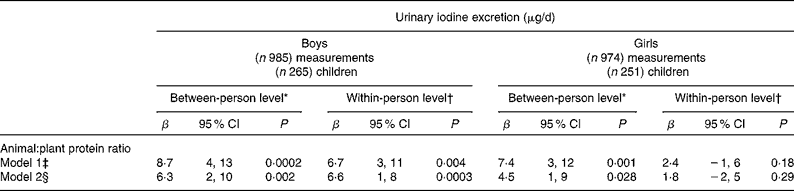
* β-Values of the between-person level indicate a difference in 24-h UI in μg/d for a change of one unit of the ratio of animal:plant protein intake between the individuals across time.
† β-Values of the within-person level indicate an intra-individual change in 24-h UI (in μg/d) excretion with a one unit change in the animal:plant protein ratio within one child.
‡ Model adjusted for the variables: age (years); time period (1993–2010), time × time; total energy (MJ/d); 24-h urine volume (ml/d); milk and all milk products (g/d).
§ Model additionally adjusted as for model 1 plus 24-h Na excretion (mg/d).

Fig. 1 Least-squares means (95 % confidence intervals) of 24-h urinary iodine excretion (24-h UI, μg/d) by category of animal:plant protein ratio in (a) boys and (b) girls. Model 2 (Table 3) of the multivariable-adjusted linear mixed models was used for prediction. P for continuous trend refers to the P values obtained in linear regression models with 24-h UI as continuous variable. , Between-person changes (P for trend = 0·0023 in boys and P for trend = 0·028 in girls);
, within-person changes (P for trend = 0·0003 in boys and P for trend = 0·29 in girls). Medians of animal:plant protein ratio protein in low (Q1), middle (Q2–Q3) and high (Q4) categories were in boys: 0·48, 0·90 and 1·61; in girls: 0·47, 0·92 and 1·54, respectively. The pie graph presents the different proportions of protein sources in the categories of animal:plant protein ratios: animal (grey), dairy (white) and plant-based (black). Animal protein contribution by ratios category was (a) in boys: low 19 %, middle 31 % and high 45 %; (b) in girls: low 20 %, middle 33 % and high 46 %, respectively.
In addition, a separate analysis was conducted using the same PROC MIXED model to evaluate the strength of association of the animal:plant protein ratio and 24-h UI, including dairy protein in the animal:plant protein ratios (dairy+animal-/plant-based protein). The results of this analysis were similar to those presented in Table 3; the effect estimates were only slightly enhanced. For instance, in boys at the between-person level, the effect estimate increased from 8·7 μg/d (ratios excluding dairy) to 8·9 μg/d (including dairy) (model 1) and 6·3 to 9·1 μg/d (model 2). In girls, the corresponding values were 7·4 μg/d and 7·4 μg/d (same values) (model 1) and 4·5 to 7·4 μg/d (model 2) respectively.
Discussion
In the present longitudinal analysis of children consuming a diet characterised by relatively high protein intake (typical Western diet), we examined the importance of changes in the proportion of animal to plant protein for changes in iodine status, and the corresponding role of salt intake. Our findings suggest that a high ratio of animal:plant protein is associated with greater iodine excretion (μg/d), even if other relevant dietary sources of iodine (e.g. salt intake) are controlled for.
Suggestive evidence of a higher risk of sub-optimal iodine status has already been proposed when diets lack animal food products. This notion builds on studies mainly conducted in adults, who are vegetarians or vegans, which may lead to deficits in iodine as well in other micronutrients( Reference Krajcovicová-Kudlácková, Bucková and Klimes 16 , Reference Remer, Neubert and Manz 17 , Reference Leung, Lamar and He 50 ), whereas in children this information is scarce. Most previous cross-sectional studies in children characterising iodine status reported urinary iodine concentration (μg/l), but usually lacked data on the dietary habitual intake( Reference Perrine, Sullivan and Flores 6 , Reference Watutantrige Fernando, Barollo and Nacamulli 7 , Reference Vandevijvere, Mourri and Amsalkhir 12 , Reference Verkaik-Kloosterman, van't Veer and Ocké 51 , Reference Skeaff, Thomson and Wilson 52 ). In our longitudinal analysis, we were able to simulate different dietary protein patterns, and quantify the effect of lower animal food intake, resembling almost a vegetarian diet, on iodine excretion. One example to illustrate the apparent effect of increasing the animal:plant protein ratio on the 24-h UI can be deduced from our model. Assuming a 50 g total protein intake (approximately average protein intake for boys in the low-animal:plant protein ratio), made up by 20 g from dairy, 10 g from animal and 20 g from plant protein, the estimated animal:plant protein ratio is 0·5. Increasing the animal:plant protein ratio by one unit would modify the proportion of the sources, i.e. an intake of 18 g from animal protein, and 12 g of plant-protein, keeping the dairy protein in 20 g. Theoretically, according to our adjusted model (model 2), about 6 μg/d higher 24-h UI would be expected between boys with otherwise similar dietary intakes (including Na intake) and within the same boy, with one unit increase in animal:plant protein ratio. In girls, no association was found between an increase in the ratio and changes at the within person level, possibly due to smaller individual changes that we were not able to fully identify.
One possible mechanism thought to explain the positive association of animal:plant protein ratios with iodine excretion is the suggested association between Na intake and consumption of animal foods. The main dietary sources of Na ingested by children in Europe and Northern America are processed foods, often of animal origin( Reference Libuda, Kersting and Alexy 28 , 53 , Reference Huybrechts, De Keyzer and Lin 54 ). Longitudinal analysis suggests that meat intake (especially cold cuts) increases 24-h Na excretion as earlier reported in a group of DONALD children( Reference Alexy, Cheng and Libuda 27 ). These findings were confirmed by our analysis as we observed higher Na intake in the high animal:plant protein ratio category (Table 2). However, the positive association of animal:plant protein ratio with 24-h UI in the longitudinal PROC MIXED model remained significant after adjustment for Na excretion, albeit with somewhat reduced effect estimates (β-values of the animal:plant protein ratio). Therefore, the association between animal:plant protein ratio and 24-h UI in children seems to be only partially mediated by the amount of Na intake. It is interesting that when including dairy protein in the animal protein fraction from the animal:plant protein ratios predicted a quite similar increase of 24-h UI, compared to the β-values when the dairy is excluded from the ratios. It is possible that the relatively high but constant contribution from the dairy group observed over the whole range of animal and plant protein categories (Fig. 1, pie graphs) explains the observed results.
Traditionally, the iodine status of populations is measured in terms of urinary iodine concentration (μg/l), generally obtained from spot urine samples( Reference Andersson, Karumbunathan and Zimmermann 55 , Reference Zimmermann and Andersson 56 ). More recently, it has been acknowledged that whenever feasible, 24-h UI (μg/d) should be the ‘reference standard’ for measuring iodine intake, as it incorporates urine volume, which can strongly affect dilution of the sample and therefore iodine concentration( Reference Johner, Gunther and Remer 4 , Reference Dary 57 , Reference Vejbjerg, Knudsen and Perrild 58 ). With our analysis, which comprises a large number of 24-h urine samples (approximately 2000), we were able to confirm the importance of using 24-h UI (μg/d) instead of concentration (μg/l) to define the iodine status. Based on the WHO 100 μg/l cut-off for iodine adequacy, our observed median concentration in boys (109 μg/l) suggests sufficiency, and in girls (94 μg/l) modest insufficiency. Due to an often better hydration status in females compared to males( Reference Ebner and Manz 59 , Reference Montenegro-Bethancourt, Johner and Remer 60 ), such a difference is not unexpected; accordingly, energy-corrected 24 h excretion rates of iodine (Table 1) denoted that no sex differences exist.
As trends towards alternative nutrition practices, i.e. preferences to consume organic foods, use of no iodised salt, and vegetarian diets, are becoming more popular in European children( Reference Gorczyca, Prescha and Szeremeta 61 , Reference van Winckel, Vande Velde and De Bruyne 62 ), the issue of what constitutes an optimum diet and micronutrient composition has important public health implications. In an effort to achieve healthier diets, even slight changes in food sources, especially in animal products (a decline in dairy, eventually, increasing grain intakes), may potentially negatively affect micronutrients such as iodine as recently observed by Perrine et al. ( Reference Perrine, Sullivan and Flores 6 ) in a group of USA schoolchildren. Accordingly, when the subjects of the present analysis were divided into low v. high animal:plant protein ratios, the proportion of children with iodine status below EAR was higher in the lower animal foods consumers, although the differences were not significant. Also, the observed effect estimates for a change of animal:plant protein ratio for 24-h UI were small. A change in the animal:plant protein ratio of about one unit predicted a difference in 24-h UI of approximately 6 μg/d, equalling to about 10 % of the EAR. Nonetheless, this dietary factor should not be overlooked as a long-term etiological risk factor that might contribute to cause iodine deficiency disorders in children, such as cognitive performance as literature suggests( Reference Gordon, Rose and Skeaff 63 , Reference Santiago-Fernandez, Torres-Barahona and Muela-Martínez 64 ). In particular, with regard to the planned salt reduction measures that will also reduce the intake of iodised salt (the main iodine source for children), the achievement of an adequate intake in nearly all children is an important goal.
Discrepancies on the adequate amounts and sources of protein intake in children exist. It has been shown in epidemiological and clinical studies that the amounts of dietary protein intake as well as its sources are important in the prevention or risk increase of chronic diseases, as reviewed in the report by Hörnell et al. ( Reference Hörnell, Lagström and Lande 19 ). In the present study population, the median protein intake (1·7 g/kg) was higher than the 0·9 g/kg recommended for German children( 65 ); however, the overall contribution was within the range of representative German schoolchildren's dietary protein intake (10–15 % of energy)( Reference Mensing, Heseker and Richter 66 ).
Strengths of the present study included the longitudinal design, with two to seven repeated measurements throughout childhood for each individual and the available data in a relatively large number of children, which better allowed us to use models more suitable for the evaluation of the association of diet with urinary biomarkers (24-h UI) than models based on cross-sectional designs. Even though single 24-h UI collections do not reflect habitual iodine intake( Reference Vejbjerg, Knudsen and Perrild 58 ), the repeated measures of dietary intakes concomitant with analysis of urine samples in the same subject allows both assessment of the gross average iodine status over years and the influence thereupon of the children's changes in dietary components (from year to year).
As it is recognised, the participants of the DONALD Study are characterised by a higher socioeconomic status than the overall German population( Reference Buyken, Alexy and Kersting 31 ), and do not represent subjects with rather extreme dietary characteristics, e.g. a very high intake of Na, as present in other population groups( Reference Mensing, Heseker and Richter 66 ). However, despite the homogeneity of our sample, we could still observe significant associations regarding the maternal education with the children's preferred protein sources. The higher proportion of mothers with a higher level of education in the low animal:plant protein ratio category suggest that homes with better educated mothers probably are more likely to adopt more healthy food patterns, which potentially may in turn lead to a moderate deficiency in iodine supply.
In conclusion, the present study shows a positive association between higher ratio of animal:plant protein and iodine status in children. Accordingly, children with healthier and therefore more desirable food patterns (lower intake of animal protein) demonstrate somewhat lower iodine excretion. As many children in Western populations are probably already in the highest animal:plant protein ratio, increasing animal protein intake would not be advisable. To nonetheless ensure adequate iodine nutrition, alternative prophylactic measures to prevent iodine deficiency are needed. One solution could be the mandatory use of iodised salt in processed foods and for bread( Reference Vandevijvere, Mourri and Amsalkhir 12 , Reference Harris, Jooste and Charlton 67 , Reference Clifton, Hodyl and Fogarty 68 ) (on a voluntary basis, which currently in Germany is only < 30 %( Reference Johner, Thamm and Nöthlings 5 )) in addition to the use of iodised salt at home. Furthermore, substituting some of the meat protein for animal foods with higher iodine content (e.g. fish) may also benefit the iodine nutrition in those consuming Western type diets. Altogether, the existing dietary recommendations that advise the limitation in the intake of animal protein and to increase the plant-based foods to prevent chronic diseases do not contradict with adequate iodine nutrition; however, potentially, they may do so if no further actions towards general iodine prophylaxis measures are taken.
Acknowledgements
The participation of all children and their families in the DONALD Study is gratefully acknowledged. We also thank the staff of the Research Institute of Child Nutrition for carrying out the anthropometric measurements, dietary data and for urine laboratory analyses.
The DONALD Study was supported by the Ministry of Innovation, Science, Research and Technology of North Rhine-Westphalia, Germany. G. M.-B. received a research fellowship from the Deutscher Akademischer Austausch Dienst.
The authors' contributions are as follows: T. R. and G. M.-B. designed the research; T. R. was responsible for the urine analysis; G. M.-B. with S. A. J. performed statistical analysis; P. S. critically revised the manuscript for important intellectual content. All authors were involved in data interpretation and reviewing the manuscript. G. M.-B. wrote the manuscript and T. R. had primary responsibility for final content. All authors made contributions to and approved the final manuscript.
The authors declare no personal or financial conflicts of interest.