Introduction
Many psychologists and psychiatrists believe that humans are the only animals who can become depressed. Although humans have two large cerebral hemispheres covered with an extensive cerebral cortex, emotions are regulated by brain structures that were already present in our earliest vertebrate ancestors about 560 million years ago. We suggest that dysfunction of these ancient brain structures is the primary cause of mood, anxiety, and stress disorders.Reference Loonen and Ivanova1, Reference Loonen and Ivanova2 Of special interest in this regard is the habenula (Figure 1), because this structure regulates the intensity of appetitive-searching and adversity-avoiding behavior. This small nuclear complex, measuring about 20–30 cubic millimeters in each hemisphere,Reference Batalla, Homberg and Lipina3 which was well conserved during the evolution of vertebrates,Reference Loonen and Ivanova4, Reference Loonen and Ivanova5 can only be studied with high-resolution functional magnetic resonance imaging techniques,Reference Batalla, Homberg and Lipina3 and has hitherto not received proper attention in functional neuroimaging studies.
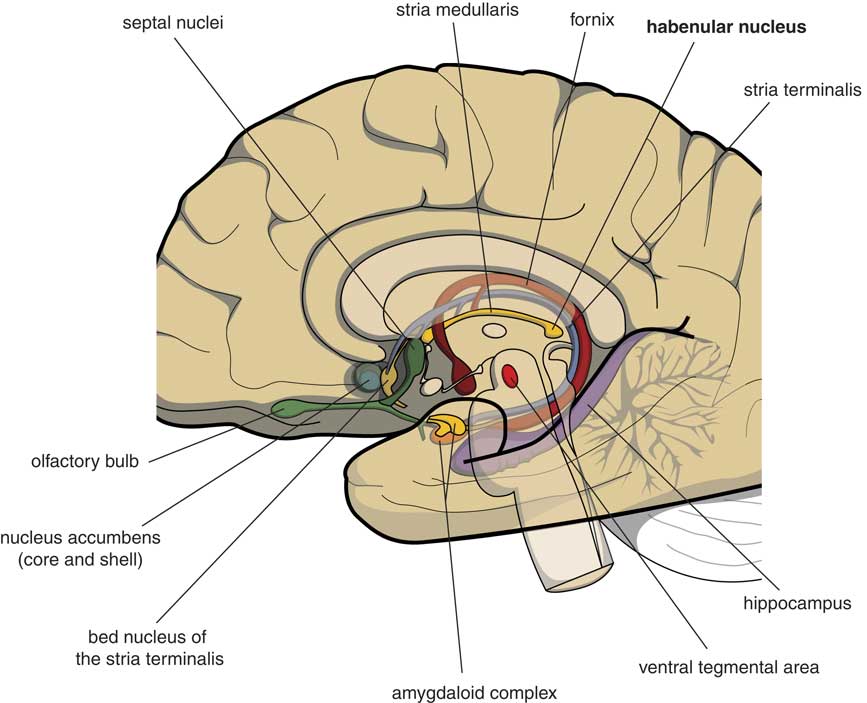
Figure 1 Mesial view on the right cerebral hemisphere (original figure).
The starting point for the development of our model was the idea that behavior can be considered as a mechanism by which organisms adapt to the ever-changing circumstances within their biosphere: the capacity to obtain food or procreative opportunities and the ability to escape from dangers is shown by all free-moving animals. The competence to show appetitive-searching (reward-seeking) and adversity-avoiding (misery-fleeing) activity must therefore have been present in our earliest evolutionary vertebrate ancestors living about 560 million years ago, and their brains, which are comparable with the modern lamprey’s cerebrum, and lack the human cerebral cortex, must have been capable of regulating these behaviors.Reference Loonen and Ivanova4, Reference Loonen and Ivanova5 Yet the question arises as to whether or not the human cerebral cortex nevertheless initiates and controls reward-seeking and misery-fleeing behavior. For this reason, it is of interest to study the evolutionary development of the brain in vertebrates.Reference Loonen and Ivanova1, Reference Loonen and Ivanova4, Reference Loonen and Ivanova5 This cannot be accomplished by studying the fossil remains of these ancestors, but we can use the idea that during several stages of evolution the development of some representatives of the relevant phases stopped. Current representatives of these animals are believed to have essentially the same appearance as the animals living several hundred million years ago. Hence, the brains of lampreys, sharks, lungfish, frogs, turtles, opossums, rats, and monkeys correspond to the brains of our human ancestors from about 560 million years ago until the present day.Reference Moreno and González6
The First Vertebrates
The first vertebrates had a brain comparable to the modern lamprey. The brain of the first vertebrates already had a primitive hemisphere, but this was small and insignificant. Next to this hemisphere, the lamprey’s forebrain also includes a medial pallium (cortical tissue), which finally gave rise to the human hippocampus (Figure 2). In humans, motor activity is initiated and controlled by the cerebral cortex, but this does not appear to be the case in the lamprey. The subpallium of the lamprey contains an extrapyramidal system, which has a similar composition and organization to its human subcortical equivalent but which directs motor centers within the lower brain, controlling motor behavior from there.Reference Robertson, Kardamakis and Capantini7 Similar to the human system, the proximal part of this lamprey extrapyramidal equivalent is termed the “striatum,” and the distal area connected with the motor centers is called the “pallidum.” The activity of the striatum is regulated by dopaminergic neurons originating within the animal’s midbrain, which is also comparable with the human extrapyramidal system.Reference Loonen and Ivanova8 The activity of these dopaminergic neurons, as well as that of adrenergic and serotonergic centers within the upper brainstem are, in turn, regulated by the habenula (Hb). The activity of this motor control system is influenced by a specific nucleus termed the “habenula-projecting globus pallidum” (GPh). The GPh controls the activity of the extrapyramidal system by affecting the activity of midbrain dopaminergic neurons;Reference Robertson, Kardamakis and Capantini7, Reference Stephenson-Jones, Kardamakis, Robertson and Grillner9 it inhibits an animal’s behavior when motor activity is not rewarding.
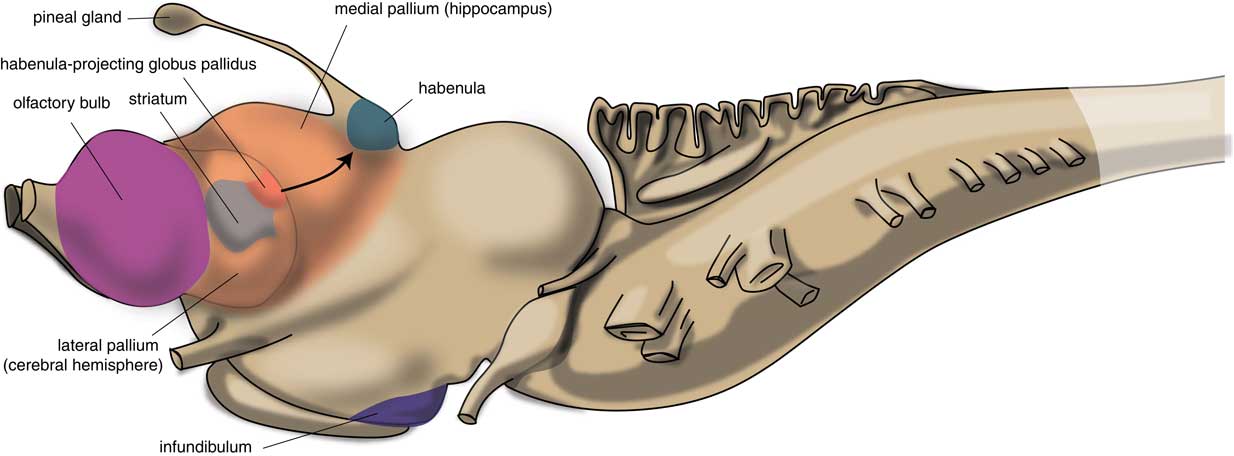
Figure 2 Position of striatum and habenula-projecting globus pallidus in the lamprey (adapted from Stephenson-Jones et al.Reference Stephenson-Jones, Kardamakis, Robertson and Grillner9).
The First Land Animals
A later stage of development is represented by animals with a brain comparable to that of frogs. In our amphibian-like ancestors, the subpallium of the lamprey was maintained as the extended amygdala, and a new striatopallidum had developed as a starting point for the human extrapyramidal system. The amphibian-like pallium did not at that point have the same function as the human cerebral cortex. The ventral and ventrolateral parts of this pallium were later included into the corticoid amygdala, and the dorsolateral (largely) and medial parts into the hippocampus (Figure 3). The human amygdaloid complex can be neuroanatomically divided into the “deep nuclei” and “superficial nuclei,” which have cortex-like cell types and “remaining” or “extended amygdalar nuclei,” deriving from the lamprey striatopallidum.Reference Moreno, Morona and López10, Reference González, Morona, Moreno, Bandín and López11 The bed nucleus of the stria terminalis represents the lamprey’s pallidum and may contain the amphibian GPh.Reference Loonen and Ivanova1, Reference Loonen and Ivanova5
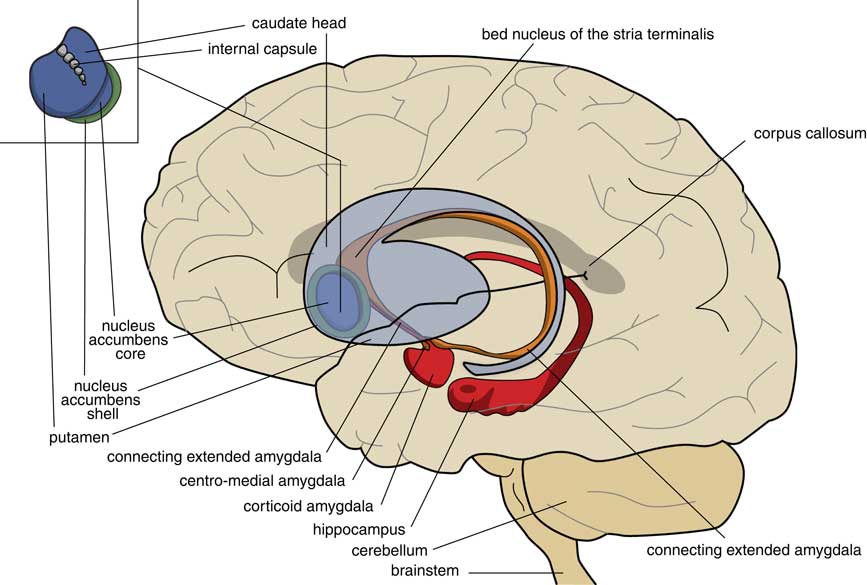
Figure 3 Position of the ancestor’s endbrain within the human brain (adapted from Loonen and IvanovaReference Loonen and Ivanova1 and Loonen et al.Reference Loonen, Schellekens and Ivanova29). Corticoid amygdala and hippocampus derive from our first vertebrate ancestors’ pallium (lamprey-like telencephalon) and extended amygdala (centromedial amygdala, the bed nucleus of the stria terminalis, and their connecting parts) from this ancestor’s striatopallidum. The nucleus accumbens derives from our amphibian-like ancestor’s striatum.
New Cerebral Capacities of Mammals
Primitive reptiles with a turtle-like brain precede more developed reptiles and birds on the one hand and mammals on the other. In our mammalian ancestors, the cerebral neocortex and dorsal thalamus gradually developed into its current human form,Reference Butler12, Reference Butler13 and, parallel to the expansion of the cerebral cortex, a dorsal extrapyramidal circuitry evolved in modular fashion.Reference Robertson, Kardamakis and Capantini7 These cortico-striato-thalamo-cortical circuits serve to correct the intensity of the intracortical stimulation of the output-generating frontal cortex.Reference Loonen and Ivanova8 Especially noteworthy are the extrapyramidal circuits, including the most ancient ventral part of the striatum, the nucleus accumbens, regulating motivation to show reward-seeking (core part) and misery-fleeing (shell part) behavior.
The Connectivity of the Habenula
The output structures of the human habenuloid complex are highly similar to the situation in the lamprey.Reference Loonen and Ivanova4 Information encoded by the lateral habenula (LHb) and the medial habenula (MHb) is transmitted through the fasciculus retroflexus (FR) axon bundle to several midbrain monoaminergic nuclei.Reference Batalla, Homberg and Lipina3, Reference Hikosaka14 The majority of the output, however, is given to two specific midbrain areas: the rostromedial tegmental nucleus (RMTg) and the interpeduncular nucleus (IPN) (Figure 4). The RMTg is a GABAergic midbrain nucleus that inhibits the activity of the dopaminergic neurons of the VTA and SNc.Reference Batalla, Homberg and Lipina3 The IPN is a singular, unpaired structure located at the ventral midline of the midbrain with widespread peptidergic ascending and descending projections.Reference Klemm15, Reference Morley16 The major efferent pathways originating in the IPN project to the dorsal tegmental nucleus,Reference Morley16 the VTAReference Klemm15 and the raphe nuclei.Reference Klemm15, Reference Bianco and Wilson17
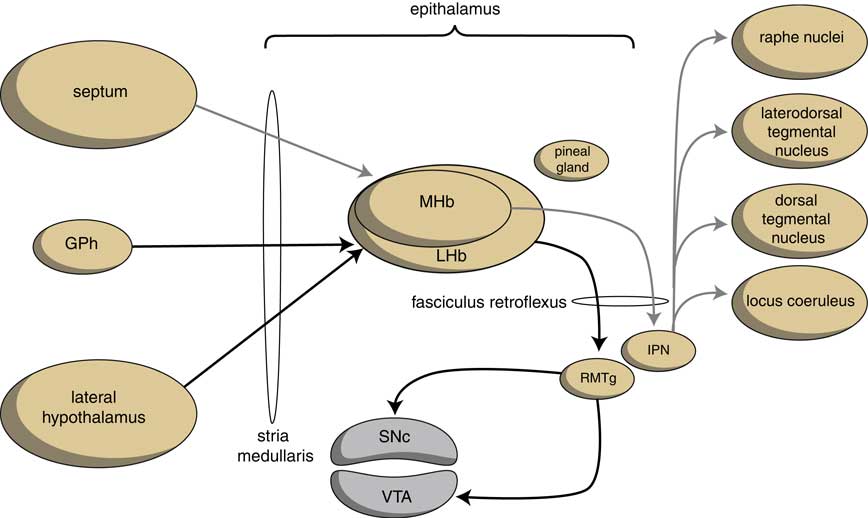
Figure 4 Simplified scheme showing the connectivity of the medial and lateral habenula (adapted from HikosakaReference Hikosaka14 and Loonen et al.Reference Loonen, Schellekens and Ivanova29). GPh=habenula-projecting pallidum; IPN=interpeduncular nucleus; LHb=lateral habenula; MHb=medial habenula; RMTg=rostromedial tegmental nucleus; SNc=substantia nigra pars compacta; VTA=ventral tegmental area.
The Hb receives signals from the septum, hippocampus, ventral pallidum, lateral hypothalamus, globus pallidus, and other areas of the basal ganglia.Reference Batalla, Homberg and Lipina3 The main input to the MHb comes from the septum, in particular the medial septum and the adjacent nucleus of the diagonal band of Broca.Reference Batalla, Homberg and Lipina3, Reference Loonen and Ivanova5 This input structure may in turn receive input from the corticoid amygdala via the hippocampus and fornix.Reference Loonen and Ivanova5 The LHb primarily receives glutamatergic afferents from the preoptic area, lateral hypothalamus, the globus pallidus, the anterior cingulate, and medial prefrontal cortex.Reference Loonen and Ivanova5 The lateral hypothalamus may in turn receive input from the corticoid and extended amygdala.Reference Loonen and Ivanova5
The human habenuloid complex, therefore, is probably (at least partly) controlled by the amygdaloid complex in regulating the intensity of reward-seeking and distress-avoiding (misery-fleeing) behavior (Figure 5). The amygdala is well-known for playing an important role in selecting that sensory input that is particularly relevant and should receive priority in taking action (salience attribution).Reference Loonen and Ivanova18, Reference Loonen and Ivanova19 The process of reward pursuing consists of at least three psychological components: learning to value (attentive salience); incentive salience or “wanting”; and experiencing pleasure resulting in “liking.”Reference Berridge, Robinson and Aldridge20, Reference Berridge21 The first component is believed to be addressed by the amygdala,Reference Balleine and Killcross22, Reference Morrison and Salzman23 which can “learn” to appreciate sensory appetitive information within the context of external and internal circumstances and to initiate a proper response. The second component is regulated by mesocorticolimbic mechanisms,Reference Berridge, Robinson and Aldridge20 with a central role for the nucleus accumbens (NAcb).Reference Kelley24, Reference Cardinal25 Important neural substrates for this system are dopaminergic mesolimbic and mesocortical projections coming from the ventral tegmental area.Reference Berridge and Robinson26, Reference Bromberg-Martin, Matsumoto and Hikosaka27 The amygdala can regulate the activity of the nucleus accumbens via its connectivity with the LHb and VTA. The same is probably true for the regulation of the process of distress avoidance via its connectivity with the MHb and IPN. This connectivity may play an important role in psychotic disorders (salience dysregulation syndrome)Reference Loonen and Ivanova19 and addiction,Reference Batalla, Homberg and Lipina3, Reference Loonen, Schellekens and Ivanova29 as well as mood and anxiety disorders.Reference Loonen and Ivanova2, Reference Loonen and Ivanova18, Reference Loonen and Ivanova28, Reference Loonen, Kupka and Ivanova30
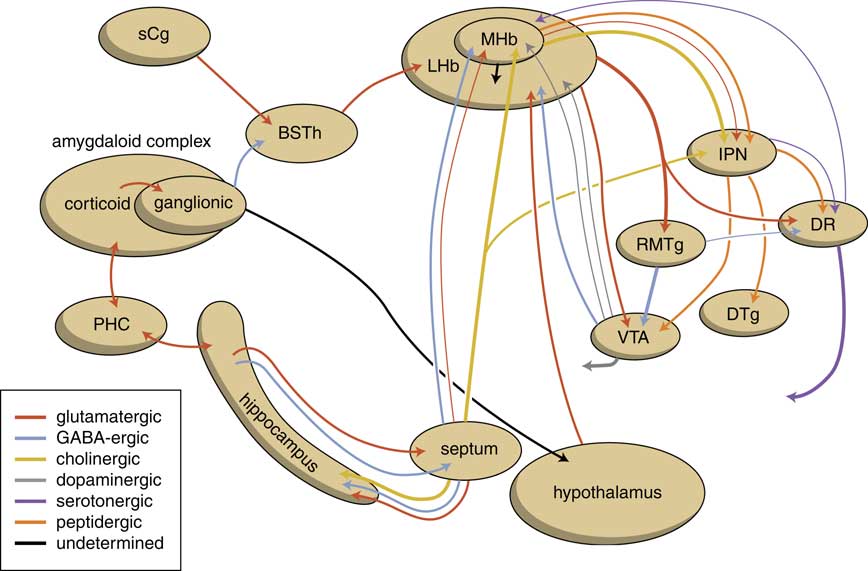
Figure 5 Scheme showing the neurochemical connectivity of the amygdalo-hippocampal system to the midbrain through the habenular complex (adapted from Loonen and IvanovaReference Loonen and Ivanova1, Reference Loonen and Ivanova5). BSTh=habenula-projecting part of the bed nucleus of the stria terminalis; DR=dorsal raphe nucleus; DTg=dorsal tegmental nucleus; IPN=interpeduncular nucleus; LHb=lateral habenula; MHb=medial habenula; PHC=parahippocampal cortex; RMTg=rostromedial tegmental nucleus; sCg=subgenual cingulate gyrus; VTA=ventral tegmental area.
The Role of the Habenula
Although the cerebral cortex may play an important role in determining an exact behavioral response, it is unlikely that it took over the primary role of the subcortex. The amygdaloid complex initiates reward-seeking and misery-fleeing behavior by giving output via its striatopallidal part to the hypothalamus and midbrain.Reference Loonen and Ivanova5, Reference Loonen and Ivanova18 The hypothalamus is connected with the lateral Hb (Figure 4), which also receives input from the nuclear amygdala via the habenular-projecting part of the bed nucleus of the stria terminalis (BSTh; Figure 5).Reference Loonen and Ivanova1, Reference Loonen and Ivanova5 The corticoid amygdala and hippocampus are also connected with the medial Hb.Reference Loonen and Ivanova1, Reference Loonen and Ivanova5 By regulating the activity of monoaminergic centers within the upper brainstem, the Hb modulates motivation to show misery-fleeing and reward-seeking behavior. This pathway includes the fasciculus retroflexus, midbrain nuclei, and the nucleus accumbens.Reference Loonen and Ivanova1
The Habenula-Projecting Pallidum in Humans
But what happened to our ancestor’s habenula-projecting globus pallidus (GPh), which in lamprey evaluates the results of reward-seeking behavior?Reference Stephenson-Jones, Kardamakis, Robertson and Grillner9 Evidence exists that a homolog of this structure still exists in humans.Reference Loonen and Ivanova1, Reference Loonen and Ivanova5 Habenula-projecting glutamatergic neurons have been identified within the border region of the globus pallidus (GPb). These GPb neurons also appear to exist and have the same function in mice.Reference Stephenson-Jones, Yu and Ahrens31 The GPb neurons may be involved in “evaluation behavior,” where the intensity is regulated by the dorsal extrapyramidal circuits—complex cognitive (reasoning) and motor (kinesia) patterns. Corresponding habenula-projecting neurons within the ventral pallidum (VPh) may be involved in evaluating the activity of the circuits including the core and shell parts of the nucleus accumbens: these include, respectively, reentry circuits regulating motivation to show reward-seeking and misery-fleeing behavior. Habenula-projecting neurons of the bed nucleus of the stria terminalis (BSTh) may play this role with respect to the emotional responses that are regulated by the amygdaloid complex. Hence, our ancestors’ GPh may have split up into three parts in humans: the GPb, VPh, and BSTh. However, neither VPh nor BSTh neurons have, until now, been proven to be true homologs of lamprey GPh neurons.
Dysfunction of these human GPh homologs is likely to play an important role in causing symptoms of several mental disorders.Reference Loonen and Ivanova1 Neuroplastic changes within circuitry running from the amygdalar/hippocampal complex to the striatum via the habenular complex and midbrain monoaminergic centers may take an essential position within the pathogenesis of such disorders. In our opinion, the study of the connectivity between the amygdala, pallidum, and habenula with high-resolution neuroimaging techniques is indicated.
Conclusions
Although the cerebral cortex, with its highly developed neuronal circuitry and recurrent collateral connectivity, is by far the most likely candidate as a brain structure to process input and to estimate the exact behavioral output, vital emotions (e.g., pain, fear, happiness, and pleasure) were probably already used by our most primitive ancestors to regulate their behavior. Neuroplastic changes occurring within this ancient circuitry of the human brain that derives from the early vertebrate forebrain should therefore be considered to play an essential role in the pathogenesis of depressive disorders and in the mechanism of antidepressant treatments.Reference Loonen and Ivanova28
Disclosures
The authors hereby declare that they have no conflicts of interest to disclose.