1. Introduction
The North China Craton (NCC) is one of the oldest known cratons in China, and has a unique evolutionary history. Based on the research of mantle xenoliths from the Palaeozoic kimberlites and Cenozoic basalts, the NCC underwent reactivation and dramatic lithospheric thinning (> 100 km) (Griffin et al. Reference Griffin, Zhang, O’Reilly and Ryan1998; Fan et al. Reference Fan, Zhang, Baker, Jarvis, Mason and Menzies2000; Menzies et al. Reference Menzies, Xu, Zhang and Fan2007; Zhu et al. Reference Zhu, Chen, Wu and Liu2011; Wu et al. Reference Wu, Yang, Xu, Wilde and Walker2019). Although some understanding of lithospheric thinning in the NCC has been obtained by petrology, geochemistry and geophysics, the initial timing and geodynamics mechanism are still controversial (Wu et al. Reference Wu, Lin, Wilde, Sun and Yang2005 a, Reference Wu, Xu, Gao and Zheng2008; Zhai et al. Reference Zhai, Fan, Zhang, Sui and Shao2007). The initial timing of thinning and destruction of the NCC ranges from Late Triassic (Duan et al. Reference Duan, Zeng, Yang, Liu, Wang and Zhou2014) to Late Jurassic (Jiang et al. Reference Jiang, Jiang, Ling and Ni2010) and Early Cretaceous (Wu et al. Reference Wu, Xu, Gao and Zheng2008). Regarding the geodynamics mechanism, there exist delamination (Deng et al. Reference Deng, Mo and Zhao1994, Reference Deng, Su, Niu, Liu, Zhao, Zhao, Zhou and Wu2007; Gao et al. Reference Gao, Rudnick, Carlson, McDonough and Liu2002, Reference Gao, Rudnick, Yuan, Liu, Liu, Xu, Ling, Ayers, Wang and Wang2004; Xu et al. Reference Xu, Gao, Wang, Wang and Liu2006 a, b; Windley et al. Reference Windley, Maruyama and Xiao2010) and thermo-chemical erosion (Menzies et al. Reference Menzies, Fan and Zhang1993; Xu et al. Reference Xu, Ma, Huang, Iizuka, Chung, Wang and Wu2004; Zheng et al. Reference Zheng, Griffin, O’Reilly, Yang, Li, Zhang, Zhang and Liou2006) models.
Adakites were first proposed by Defant & Drummond (Reference Defant and Drummond1990) on the basis of their geochemical features (e.g. high Sr/Y and La/Yb ratios; low Y and Yb contents). Scholars proposed that adakites may have originated from either: partial melting of hot subducted oceanic crust (Defant & Drummond, Reference Defant and Drummond1990); partial melting of the thickened lower crust (Muir et al. Reference Muir, Weaver, Bradshaw, Eby and Evans1995); or assimilation and fractional crystallization (AFC) processes associated with basaltic magma (Castillo et al. Reference Castillo, Janney and Solidum1999), the reaction of delaminated lower crust with mantle peridotite (Gao et al. Reference Gao, Rudnick, Yuan, Liu, Liu, Xu, Ling, Ayers, Wang and Wang2004) or the mixing of basaltic and felsic magmas (Guo et al. Reference Guo, Nakamuru, Fan, Kobayoshi and Li2007). The various origins of adakites play a critical role in understanding the growth and evolution of the crust (Guo et al. Reference Guo, Fan and Li2006). Large-scale Mesozoic magmatism is one type of geological evidence of the lithospheric thinning and destruction of the NCC (Wu et al. Reference Wu, Lin, Wilde, Sun and Yang2005 a; Xu et al. Reference Xu, Li, Pang and He2009; Yang et al. Reference Yang, Wu, Wilde, Liu, Zhang and Xie2009). It is noteworthy that a large number of adakitic rocks (c. 175–110 Ma) have been identified from the Mesozoic magmatic activity, distributed along the edge of the NCC (Fig. 1a; Zhang & Wang, Reference Zhang and Wang2001; Xiong et al. Reference Xiong, Liu and Zhu2011). Because the age and distribution of adakites are consistent with the thinning range of the NCC, the age, source and origin of adakites can provide an important window into the processes and mechanisms of lithospheric thinning and destruction of the NCC.

Fig. 1. (a) Distribution of Mesozoic adakites (ages mainly 175–110 Ma) in the eastern NCC (after Zhang & Wang, Reference Zhang and Wang2001; Xiong et al. Reference Xiong, Liu and Zhu2011); and (b) geological map and distribution of Mesozoic magmatic rocks in the Liaodong Peninsula (modified after Wu et al. Reference Wu, Yang, Wilde and Zhang2005 b; Yang et al. Reference Yang, Sun, Chen, Wilde and Wu2007 a).
The Liaodong Peninsula is one of the most important parts of the NCC, which also underwent complex magmatic activity and dramatic lithospheric thinning (Fig. 1b; Zhu et al. Reference Zhu, Chen, Wu and Liu2011). Studies shown that a large number of Mesozoic magmatic rocks, such as A-type granite, mafic rocks, calc-alkaline I-type rocks and adakites, are distributed in the Liaodong Peninsula (Wu et al. Reference Wu, Xu, Gao and Zheng2008; Liu et al. Reference Liu, Ji, Shen, Guang and Davis2011, Reference Liu, Shen, Ji, Guan, Zhang and Zhao2013; Wang et al. Reference Wang, Burov, Gumiaux, Chen, Lu, Mezri and Zhao2015). These Mesozoic rocks indicate that it is an important place to study the thinning of the NCC. Previous studies on these rocks have obtained some periodic results (Yang et al. Reference Yang, Wu, Zhang, Zhang and Wilde2004, Reference Yang, Sun, Zhang and Wilde2012; Wu et al. Reference Wu, Xu, Gao and Zheng2008; Duan et al. Reference Duan, Zeng, Yang, Liu, Wang and Zhou2014). However, the geodynamics setting of Early Cretaceous magmas still remains controversial (Sun et al. Reference Sun, Ding, Hu and Li2007; Xiao et al. Reference Xiao, Li, Feng, Qiu and Zhang2010; Zhu et al. Reference Zhu, Chen, Wu and Liu2011; Zhang, Reference Zhang2013). Recently, we discovered a set of Early Cretaceous magmatic rocks with adakitic features in Liaodong Peninsula (Fig. 2). In this paper, we use major- and trace-element compositions, zircon U–Pb ages and Hf isotopes to restrict their magma sources and origin. Our aim is to reveal the tectonic dynamic setting of the Early Cretaceous magmatic rocks in the Liaodong Peninsula, and provide new evidence for the mechanism of lithospheric thinning and destruction of the NCC.

Fig. 2. Geological sketch map of the Pulandian region with sample locations.
2. Geological setting and sample descriptions
The NCC is triangular in shape, with the Central Asian Orogenic belt, and the Qinling–Dabie and Sulu high–ultrahigh-pressure metamorphic belts, located to its north, south and east, respectively (Fig. 1a; Zhao et al. Reference Zhao, Sun, Wilde and Sanzhong2005; Liu et al. Reference Liu, Wei, Zhang, Chen and Zhang2020). The NCC was formed as a result of the collision between eastern and western blocks along the central orogenic belt during the Palaeoproterozoic Era (c. 1.85 Ga; Zhao et al. Reference Zhao, Sun, Wilde and Sanzhong2005; Zhai & Santosh, Reference Zhai and Santosh2011), after which the NCC remained relatively stable and formed sedimentary basins and thick sedimentary rocks. The NCC then began to activate during the Mesozoic Era, which resulted in the thinning and destruction of the lithosphere, and the formation of a large number of ore deposits, magmatic rocks and extensional structures (Wu et al. Reference Wu, Lin, Wilde, Sun and Yang2005 a; Zhu et al. Reference Zhu, Yang and Wu2012; Zhu & Xu, Reference Zhu and Xu2019).
Located in the eastern part of the NCC (Fig. 1a), the Liaodong Peninsula can be divided into three tectonic units: the Archean Liaobei block in the north, the Archean Liaonan block in the south and the Palaeoproterozoic Jiao-Liao-Ji orogenic belt (JLJOB) in the middle (Liu et al. Reference Liu, Nutman, Compston, Wu and Shen1992; Wu et al. Reference Wu, Yang, Wilde and Zhang2005 b). The basement rocks mainly consist of Early Archean tonalite, trondhjemite and granodiorite (TTG) suites in the Liaobei block; Late Archean diorite, tonalite and granodiorite in the Liaonan block; and Palaeoproterozoic Liaohe Group in the JLJOB (Lu, Reference Lu2004; Wu et al. Reference Wu, Yang, Wilde and Zhang2005 b). These basement rocks were then covered by Mesoproterozoic–Palaeozoic sedimentary strata (Yang et al. Reference Yang, Wu, Wilde and Liu2007 b). The reactivation of the NCC resulted in the widespread distribution (c. 20 000 km2) of Mesozoic intrusive rocks in the Liaodong Peninsula (Fig. 1b; Yang et al. Reference Yang, Wu, Wilde and Liu2007 b). These Mesozoic magmatic rocks, consisting of syenite, monzogranite, granodiorite and diorite, were mainly deposited during three episodes: (1) Late Triassic (mainly 230–210 Ma); (2) Jurassic (mainly 180–155 Ma); and (3) Early Cretaceous (mainly 131–106 Ma) (Li, Reference Li2019). In addition, minor Mesozoic mafic dykes also occur in in the Liaodong Peninsula (Fig. 1b).
The Pulandian area covers an area of about 17 km2 and is distributed in the western part of the Liaodong Peninsula (Fig. 1b). The Precambrian basement is mainly composed of Neoarchean gneissic complex (e.g. biotite plagioclase gneiss) and Mesoproterozoic quartz diorite (Fig. 2). The intrusive rocks are mainly composed of intermediate-acidic rocks, which include monzodiorite, granodiorite, porphyritic monzogranite and monzogranite. In this study, these intermediate-acidic rocks were collected in the Pulandian area for research (Fig. 2).
The monzodiorite (sample no. Yd2005) is grey-white in colour and contains plagioclase (50–55%), biotite (15–20%), K-feldspar (5–10%), quartz (5–10%) and minor hornblende (c. 5%) (Fig. 3a–c). Typical polysynthetic twinning can be seen in plagioclases (Fig. 3c). The granodiorite (sample nos Yd2006 and Yd2010) is medium-grained and comprises plagioclase (45–50%), K-feldspar (20–25%), quartz (15–20%) and minor hornblende and biotite (c. 5%) (Fig. 3d–i). Small amounts of fine-grained biotite and hornblende are found in the margins of large-grained plagioclase and quartz. Sample Yd2010 contains minor perthite (Fig. 3i). The porphyritic monzogranite (sample no. Yd2009) has porphyritic texture, consisting of phenocryst (c. 15%) and matrix (c. 85%) (Fig. 3j–l). The phenocryst mainly consists of quartz, K-feldspar, plagioclase and biotite (Fig. 3l). The matrix is composed of fine-grained plagioclase, quartz and minor biotite.
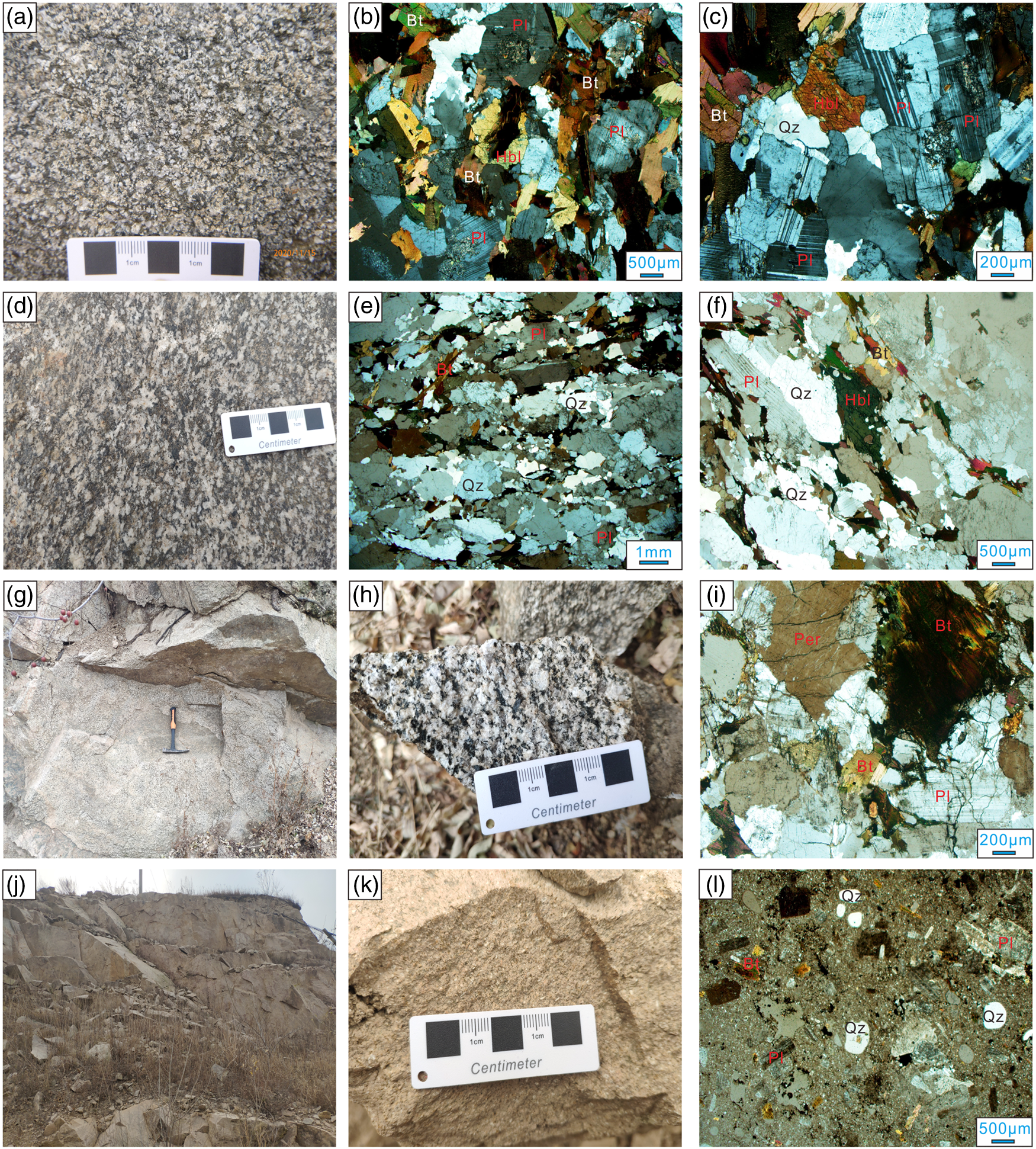
Fig. 3. Hand specimens and crossed-polarized light micrographs of rocks from Liaodong Peninsula from (a–c) monzodiorite (sample Yd2005); (d–f) granodiorite (sample Yd2006); (g–i) granodiorite (sample Yd2010); and (j–l) porphyritic monzogranite (sample Yd2009). Hbl – hornblende; Bt – biotite; Per – perthite; Pl – plagioclase; Qz – quartz.
3. Analytical methods
3.a. Zircon U–Pb dating
Zircons were separated from samples by magnetic and heavy-liquid separation methods at Langfang Hongxin Geological Exploration Technology Service Co. Ltd, Hebei Province, China. Zircons were observed and imaged to reveal their internal structure under cathode-luminescence (CL). Zircon laser ablation inductively coupled plasma mass spectrometry (LA-ICP-MS) U–Pb dating was conducted at the Laboratory of Mineral Resources Evaluation in Northeast Asia, Ministry of Nature Resources of China, Changchun (LMRENA), using a 193 nm ArF laser ablation system and Agilent 7900 ICP-MS. The denudation frequency and spot diameter of laser were 7 Hz and 32 μm, respectively. Standard zircon 91500 was adopted as the external standard for age calibration. These 91500 zircons yield a 206Pb/238U age range 1060.9–1069.1 Ma, with weighted mean 1065.0 Ma, which is consistent with the recommended values of 1064 Ma for 91500 zircons within analytical errors. NIST610 was used as an external standard and 29Si as an internal standard to normalize zircon trace-element contents. The isotope ratio was calculated using the LA-ICP-MS DATECAL program (Liu et al. Reference Liu, Hu, Gao, Günther, Xu, Gao and Chen2008). Pb correction methods according to Andersen (Reference Andersen2002) were followed. The calculated age and Concordia diagrams were calculated using the Isoplot3 program (Ludwig, Reference Ludwig2003).
3.b. Major- and trace-element analysis
Whole-rock major- and trace-element geochemical analyses were undertaken at LMRENA. Altered surfaces were removed from all samples and were ground to 200 mesh. The X-ray fluorescence (XRF) and ICP-MS were used for major- and trace-element testing, respectively. The trace-element analytical samples were prepared by dissolving in mixed acid (HF + HNO3) in Teflon bombs. The national standards GBW07103 and GBW07105 were adopted for element content correction. The analytical precision of major- and trace-element testing was 1% and 5%, respectively.
3.c. Zircon Hf isotopic analyses
Zircon in situ Lu–Hf isotope analyses were conducted using a Neptune-plus multicollector (MC) ICP-MS and NewWave UP213 laser ablation system at Yanduzhongshi Geological Analysis Laboratory, Beijing. The denudation frequency and spot diameter of the laser were 8 Hz and 50 μm, respectively. Standard zircons (e.g. GJ-1, Mud Tank, Penglai and 91500) were treated as precision control (Yuan et al. Reference Yuan, Gao, Dai, Zong, Günther, Fontaine, Liu and Diwu2008; Li et al. Reference Li, Long, Li, Liu, Zheng, Yang, Chamberlain, Wan, Guo, Wang and Tao2010). The testing steps and calibration methods used are described by Wu et al. (Reference Wu, Yang, Xie, Yang and Xu2006) and Guo et al. (Reference Guo, Chen, Zeng and Lou2012).
4. Analytical results
4.a. Zircon U–Pb ages
The zircon U–Pb results are provided in online Supplementary Table S1 (available at http://journals.cambridge.org/geo). Zircon grains are grey, subhedral to euhedral, 50–150 μm long and with aspect ratios of 1:1 to 2:1. Zircons from samples Yd2006, Yd2009 and Yd2010 all display typical oscillatory zoning in cathodoluminescence (CL) images, whereas no obvious oscillatory zoning was observed in zircons from sample Yd2005 (Fig. 4). Zircons from sample Yd2005 show variable Th (7–778 ppm) and U (51–303 ppm) contents, and Th/U ratios of 0.11–2.57. A total of 23 concordant analyses yielded a 206Pb/238U weighted mean age of 123.3 ± 1.6 Ma (Fig. 5a). Eighteen zircons from sample Yd2006 have Th content of 67–346 ppm, U content of 48–266 ppm and Th/U ratios of 0.71–1.44, and yielded a 206Pb/238U weighted mean age of 125.5 ± 1.5 Ma (Fig. 5b). Sixteen zircons from sample Yd2009 display Th content of 68–690 ppm, U content of 115–410 ppm and Th/U ratios of 0.34–2.07, giving a mean age of 120.0 ± 1.9 Ma (Fig. 5c). Twenty concordant zircon analyses from sample Yd2010 yielded Th content of 166–760 ppm, U contents of 194–463 ppm and Th/U ratios of 0.72–1.34, with a mean age of 127.1 ± 1.2 Ma (Fig. 5d). Their ages range from 127–120 Ma, suggesting that they were formed during the Early Cretaceous Epoch. All zircons have high Th/U ratios (> 0.1), suggesting a magmatic origin (Hoskin & Schaltegger, Reference Hoskin and Schaltegger2003).
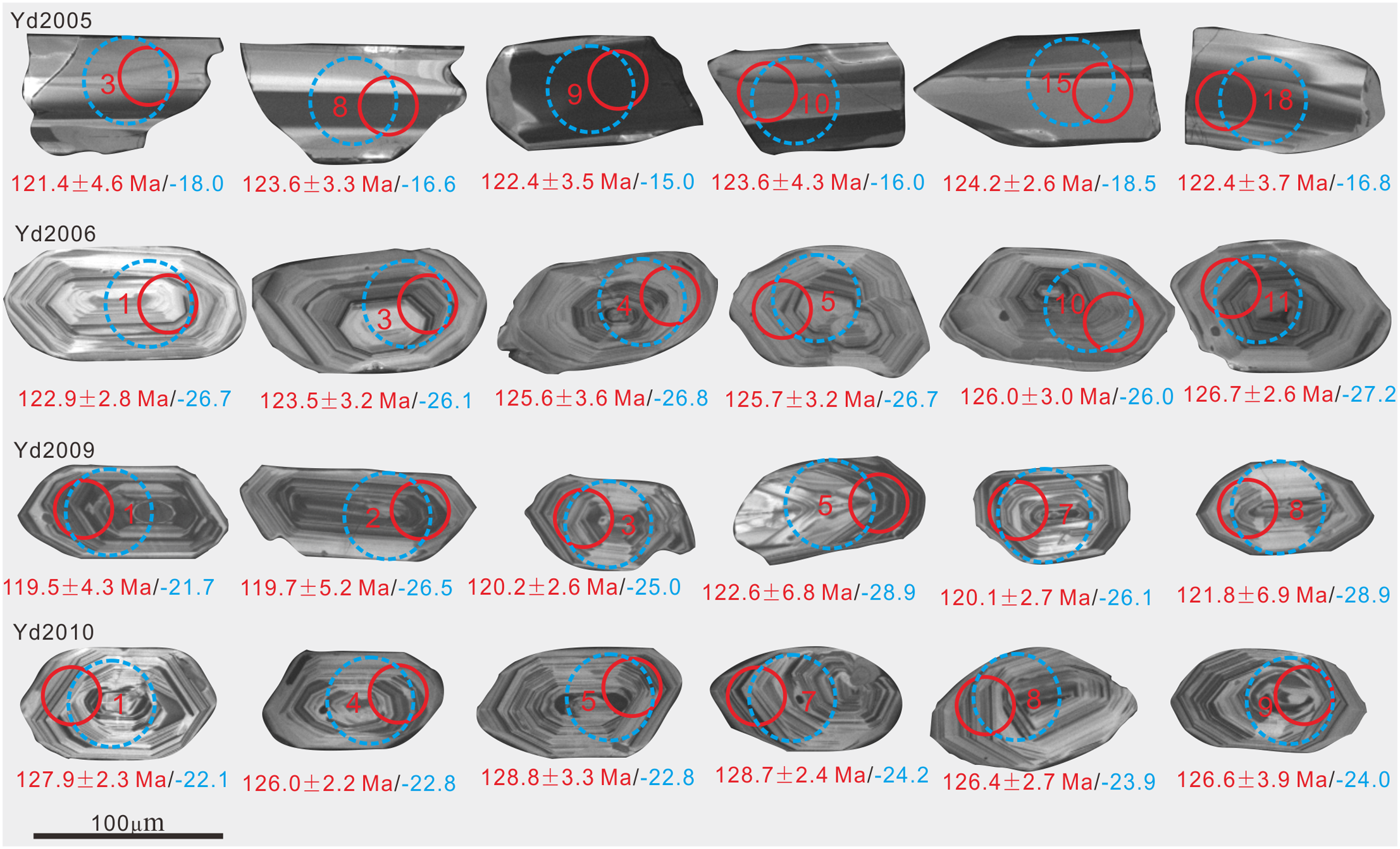
Fig. 4. Representative CL images of zircons from the samples. Solid red circles and blue circles indicate the locations of in situ U–Pb and Hf analyses spot, respectively.

Fig. 5. Zircon U–Pb concordia and weighted mean diagrams of the Pulandian samples.
4.b. Major- and trace-element geochemistry
The major- and trace-element composition results are provided in online Supplementary Table S2. All samples have low loss on ignition (LOI; 0.29–1.11 wt%). Sample Yd2005 has low SiO2 contents (55.93–56.16 wt%), high MgO contents (3.63–4.13 wt%) and high Al2O3 contents (16.78–17.58 wt%), giving Mg no. values of 48.24–50.77. Its K2O and Na2O contents are 2.48–2.58 wt% and 3.55–3.77 wt%, respectively, with low K2O/Na2O ratios (0.69–0.70). The samples demonstrate high-K and subalkaline features (Fig. 6a, b). In contrast, samples Yd2006, Yd2009 and Yd2010 show high SiO2 (63.77–70.80 wt%), high Al2O3 (14.13–17.38 wt%) and low MgO (0.36–1.78 wt%) contents, with Mg no. values of 21.18–46.83. Their K2O and Na2O contents are 2.05–5.37 wt% and 4.08–4.51 wt%, respectively, with variable K2O/Na2O ratios (0.45–1.31). The K2O contents of these Pulandian plutons show positive correlation with the SiO2 contents (Fig. 6b), whereas Fe2O3T, TiO2, P2O5, MgO, CaO and Al2O3 contents display negative correlations (Fig. 7a–f). The granodiorite (samples Yd2006 and Yd2010) shows high-K and subalkaline characteristics, whereas the porphyritic monzogranite (sample Yd2009) demonstrates shoshonitic and alkaline properties (Fig. 6a, b).

Fig. 6. (a) SiO2 and K2O + Na2O correlation (TAS) diagram (after Wilson, Reference Wilson1989); and (b) SiO2 and K2O correlation diagram (after Peccerillo & Taylor, Reference Peccerillo and Taylor1976). Data from Wu et al. (Reference Wu, Lin, Wilde, Sun and Yang2005 a, b); Wang et al. (Reference Wang, Wyman, Xu, Zhao, Li, Wei, Ma and He2007); Yang et al. (Reference Yang, Sun, Chen, Wilde and Wu2007 a, b).

Fig. 7. Harker diagrams display the major-element variations of the Pulandian plutons.
Samples have total rare earth element (REE) contents of 74–190 ppm and show enrichment in low REE (LREE) with high (La/Yb)N ratios (13.5–57.5). They have low Yb contents (0.54–1.24 ppm), low Y contents (4.9–16.4 ppm), high Sr contents (294–711 ppm; with the exception of one sample with 188 ppm content) and Sr/Y ratios of 38.5–108. In the chondrite-normalized REE pattern (Fig. 8), they display no clear Eu anomalies (Eu/Eu* = 0.79–1.21). The primitive-mantle-normalized spidergram (Fig. 8) reveals that these samples are enriched in large-ion lithophile elements (LILEs; e.g. Ba, K, Pb and Sr) and depleted in high-field-strength elements (HFSEs; e.g. Nb, Ta, P and Ti).

Fig. 8. Chondrite-normalized REE and primitive-mantle-normalized trace-element patterns for the samples. Normalized data for normalization and plotting after Sun & McDonough (Reference Sun and McDonough1989). K-rich adakites data from Wang et al. (Reference Wang, Wyman, Xu, Zhao, Li, Wei, Ma and He2007).
4.c. Zircon Hf isotopic compositions
The results of the zircon Hf isotopic analysis are provided in online Supplementary Table S3. Sample Yd2005 yielded relatively uniform 176Hf/177Hf ratios of 0.282275 to 0.282176, with ϵ Hf(t) values of −18.5 to −15.0 and two-stage model ages (T DM2) of 2351–2131 Ma. Sample Yd2006 zircon ϵ Hf(t) values ranged from −27.2 to −25.4, with 176Hf/177Hf ratios of 0.281977–0.281927 and T DM2 ages of 2899–2789 Ma. Sample Yd2009 yielded zircon ϵ Hf(t) values ranging from −28.9 to −21.1, with 176Hf/177Hf ratios of 0.282107–0.281882 and T DM2 ages of 3004–2511 Ma. The ϵ Hf(t) values of sample Yd2010 ranged from −24.6 to −22.1, and its corresponding 176Hf/177Hf ratios and TDM2 ages ranged over 0.282071–0.282000 and 2937–2580 Ma.
5. Discussion
5.a. Rock type and petrogenesis
Although the samples yielded very low LOI (0.29–1.11 wt%), the effect of element migration must be excluded before the geochemical characteristics of the rocks can be interpreted. Studies have shown that the major elements, HFSEs and REEs can remain constant during weak alteration, whereas LILEs can migrate more easily (Alirezaei & Cameron Reference Alirezaei and Cameron2002); LILEs are therefore good indicators to gauge the effect of weak alteration on the samples. Some ratios (e.g. K/Sr and La/Th) can be used to monitor the mobility of the LILEs (Rudnick et al. Reference Rudnick, McLennan and Taylor1985). All sample have low to medium K/Sr ratios (26–233) and low La/Th ratios (3.0–7.1), suggesting that the weak alteration did not modify their element compositions (Xin et al. Reference Xin, Sun, Zhang, Fan and Li2019). The geochemical data of the selected samples, such as major- and trace-element content, therefore reflects their inherited magmatic source characteristics.
All samples in this study are characterized by high Sr contents (294–711 ppm), high Al2O3 contents (14.13–17.58 wt%), high Sr/Y ratios (38.5–108), low Yb contents (0.54–1.24 ppm), low Y contents (4.9–16.4 ppm) and no clear Eu anomalies (Eu/Eu* = 0.79–1.21), displaying typical adakitic geochemical characteristics (Defant & Drummond, Reference Defant and Drummond1990). In addition, most samples fall within the classification of adakite (Fig. 9a, b). These rocks have K2O/Na2O ratios (0.48–1.31) higher than for those of typical adakitic rocks (c. 0.4; Moyen, Reference Moyen2009), which are similar to K-rich adakites from Dabie orogen (Fig. 10a; Wang et al. Reference Wang, Wyman, Xu, Zhao, Li, Wei, Ma and He2007). The trace-element pattern of the rocks is also similar to that for the K-rich adakite rocks (Fig. 8); the samples can therefore be identified as K-rich adakitic rocks.

Fig. 9. (a) Sr/Y versus Y diagram (after Defant et al. Reference Defant, Xu, Kepezhinskas, Wang, Zhang and Xiao2002); and (b) (La/Yb)N versus YbN diagram (after Martin, Reference Martin1999). Data from Zhang & Wan (Reference Zhang and Wang2001) and Xiong et al. (Reference Xiong, Liu and Zhu2011).

Fig. 10. (a) K2O/Na2O versus K2O and (b) La/Yb versus La (after Wang et al. Reference Wang, Wyman, Xu, Zhao, Li, Wei, Ma and He2007).
Adakites are formed during various tectonomagmatic processes, such as partial melting of subducted oceanic slab (Defant & Drummond, Reference Defant and Drummond1990; Wang et al. Reference Wang, Wyman, Xu, Zhao, Li, Wei, Ma and He2007); AFC associated with basaltic magmas (Wareham et al. Reference Wareham, Millar and Vaughan1997; Castillo et al. Reference Castillo, Janney and Solidum1999); magma mixing of basaltic magmas and crustal-derived felsic magmas (Guo et al. Reference Guo, Nakamuru, Fan, Kobayoshi and Li2007; Xu et al. Reference Xu, Ma and Zhang2012); partial melting of thickened lower continental crust (Atherton & Petford, Reference Atherton and Petford1993; Petford & Atherton, Reference Petford and Atherton1996; Wang et al. Reference Wang, McDermott, Xu, Bellon and Zhu2005); and partial melting of delaminated lower continental crust (Gao et al. Reference Gao, Rudnick, Yuan, Liu, Liu, Xu, Ling, Ayers, Wang and Wang2004; Hou et al. Reference Hou, Gao, Qu, Rui and Mo2004).
Adakitic rocks were originally defined as the partial melting of subducted young, hot slabs (Defant & Drummond, Reference Defant and Drummond1990). As the slab melt rises, it is metasomatized by mantle-derived material, resulting in rocks characterized by low K2O/Na2O ratios (c. 0.5) and high MgO (c. 4–9 wt%), Mg no. (> 50), Ni and Cr contents (Stern & Kilian, Reference Stern and Kilian1996). The Pulandian adakitic rocks have high K2O/Na2O ratios (mean 0.85) and low MgO content (0.36–4.13 wt%), Mg no. (mean 37.7), Ni content (mean 20 ppm) and Cr content (mean 44 ppm), which are inconsistent with the features of melt of subducted oceanic slab. The samples have low Ce/Pb ratios (mean 7.6) and obvious negative Nb and Ta anomalies (Fig. 7), indicating that they may have originated in continental crustal source rather than partial melting or AFC of oceanic basalts (Ce/Pb > 20) (Rudnick & Fountain, Reference Rudnick and Fountain1995; Plank, Reference Plank2005). Moreover, no clear Eu anomalies in all the samples imply that fractional crystallization of plagioclase did not play a crucial role in the process of their formation (Wang et al. Reference Wang, Wyman, Xu, Zhao, Li, Wei, Ma and He2007). The lack of obvious indications of fractional crystallization (Fig. 10b) and of large-scale mafic rocks around Pulandian area (Fig. 2) also support the fact that these rocks were not formed from AFC of basalt. The significant negative ϵ Hf(t) values (−33.3 to −15.0) indicate that they derived from partial melting of the ancient crust rather than magmatic mixing (Fig. 11a). The geochemical characteristics of the samples are similar and there are no mafic microgranular enclaves in the rocks (Figs 3, 6, 8); magma mixing therefore does not explain their origin. The melt formed by partial melting of the delaminated lower crust may have been upwelling, resulting in interaction with the mantle and the production of geochemical features similar to those of the melting of the subducted oceanic slab (Xu et al. Reference Xu, Ma and Zhang2012); the rocks therefore did not originate from the partial melting of the delaminated lower crust. Experimental petrology shows that adakitic magmas can be formed by partial melting of the thickened lower continental crust (Petford & Atherton, Reference Petford and Atherton1996; Xiong et al. Reference Xiong, Adam and Green2005). The melts from the above model are characterized by low MgO (< 2 wt%) and Mg no. (< 50), and high SiO2 (> 60 wt%) and K2O (> 2 wt%) contents, which are matched by the features of all samples (see online Supplementary Table S2). As shown in TiO2 versus SiO2 and MgO versus SiO2 diagrams (Fig. 12), the majority of the samples from Pulandian plot within the field of thickened lower-crust-derived adakitic rocks.

Fig. 11. (a) ϵ Hf(t) versus age (Ma) and (b) age histogram of Mesozoic magmatic rocks of NCC. Data from Yang et al. (Reference Yang, Wu, Zhang, Zhang and Wilde2004, Reference Yang, Sun, Chen, Wilde and Wu2007 a, b).

Fig. 12. (a) TiO2 versus SiO2 and (b) MgO versus SiO2 diagrams for samples. The field of subducted oceanic crust-derived adakites are after Wang et al. (Reference Wang, Wyman, Xu, Zhao, Li, Wei, Ma and He2007). The field of thickened lower crust-derived adakites are after Atherton & Petford (Reference Atherton and Petford1993) and Muir et al. (Reference Muir, Weaver, Bradshaw, Eby and Evans1995), and the field of delaminated lower crust-derived adakities are after Wang et al. (Reference Wang, Xu, Zhao, Bao, Xu and Xiong2004 a, b, Reference Wang, Wyman, Xu, Zhao, Li, Wei, Ma and He2007).
Previous studies shown that while partial melting if the garnet is remained as residue, the partial melt result HREE depletion (Othman et al. Reference Othman, White and Patchett1989; Wang et al. Reference Wang, Wyman, Xu, Zhao, Li, Wei, Ma and He2007). Plagioclase has a large partition coefficient for Sr and Eu elements, and its crystallization can result in Sr and Eu negative anomalies (Nash & Crecraft, Reference Nash and Crecraft1985). On the basis of the geochemical features (online Supplementary Table S2; Fig. 8), the residues are rich in garnet and poor in plagioclase. According to partial melting experimental research, garnet-absent and plagioclase-rich samples indicate formation pressures lower than 10 kbar, both garnet and plagioclase are present at formation pressures of 12.5–15 kbar, and garnet-rich and plagioclase-absent samples indicate formation pressures higher than 15 kbar (Skjerlie & Johnston, Reference Skjerlie and Johnston1993; Sen & Dunn, Reference Sen and Dunn1994; Patiño Douce & Beard, Reference Patiño Douce and Beard1995; Litvinovsky et al. Reference Litvinovsky, Steele and Wickham2000). The above-mentioned residues can therefore only form in a high-pressure environment, in accordance with the pressure of thickened lower continental crust. The source and petrogenesis of the Pulandian plutons can be further illustrated with diagrams discerning the source nature of adakites (Foley et al. Reference Foley, Tiepolo and Vannucci2002). In the K2O versus K2O/Na2O diagram (Fig. 10a), samples fall within the metabasaltic and eclogite melt fields, similar to the adakitic granites in the Dabie Orogen. In the (La/Yb)N versus YbN diagram (Fig. 9b), all plots are mainly close to the melt of eclogite. We therefore suggest that the Pulandian adakitic rocks were formed by partial melting of thickened eclogitic lower crust.
5.b. Tectonic setting and geodynamic mechanism
Magmatic and volcanic activities were strong in the Liaoning Peninsula during the Early Cretaceous Epoch, which resulted in the formation of numerous intrusive rocks (Fig. 1b). The lithology of magmatic rocks in this period is mainly monzogranite, granodiorite and alkaline granite (Yang et al. Reference Yang, Wu, Zhang, Zhang and Wilde2004; Liu et al. Reference Liu, Li, Zhu and Zhao2019). According to the results of previous research, some Early Cretaceous A-type granites and alkaline rocks have been reported in the Liaoning Peninsula – for example, Qianshan granite (127 Ma), Taipingshan alkaline feldspar granite (129 Ma), Guanmenshan Granite porphyry (126 Ma) and quartz syenite (127 Ma) – which indicate that the Liaodong Peninsula existed in an extensional tectonic setting (Yang et al. Reference Yang, Sun, Chen, Wilde and Wu2007 a, b; Liu et al. Reference Liu, Guo and Zhu2016, Reference Liu, Li, Zhu and Zhao2019). Metamorphic core complexes (MCC) are also widely developed in the Liaoning Peninsula; for example, the Yinmawan (129–120 Ma) and Gudaoling (127–118 Ma) plutons were emplaced along the detachment fault of the Liaonan–Wanfu MCC (130–113 Ma), which was in an extensional tectonic environment (Guan et al. Reference Guan, Liu, Ji, Zhao, Hu and Gregory2008; Ji, Reference Ji2010). Zhu & Xu (Reference Zhu and Xu2019) reported that the slab rollback of the Palaeo-Pacific Ocean began at c. 145 Ma, which may have resulted in the extension of the NCC. We therefore believe that the Early Cretaceous (130–120 Ma) extension was closely related to the slab rollback of the Palaeo-Pacific Ocean. The age of the Pulandian plutons is 127–120 Ma, which is nearly the same as the above pluton ages. The Pulandian plutons show LILE-enriched and HFSE-depleted characteristics, which indicate that these rocks were formed in a subduction environment. In the tectonic diagrams (Fig. 13), all samples fall within the area of arc-related environment. Combined with age and features, we believe that they were formed in an extensional setting involved in slab rollback of the Palaeo-Pacific Ocean.

Fig. 13. (a) Y versus Nb and (b) (Y + Nb) versus Rb (Pearce et al. Reference Pearce, Harris and Tindle1984) for Pulandian sample rocks. VAG – volcanic-arc granites; syn-COLG – syn-collisional granites; ORG – orogenic ridge granites; WPG – within-plate granites.
Previous studies on mantle xenoliths indicate that the thickness of the NCC was 200 km during the Palaeozoic Era and 80–100 km during the Cenozoic Era, suggesting that significant destruction and lithospheric thinning occurred (Fan et al. Reference Fan, Zhang, Baker, Jarvis, Mason and Menzies2000; Wu et al. Reference Wu, Yang, Xu, Wilde and Walker2019). The constructed seismic data of the NCC also support dramatic thinning (Zhu et al. Reference Zhu, Chen, Wu and Liu2011). In recent years, there has been more progress in determining the dynamic setting of lithospheric thinning of NCC (Wu et al. Reference Wu, Lin, Wilde, Sun and Yang2005 a; Zheng et al. Reference Zheng, Griffin, O’Reilly, Yu, Zhang, Pearson and Zhang2007, Reference Zheng, Xu, Zhao and Dai2018; Zhu et al. Reference Zhu, Yang and Wu2012; Niu et al. Reference Niu, Liu, Xue, Shao, Chen, Duan, Guo, Gong, Hu, Hu, Kong, Li, Liu, Sun, Sun, Ye, Xiao and Zhang2015). However, the geodynamic mechanism remains controversial and researchers are focused on two separate models: (1) rapid lithospheric delamination (e.g. Gao et al. Reference Gao, Rudnick, Yuan, Liu, Liu, Xu, Ling, Ayers, Wang and Wang2004; Wu et al. Reference Wu, Yang, Wilde and Zhang2005 b, Reference Wu, Xu, Gao and Zheng2008); and (2) thermo-mechanical-chemical erosion (e.g. Griffin et al. Reference Griffin, Zhang, O’Reilly and Ryan1998; Zheng et al. Reference Zheng, Griffin, O’Reilly, Yu, Zhang, Pearson and Zhang2007). Frequent magmatic activity was one of the important forms of destruction of NCC; the Liaodong Peninsula is the strongest and most typical area of destruction (Liu et al. Reference Liu, Ji, Shen, Guang and Davis2011). A fuller understanding of the magmatic rocks of the Liaodong Peninsula will therefore yield knowledge of the lithospheric thinning and destruction of NCC.
During the Jurassic Period, the intrusive rocks in Liaodong Peninsula were dominated by quartz diorite, granodiorite and monzogranite, etc., most of which belong to high-K calc-alkaline granite (Fig. 6b; Yang et al. Reference Yang, Wu, Zhang, Zhang and Wilde2004, Reference Yang, Sun, Chen, Wilde and Wu2007 a, b; Wu et al. Reference Wu, Yang, Wilde and Zhang2005 b; Sun et al. Reference Sun, Suzuki, Wu and Lu2005). There were also many Jurassic adakities (Fig. 9), derived from the partial melting of the thickened lower crust (Gao et al. Reference Gao, Rudnick, Yuan, Liu, Liu, Xu, Ling, Ayers, Wang and Wang2004; Wu et al. Reference Wu, Yang, Wilde and Zhang2005 b; Zhu et al. Reference Zhu, Yang and Wu2012). In addition, the negative Lu–Hf isotopes also supported the theory that Jurassic granites are mainly derived from the partial melting of ancient thickened lower crust (Fig. 11a). The existence of thickened lower crust is the premise of lithospheric delamination. The rock types of Early Cretaceous intrusions in Liaodong Peninsula are complex, mainly composed of I-type granite, A-type granite, alkaline and mafic rock (Fig. 6; Sun & Yang, Reference Sun and Yang2009; Lan et al. Reference Lan, Fan, Hu, Tomkins, Yang and Liu2011). According to previous studies on geochemistry and Lu–Hf isotopes (−30 to +5; Fig. 11a), rocks from this period may have originated from different magma sources such as depleted mantle, enriched lithospheric mantle or ancient lower crust (Wu et al. Reference Wu, Yang, Xu, Wilde and Walker2019), possibly the result of intensive crust–mantle interaction caused by asthenosphere upwelling after lithospheric delamination of the NCC. The wide distribution of magmatic rocks (Fig. 11b) and extensional tectonics over a short period (c. 130–120 Ma) in the Liaodong Peninsula (Liu et al. Reference Liu, Davis, Zhiyong and Wu2005) is consistent with the rapid lithospheric delamination characteristics. We therefore suggest that the lithospheric delamination model is a better geodynamic mechanism for the thinning and destruction of the NCC than thermo-mechanical-chemical erosion.
Several possible trigger factors for lithospheric delamination have recently been proposed, including: (1) thickened lower crust (Gao et al. Reference Gao, Rudnick, Yuan, Liu, Liu, Xu, Ling, Ayers, Wang and Wang2004, Reference Gao, Zhang, Xu and Liu2009); (2) hydrous fluids or melts related to Palaeo-Pacific subduction (Gao et al. Reference Gao, Zhang, Xu and Liu2009; Zhu et al. Reference Zhu, Chen, Wu and Liu2011; Zhu & Xu, Reference Zhu and Xu2019); and (3) upwelling asthenosphere (Deng et al. Reference Deng, Su, Niu, Liu, Zhao, Zhao, Zhou and Wu2007; Liu et al. Reference Liu, Wei, Zhang, Chen and Zhang2020). First, thickened lower crust is a crucial pre-condition to lithosphere delamination. The thickened lower crust undergoes a phase transformation of the eclogite, which makes it denser than the lithospheric mantle (Kay & Kay, Reference Kay and Kay1993). The Jurassic subduction of the Palaeo-Pacific Ocean caused the crust of the NCC to thicken (Fig. 14a; Wu et al. Reference Wu, Yang, Wilde and Zhang2005 b; Zhu & Xu, Reference Zhu and Xu2019). The Jurassic and Early Cretaceous adakites in the Liaodong Peninsula provide evidence for the thickened crust of the NCC (Yang et al. Reference Yang, Wu, Zhang, Zhang and Wilde2004; Wu et al. Reference Wu, Yang, Wilde and Zhang2005 b; Zhang et al. Reference Zhang, Zhao, Zheng and Dai2010; this study). The thickened crust (eclogitic layer) could not directly sink because of the refractory and buoyant nature of the NCC lithospheric mantle (Deng et al. Reference Deng, Su, Niu, Liu, Zhao, Zhao, Zhou and Wu2007). Secondly, the lithospheric mantle was metasomatized by hydrous fluids or melt produced by the subduction of the Palaeo-Pacific subduction (Niu et al. Reference Niu, Liu, Xue, Shao, Chen, Duan, Guo, Gong, Hu, Hu, Kong, Li, Liu, Sun, Sun, Ye, Xiao and Zhang2015). At c. 145 Ma the Palaeo-Pacific Ocean started to rollback, resulting in asthenosphere upwelling (Fig. 14b; Zheng et al. Reference Zheng, Xu, Zhao and Dai2018; Zhu & Xu, Reference Zhu and Xu2019). At c. 130–120 Ma, the Palaeo-Pacific Ocean reached its maximum rate of subduction slab rollback, transitioned into a high-angle subduction and produced a stagnation slab in the mantle zone (Fig. 14c; Zhu & Xu, Reference Zhu and Xu2019). Because of the slower slab rollback and weak asthenosphere upwelling at 145 Ma, we believe that the refractory and thick lithospheric mantle of the NCC experienced a small-scale partial melting (Zheng et al. Reference Zheng, Chen, Xu and Zhang2016, Reference Zheng, Xu, Zhao and Dai2018). At c. 130–120 Ma, the stagnation slab began to dehydrate, changing the physical properties of the overlying lithospheric mantle (e.g. decreasing its viscosity) and causing its stability to be disrupted (Zhu et al. Reference Zhu, Chen, Wu and Liu2011). Under the combined action of these processes, the lithosphere of the NCC underwent delamination. This resulted in a rapid upwelling of large amounts of asthenosphere, providing more heat and accelerating partial melting of the lithospheric mantle. During this period, a large number of rocks of different sources and properties were generated (e.g. I-/A-type granite, adakites and rock; Yang et al. Reference Yang, Wu, Zhang, Zhang and Wilde2004; Wu et al. Reference Wu, Yang, Wilde and Zhang2005 b; this study). Meanwhile, the widespread extensional tectonics and metamorphic core complexes appear during this stage.
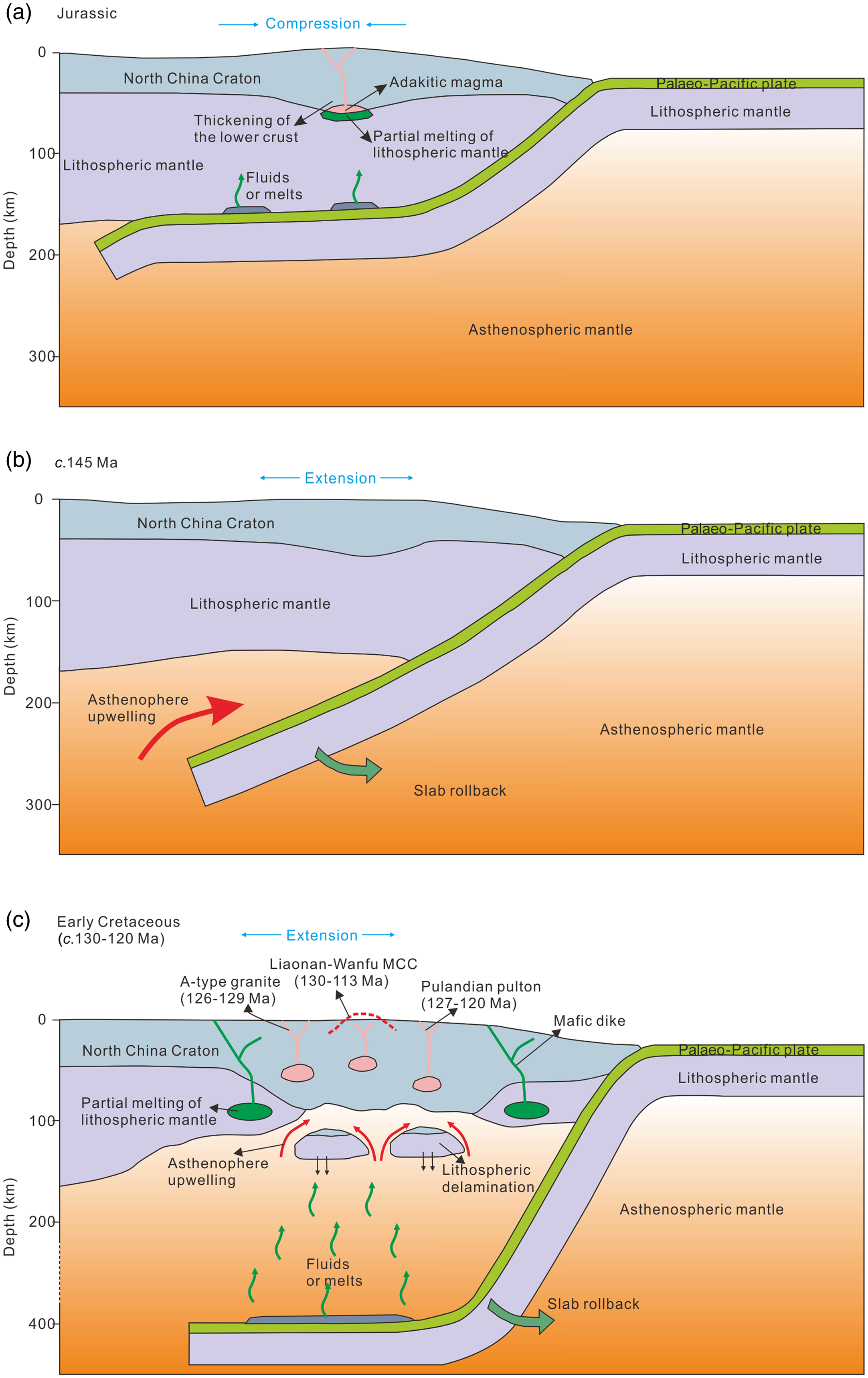
Fig. 14. Lithospheric evolution process of the NCC during the Mesozoic Era. (a) Jurassic: the Palaeo-Pacific Ocean subducted, causing the NCC crust to thicken (Wu et al. Reference Wu, Yang, Wilde and Zhang2005 b). (b) c. 145 Ma: the subducting Palaeo-Pacific slab began to rollback with the asthenosphere upwelling (Zhu & Xu, Reference Zhu and Xu2019). (C) Early Cretaceous (c. 130–120 Ma): the Palaeo-Pacific Ocean reached its maximum rate of subduction slab rollback, transitioned into a high-angle subduction and produced a stagnation slab in the mantle zone. The stagnation slab began to dehydrate, changing the physical properties of the overlying lithospheric mantle, which caused its stability to be disrupted and its subsequent delamination. During the period, widespread extensional tectonics occurred in the shallow crust of the NCC. The Liaonan–Wanfu MCC and rocks of different types and properties were generated (e.g. I-/A-type granite, adakites rock; Yang et al. Reference Yang, Wu, Zhang, Zhang and Wilde2004; Wu et al. Reference Wu, Yang, Wilde and Zhang2005 b; this study). MCC – metamorphic core complexes.
6. Conclusions
-
(1) The Pulandian monzodiorite, granodiorite and porphyritic monzogranite are adakitic rocks that were emplaced at 127–120 Ma (i.e. Early Cretaceous).
-
(2) The Pulandian adakitic rocks were formed by partial melting of thickened eclogitic lower crust, and in an extensional setting related to slab rollback of the Palaeo-Pacific Ocean.
-
(3) As a result of the rapid slab rollback of the Palaeo-Pacific Ocean during c. 130–120 Ma, the asthenosphere upwelled and modified the thickened lithospheric mantle, which lost its stability, eventually resulting in the lithospheric delamination and thinning of the NCC.
Supplementary material
To view supplementary material for this article, please visit https://doi.org/10.1017/S0016756822000644
Acknowledgements
This research was funded by the National Key R&D Program of China (grant no. 2018YFC0603804), the National Natural Science Foundation of China (grant no. 41402060), Science and Technology Project of Department of Education, Jilin Province (grant no. JJKH20200946KJ), the Natural Science Foundation of Jilin Province (grant no. 20170101201JC) and the Self-Determined Foundation of Key Laboratory of Mineral Resources Evaluation in Northeast Asia, Ministry of Natural Resources (grant nos DBY-ZZ-19-13 and DBY-ZZ-19-15), and supported by the Graduate Innovation Fund of Jilin University (grant no. 101832020CX211).
Conflict of interest
None.