Introduction
Trichinellosis is a widespread meat-borne zoonotic disease caused by the nematode genus Trichinella. It affects a wide range of animal species and humans (Pozio Reference Pozio2015). FAO/WHO (2013) listed trichinellosis as the seventh most important foodborne parasitic disease in the world. It has a global fundamental influence on socio-economic consequences such as the international trade of animals and as a public health problem (Murrell Reference Murrell2016). Trichinellosis in man is caused by the pathogenic species Trichinella spiralis (T. spiralis) (Appleton et al. Reference Appleton, Blum, Gebreselassie and Lamb2012). The adult worms of T. spiralis reside in the mucosa of the small intestine of mammals, birds, and reptiles. However, the larvae are found inside skeletal muscles. Humans are infected via consumption of infected raw or inadequately cooked pork meat containing T. spiralis larvae (Pozio Reference Pozio2007). The clinical manifestation of trichinellosis exists in two phases: the intestinal phase, characterised by abdominal pain, nausea, vomiting, and diarrhea, and the muscle phase, resulting from the inflammatory response caused by muscle cell invasion by the larvae, which may be accompanied by fever, myalgia, eosinophilia, and palpebral or facial edema (Dvorožnáková et al. Reference Dvorožnáková, Kolodziej-Sobocinska and Hurníková2012). Early clinical diagnosis of trichinellosis is quite challenging due to non-specific symptoms and signs. Likewise, chronic forms of the disease are difficult to diagnose as it largely depends on the history of ingestion of raw or inadequately cooked meat and detection of Trichinella larvae in the muscle (Gomaa Reference Gomaa2020).
Currently, human trichinellosis can be diagnosed by identification of larvae in a muscle biopsy or the serological detection of specific anti-Trichinella IgG antibodies in serum (Sun et al. Reference Sun, Song, Jiang, Ren, Yan, Han, Liu, Zhang, Wang and Ciu2018). Nevertheless, microscopic examination of muscle biopsy specimens is not sensitive during the early stage of the disease or in cases of light infection, in addition to being unable to distinguish species. Furthermore, highly invasive tissue sampling may result in medical complications and undermine the utility of these procedures (Riddle et al. Reference Riddle, DuPont and Connor2016).
Enzyme-linked immunosorbent assay (ELISA) and Western blotting are frequently used tests for serological diagnosis of human trichinellosis. Detection of anti-Trichinella IgG is achieved by using excretory/secretory antigens of T. spiralis muscle larvae. (Gamble et al. Reference Gamble, Pozio, Bruschi, Nöckler, Kapel and Gajadhar2004). However, the main drawback of ELISA is false negative results in the early stage of infection (Wang et al. Reference Wang, Shi, Liu, Jiang, Guan, Chen and Cui2017a). Previous studies have observed that 100% detection of IgG antibodies may not be reached until at least 1–3 months after human infection (Gamble et al. Reference Gamble, Pozio, Bruschi, Nöckler, Kapel and Gajadhar2004). Furthermore, it is difficult to distinguish between recent and past infection as both human and animal hosts develop a detectable antibody response lasts for a long time after the acute phase of the disease (Yang et al. Reference Yang, Cai, Tong, Sun, Xuan, Kang, Vallée, Boireau, Cheng and Liu2016).
Nano-ELISA is a sensitive protein detection procedure based upon the use of nanoparticles and ELISA. Nanotechnology improves identification of microorganisms (Wang et al. Reference Wang, Hu, Jin, Yao and Li2006). Combination of nano technique with traditional ELISA can improve its detection limit depending on the unique physical and chemical properties of metal and metal oxide nanoparticles (Jia et al. Reference Jia, Zhong, Hua, Liu, Jing, Lou, Yao, Xiang, Jin and Zhao2009). Nanoparticles are transporters that load enzymes and antibodies for signal amplification, have enzyme-mimicking activity that replaces the natural enzyme labels, and act as signal transducers to deliver fluorescence or luminescence signals as an alternate output (Gao et al. Reference Gao, Zhou and Chandrawati2019). Promising outcomes from using nano assays in the diagnosis of cancer (Jia et al. Reference Jia, Zhong, Hua, Liu, Jing, Lou, Yao, Xiang, Jin and Zhao2009) and infectious diseases (Guirgis et al. Reference Guirgis, Sáe-Cunha, Gomes, Cavadas, Silva, Doria, Blatch, Baptista, Pereira, Azzazy, Mota, Prudêncio and Franco2012; Kamel et al. Reference Kamel, El-Baz, Demerdash, El-Karaksy, El-Gendy, Hassan, Salah, El-Moneem, Hendawy and Aly2016; Khodadadi et al. Reference Khodadadi, Madani, Hoghooghi Rad and Atyabi2021) have offered the challenge to employ it in the diagnosis of the experimental trichinellosis.
The use of DNA/RNA-based methods derives from the fact that each species of parasite carries unique DNA or RNA sequences that differentiate it from other parasites (Verma et al. Reference Verma, Das, Manjunathachar and Muwel2018). The advancement of molecular tools has resulted in great improvement in the diagnosis of infectious diseases (Bauer et al. Reference Bauer, Perez, Forrest and Goff2014). Polymerase Chain Reaction (PCR) is a highly sensitive method in the detection of Trichinella in faeces, blood, and meat (Liu et al. Reference Liu, Ren, Shi, Hu, Song, Duan, Zhang, Du, Liu, Jiang, Wang and Cui2017; Ashour et al. Reference Ashour, Aboul Assad, El-Kowrany and Abdel Ghaffar2018; Ojodale et al. Reference Ojodale, Inabo, Ella and Okubanjo2021). However, real-time PCR outperforms conventional PCR in speed, simplicity, reproducibility, and quantitative capability (Yang & Rothman Reference Yang and Rothman2004). Using fluorescent DNA intercalating dyes, such as SYBR-Green I in real-time PCR, affords considerable cost-benefit (Tajadini et al. Reference Tajadini, Panjehpour and Javanmard2014). Rep primer is a nuclear 1.6 kb repetitive element from T. spiralis DNA sequences. It can detect newborn larvae (NBL) early in mice (from 5 days post-infection, or dpi) and last for a long time (up to 19 dpi). Therefore, it could be a useful tool in early diagnosis when antibodies are not detectable (Krivokapich et al. Reference Krivokapich, Prous, Gatti and Arbusti2019). The aim of this study was to compare parasitological, serological, and molecular diagnostic methods in the early detection of trichinellosis in both its mild and severe states of infection and to detect the sensitivity of Nano-based ELISA versus real time PCR in the diagnosis of T. spiralis infection.
Material and methods
Parasite and animals
Larvae of T. spiralis were obtained from infected pig muscles from the Cairo Slaughterhouse, Cairo, Egypt and maintained at the Theodor Bilharz Research Institute, Giza, Egypt by serial passage in rats in accordance with the method described by Mayer-Scholl et al. (Reference Mayer-Scholl, Pozio, Gayda, Thaben, Bahn and Nöckler2017). Ninety-nine laboratory bred, Swiss albino male mice, about 5–6 weeks of age and weighing 20–25g, were supplied from theTheodor Bilharz Research Institute animal house and maintained in accordance with national and institutional guidelines.
Experimental study design
Animals were divided into two main groups; a control group (9 mice; Group I, normal non-infected) and experimental groups (90 mice; Groups 2 and 3). Mice were orally infected by gavage with 100 muscle larvae/mouse in Group 2 and 300 muscle larvae/mouse in Group 3 (Sun et al. Reference Sun, Wang, Liu, Jiang and Liu2015a, Reference Sun, Liu, Wang, Jiang, Wang, Liu, Liu, Zhang and Cuib). Each experimental group was then subdivided into 5 subgroups (A, B, C, D, E; 9 mice each) according to the time of sacrifice; 4, 6, 8, 10, and 15 dpi. At scarification time, the small intestine was removed, longitudinally opened, and washed for counting adult worms. Blood samples were collected, centrifuged at 1000 g for 20 min to separate serum. The collected serum was stored at -20°C until tested for T. spiralis circulating antigen by traditional and Nano-based ELISA. Blood samples were also tested for T. spiralis by SYBR Green real-time PCR using Rep primer.
Parasitological assessment
Isolation and adult worm counting
Infected mice were euthanized, and the abdominal cavity was dissected. The small intestine was taken off, washed, opened longitudinally along its whole length then cut into small pieces (about 2 cm) and kept in normal saline for 3–4 h at 37°C. The intestinal specimens were shaken well in saline, rinsed, and finally removed. Adults were allowed to sediment for 0.5 h, then the supernatant fluid was discarded, and the remaining fluid was poured into a petri dish with parallel lines drawn at its base using a permanent ink marker. Adults were counted under the dissecting microscope at 10X power (Wakelin & Lioyed Reference Wakelin and Lloyd1976).
Verification of T. spiralis infection
Two mice from each subgroup were maintained and sacrificed at 28 dpi. To verify infection, a piece of diaphragm was taken and microscopically examined to detect T. spiralis muscle larvae (Chu et al. Reference Chu, Kim, Lee, Lee, Kim and Quan2016).
T. spiralis adult worm-crude extract-antigen (CEA) preparation
Thirty mice were orally inoculated with 5000 T. spiralis muscle larvae/mouse. At 5 dpi, mice were euthanized, and the small intestine was collected, opened, washed in prewarmed phosphate-buffered saline (PBS, pH 7.2), cut into small pieces, and incubated in PBS for 1.5 h at 37°C on a 300 μm sieve. The intestinal debris was filtered through a 200 μm sieve, and the released worms were allowed to settle for 30 min then centrifuged at 600 g for 10 min after multiple washes in PBS supplemented with 100 units of penicillin/mL and 100 μg streptomycin/mL then collected in a clean tube (Zocevic et al. Reference Zocevic, Vallee, Blaga, Liu, Lacour and Boireau2011). To prepare the crude antigen, the collected worms were suspended in deionized water, exposed to 5 freeze-thaw cycles, then homogenized on ice in a glass tissue grinder. The remaining fragments were subjected to an additional round of ultrasonication (99 times 3-s cycle on ice) for further homogenization (Cui et al. Reference Cui, Liu, Wang, Zhang, Jiang, Liu and Wang2013). The homogenate was centrifuged at 15,000 g for 1 h at 4°C, then the supernatant was collected and stored at -20°C to be used as T. spiralis crude extract antigen (Yang et al. Reference Yang, Li, Liu, Sun, Liu, Zhang, Jiang, Zhang, Ren, Wang and Cui2015). The amount of protein content in prepared antigen was measured by mass spectrometry (Gillette & Carr Reference Gillette and Carr2013).
Control serum
The normal mice serum was diluted to be used as a negative control. Regarding positive control, the prepared T. spiralis crude extract antigen was added to the normal mice serum.
Polyclonal antibodies against T. spiralis adult crude extract antigen preparation
New Zealand white rabbits, 8 months old and weighing about 1.5 kg, were purchased from Rabbit Research Unit (RRU), Faculty of Pharmacy, Cairo University, Egypt. Each rabbit was immunized intramuscularly at four sites with a priming dose of 1 mg of prepared T. spiralis crude extract antigen mixed with 50 μl of aluminium hydroxide (13 mg/mL). After 15 d, rabbits were injected with the first booster dose (0.5 mg antigen mixed with 50 μl of aluminium hydroxide) followed by three booster doses at one-week intervals (Gillette & Carr Reference Gillette and Carr2013). Blood samples were taken from rabbits before the priming dose and examined with ELISA for cross-reaction with other parasites to ensure that the rabbits were parasite-free. Samples were also examined at each booster to detect the titre of anti-T. spiralis antibodies (Guobadia & Fagbemi Reference Guobadia and Fagbemi1997). When the titre was high, blood samples were collected, and anti-serum was stored at -70°C until used. Polyclonal IgG against T. spiralis crude extract antigen was purified from collected serum by Ammonium sulfate precipitation (Kent Reference Kent and Javois1999) and caprylic acid (Mckinney & Parkinson Reference McKinney and Parkinson1987).
Labeling of anti-T. spiralis IgG antibody with horseradish peroxidase (HRP) (periodate method)
As demonstrated by Tijssen & Kurstak (Reference Tijssen and Kurstak1984), 5 mg of HRP (Sigma Aldrich, St. Louis, MO, USA) was resuspended in 1.2 mL of distilled water, after addition of 0.3 mL of freshly prepared sodium periodate then kept for 20 min at room temperature. The mixture was dialyzed against 1 mm sodium acetate buffer pH 4 at 4°C with multiple changes overnight. After removal from the dialysis tube, 0.5 mL of antibody solution (polyclonal solution of 5 mg/mL in 0.02 M carbonate buffer, pH 9.6) was added. After the mixture was incubated for 2 h at room temperature, 100 μl of sodium borohydride was added, then the solution was re-incubated for 2 h at 4°C. The HRP-antibody conjugate was dialysed with several changes against 0.01M PBS, pH 7.2.
Synthesis of silver nanoparticles (AgNPs)
In brief, an Erlenmeyer flask containing 30 mL of 0.002M sodium borohydride (NaBH4) was placed in an ice bath and stirred on a stir plate for 20 min. After stirring, 2 mL of 0.001M silver nitrate (AgNO3) was dripped into the NaBH4 solution (about 1 drop/sec) followed by a few drops of 1.5M sodium chloride (NaCl) solution. Change in color of the mixture from a transparent colorless solution to a distinctively pale yellow and black color is indicative of the formation of silver nanoparticles. To prevent aggregation, 0.3% polyvinyl pyrrolidone (PVP) was added, then the mixture was decanted into a mold to leave air bubbles and unmelted PVP particles in the beaker. The solution was then centrifuged at 20,000g 14°C for 30 min to separate silver nanoparticles and freeze-dried nanoparticles kept at 5 ± 3°C. The weights of freeze-dried nanoparticles were measured (Wang et al. Reference Wang, Qiao, Chen and Ding2005).
Silver nano probes (Ag nano probes) preparation
AgNPs were coupled with anti-rabbit polyclonal IgG antibodies and HRP. AgNPs-HRP-PAb 600 ug of the prepared anti-T. spiralis antigen IgG-PAb or (HRP polyclonal antibody, HRP-PAb) (30 ug/mL, in phosphate-buffer, pH 7.5) were added to 20 mL pH adjusted AgNPs solution and mixed gently for 3 h. After mixing, 4 mL of 10 % bovine serum albumin (BSA) solution was added and the mixture was then centrifuged three times at 13,000 rpm for 45 min at 4oC after 20 min incubation period at room temperature. The pellets were re-suspended in 2 mL phosphate buffer after the last centrifugation (pH 7.2, 0.01M containing 1% BSA and 0.05% sodium azide) and then stored at 4oC before being used (Ambrosi et al. Reference Ambrosi, Castaneda, Killard, Smyth, Alegret and Merkoçi2007).
Characterization of AgNPs and Ag nano probes
Briefly, the silver nanoparticle powder in the freeze-drying cuvette was re-dissolved in deionized water and the suspension then homogenized using a Fisher Bioblock Scientific ultrasonic cleaning container (Surrey, UK). The protein content (free) in the supernatant was measured using the Bradford protein assay spectrophotometric method at 595 nm after the supernatant was collected. To determine the encapsulation efficiency (AE) and loading capacity (LC) of nanoparticles, the following formulas were used: A represents the total amount of Ab, B represents the free amount of Ab, and C represents the weight of nanoparticles.


The scanning electron microscopy (HR-TEM) images were performed at Nanotech Company (Giza, Egypt) for photo-electronic purposes. The HR-TEM used JOEL JEM-2100 operating at 200 kilovolts (kV) equipped with a Gatan Erlangshen ES500 digital camera (Pleasanton, CA) (Brice et al. Reference Brice-Profeta, Arrio, Tronc, Menguy, Letard, Cartier dit Moulin, Noguès, Chanéac, Jolivet and Sainctavit2005). Samples of AgNPs were produced by putting one drop of a dilute suspension of AgNPs in water on a carbon-coated copper grid for 3 min at room temperature (Figure 1).

Figure 1. (a) SEM silver nanoparticles 50 nm size; (b) Size distribution analysis.
Standard ELISA
The purified polyclonal T. spiralis IgG worked as capture and detector antibodies whereas polyclonal T. spiralis IgG-HRP conjugate served as secondary antibodies. The selected concentrations and dilutions were as follows: 1/10 dilution of negative control and positive control serum, 10 μg/mL polyclonal T. spiralis IgG, and 1/5000 dilution of anti-rabbit IgG-HRP conjugate.
All wells of polystyrene microplate were coated with polyclonal T. spiralis IgG, then blocked with PBS pH 7.2 containing 1% foetal calf serum and 0.05% Tween® 20 (Sigma-Aldrich P7949). To each well, tested serum samples, negative and positive control serum samples were separately added. After that, polyclonal IgG against T. spiralis CEA, antirabbit polyclonal IgG-HRP conjugate, and ortho-phenylenediamine (OPD) substrate dissolved in phosphate-citrate buffer solution pH 5 were added. The optical densities (OD) were read by an ELISA reader at a wavelength of 492 nm after the reaction was halted by 2 M-sulfuric acid (Zumaquero-Ríos et al. Reference Zumaquero-Ríos, García-Juarez, De-la-Rosa-Arana, Marcet and Sarracent- Pérez2012).
Nano-based ELISA
Nano-based ELISA was performed to detect T. spiralis circulating antigen in both the tested and control serum, as was done under standard ELISA. However, in Nano-based ELISA the prepared Ag nano probes served as secondary antibodies instead of the anti-rabbit polyclonal IgG-HRP conjugate.
Calculation and interpretation of ELISA readings
The OD values of the tested and control serum were evaluated by calculating the mean OD of the readings. The mean OD of the negative control was added to twice the standard deviation to calculate the cut off value (COV). If the sample’s OD was equal to or greater than the COV, it was considered positive for T. spiralis, while OD values less than the COV were considered a negative sample. The sensitivity, specificity, and negative and positive predictive values of different assays were calculated according to DeLong (Reference DeLong, Vernon and Bollinger1985).
Design of primers
Primer was designed based on a nuclear 1.6 kb repetitive element (Rep) from the T. spiralis DNA sequences existing in the GenBank database (https://www.ncbi.nlm.nih.gov/genbank/) with access number X06625. Amplicon length was 144 bp for Rep (Synbio Technologies, Monmouth Junction, NJ, USA).
DNA isolation and real-time PCR
For DNA extraction, each blood sample was collected individually in 5 mM EDTA and stored at -20°C prior to DNA purification. DNA was extracted using GF-1 Blood DNA Extraction Kit (#GF-BD-100, Vivantis Technologies, Oceanside, CA, USA) as guided by the manufacturer’s instructions.
Real time PCR was done with SensiFAST™ Direct Probe SuperMix PCR kit (Cat# BIO-86120, Meridian, Bioline, Memphis, TN, USA), which was utilized for amplification of extracted DNA followed by PCR. The components of the real-time PCR mixture were 25 μL SensiFAST Probe Direct SuperMix (2x), 2.5 μL forward primer (REP (5´ TTCTATTCTGCTACTGCTAACACTTCGA ´3) (Synbio Technologies), 2.5 μL, reverse primer (5´ TGGTGTGGCTCAAGAATGGA ´3) (Synbio Technologies),10μl Template DNA, and 10μl nuclease free water in a 50 μl final volume. The prepared reaction mix samples were applied in real time PCR (Step One Applied Biosystems, Foster City, CA, USA) under the following cycling conditions: Reverse Transcription (RT) by 1 cycle at 55°C for 10 min; real time enzyme inactivation by 1 cycle at 95°C for 2 min; amplification by 40 cycles; denaturation at 95°C for 10 sec; annealing at 55°C for 10 sec; and extension at 72°C for 30 sec then 1 cycle final extension at 72°C for 5 min.
Calculation of Relative Quantification (RQ) (relative expression)
The data of RT-PCR were expressed in Cycle threshold (Ct). The PCR data sheet involves Ct values of the assessed gene (REP) compared to the corresponding housekeeping gene (β-actin). A control sample was used to assess the expression of certain genes. The RQ of each target gene was measured and normalized to the housekeeping gene consistent with the calculation of delta-delta Ct (ΔΔCt) (Muller et al. Reference Muller, Janovjak, Miserez and Dobbie2002). The RQ of each gene was calculated by taking 2-∆∆Ct.
Statistical analysis
Agreement in the form of sensitivity, specificity, and accuracy of standard ELISA, Nano-based ELISA, and PCR as well as positive predictive and negative predictive values of the assays were calculated. Fisher’s exact (FE) test was used to compare the studied groups.




N is the total sample number, TP is true positive, FP is false positive, TN is true negative, and FN is false negative. The diagnostic indices (sensitivity, specificity, positive and negative predictive values) of different assays were calculated according to DeLong (Reference DeLong, Vernon and Bollinger1985).
Results
Parasitological results
Regarding the number of adult worms, the highest means and SDs in both mild and severe infection were detected at 6 dpi in group 2B (38.67 ± 5.68) and 3B (97.89 ± 6.88), whereas the lowest means and SDs in both mild and severe infection were detected at 15 dpi in group 2E (8.56 ± 1.81) and 3E (23.56 ± 6.04), with statistically significant differences between the studied groups (P < 0.001) (Table 1).
Table 1. Comparison between mean and SD of intestinal adult trichinellosis among different subgroups of both the mild and severe infection groups

* F = One way ANOVA test;
** P ≤ 0.001 is highly statistically significant
Serological assays
Detection of T. spiralis circulating antigen by traditional ELISA
Standard ELISA began to detect T. spiralis circulating antigen in serum collected at 6 dpi in the mild infection group (subgroup 2B) with sensitivity of 44.4%, which reached 77.7%, 88.8%, and 100% at 8 dpi, 10 dpi, and 15 dpi, respectively. However, in severely infected subgroups it could detect T. spiralis circulating antigen in serum collected at 4 dpi (subgroup 3A) with sensitivity of 44.4%, which reached 66.7% at 6 dpi and 100% at 8 dpi, 10 dpi, and 15 dpi. There were statistically significant differences between studied groups (P = 0.002) (Table 2).
Table 2. Sensitivity of the traditional ELISA test among different subgroups of both the mild and severe infection groups

* P ≤ 0.05 is statistically significant.
** P ≤ 0.001 is highly statistically significant.
Detection of T. spiralis circulating antigen by Nano-ELISA test
Nano-based ELISA began to detect T. spiralis circulating antigen in both the mild and severe infection groups in serum collected at 4 dpi in subgroups 2A and 3A, with sensitivity of 55.5% and 77.8% and accuracy of 63.6% and 81.8%, respectively. This sensitivity reached 100% at 8 dpi in the mild infection subgroup (subgroup 2C) and at 6 dpi in the severe infection subgroup (subgroup 3B), with statistically significant differences between studied groups (P < 0.001) (Table 3).
Table 3. Performance of Nano-based ELISA test among different subgroups of both the mild and severe infection groups

* P ≤ 0.05 is statistically significant.
** P ≤ 0.001 is highly statistically significant.
Molecular assay
Rep gene was detected in the mild infection group at 4 dpi with sensitivity 55.6% (subgroup 2A). This sensitivity increased to reach 88.9% at 6 dpi (subgroup 2B) and 100% sensitivity and accuracy at 8 dpi (subgroup 2C) with statistically significant differences between studied subgroups (P < 0.001). However, in severe infection groups, sensitivity and accuracy reached 100% at 4 dpi (subgroup 3A) with statistically non-significant differences between subgroups (P > 0.999) (Table 4). The best cutoff of quantitative PCR in prediction of severe infection was ≥ 2.5841 with area under the curve of 0.9, sensitivity of 80%, specificity of 81.5%, and positive and overall accuracy of 80.8% (P < 0.001) (Table 5, Figure 2).
Table 4. Performance of Rep gene PCR test among different subgroups of both the mild and severe infected group

** P ≤ 0.001 is highly statistically significant.
Table 5. Performance of quantitative PCR in diagnosis of severity in all studied groups

** P ≤ 0.001 is highly statistically significant.
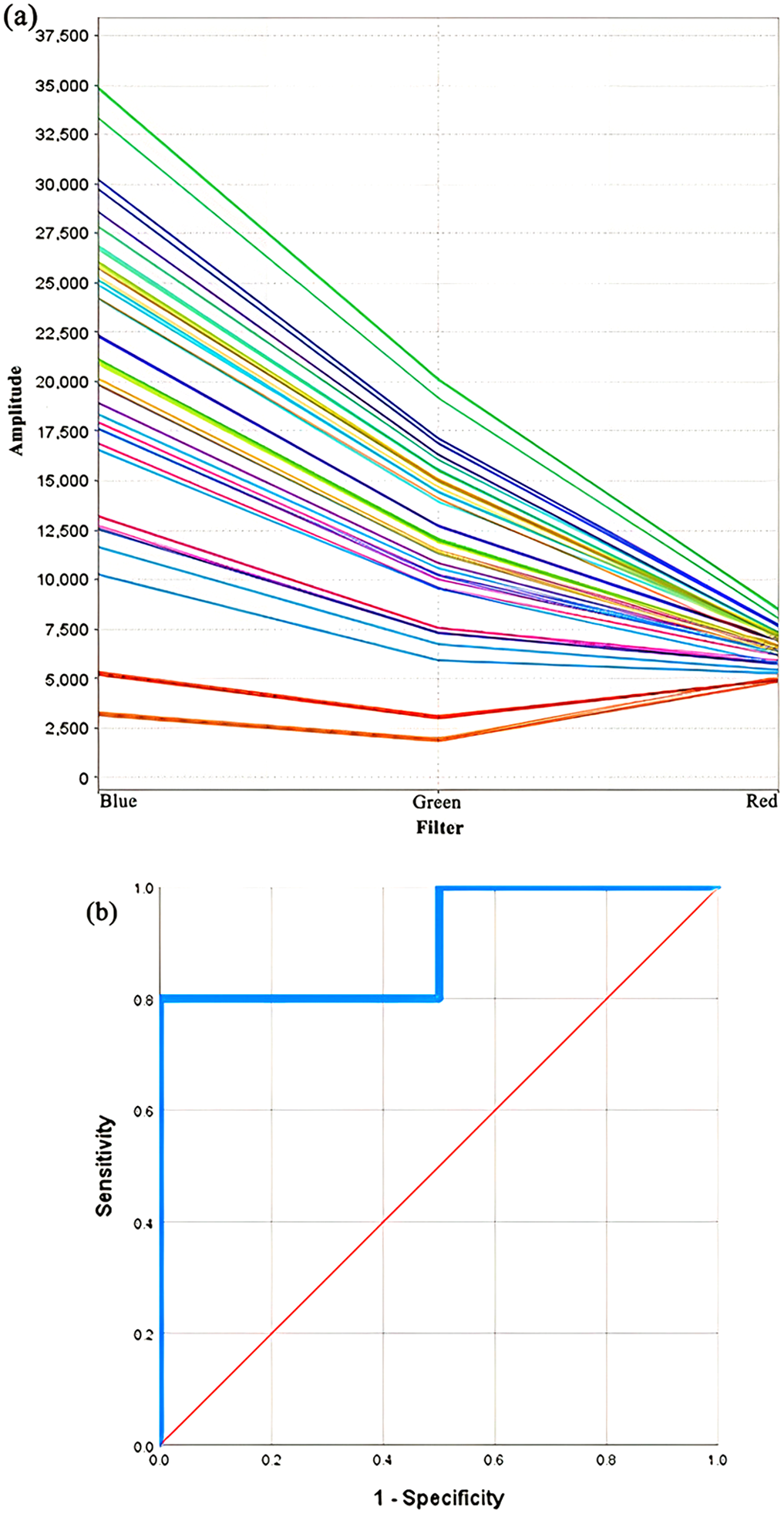
Figure 2. (a) SYBR Green fluorescence chart for DNA samples of mice, resulting from quantitative PCR and Rep gene expression; (b) Receiver operating characteristic (ROC) curve showing performance of quantitative PCR in diagnosis of severe infection. The best cutoff of quantitative PCR in prediction of severe infection was ≥ 2.5841 with area under curve of 0.9, sensitivity of 80%, specificity of 81.5%, positive predictive value of 78.3%, negative predictive value of 83%, and overall accuracy of 80.8% (P < 0.001).
When comparing the validity of traditional ELISA and Nano-based ELISA with PCR as a gold standard technique, it was observed that Nano-based ELISA gave the best results at Time 3 (8 dpi) in the mild infection group, and it had good results compared to PCR in early diagnosis of severe trichinellosis. It could detect infection from Time 1 (4 dpi) with sensitivity of 77.8%, which is lower than PCR sensitivity (100%) at this time, and gave the same results as PCR at Time 2 (6 dpi). Traditional ELISA had the lowest results in early diagnosis of T. spiralis. It attained the best results at 15 dpi in the mild infection group; however, in the severely infected group it could detect infection at Time 1 (4 dpi) but with lower sensitivity of 44.4%. At Time 2 (6 dpi) its sensitivity was still lower than PCR and Nano-ELISA then reached the same sensitivity as PCR and Nano-ELISA (100%) at Time 3 (8 dpi), 4 (10 dpi), and 5 (15 dpi) (Table 6).
Table 6. Validity of ELISA and Nano-ELISA in diagnosis of parasitic infection after using PCR as a gold standard test

Discussion
Trichinellosis is a common meat-borne zoonotic infection that affects humans and a wide variety of animal species (Pozio Reference Pozio2015). In severe cases, complications usually develop in the first two weeks of infection, although they have been reported in moderate cases with late treatment (Zarlenga et al. Reference Zarlenga, Wang and Mitreva2016). Early diagnosis of infection is crucial to initiation of effective treatment and prevention of deadly complications (Wang et al. Reference Wang, Shi, Liu, Jiang, Guan, Chen and Cui2017a).
Confirming a clinical diagnosis of human trichinellosis during the early stage of infection is difficult, and it can be misdiagnosed as alimentary intoxication or enterobacterial infection (Bai et al. Reference Bai, Hu, Liu, Tang and Liu2017). Many serological tests have been used for diagnosis of human trichinellosis, but even so, there is an apparent time gap (window period) between the development of clinical symptoms and positive serology. This window period coincides with the start of the parenteral phase when the NBL are released into circulation (Cui et al. Reference Cui, Wang, Sun, Liu, Zhang, Liu, Zhang, Jiang and Wang2015). ELISA using excretory/secretory antigens of T. spiralis muscle larvae is the frequently used serological test for diagnosis of trichinellosis (Gottstein et al. Reference Gottstein, Pozio and Nöckler2009). However, a high rate of false negative results can be obtained because most T. spiralis muscle larvae excretory-secretory antigens are stage-specific and are not detected by antibodies elicited by the parasites during the enteral phase (Wang et al. Reference Wang, Shi, Liu, Jiang, Guan, Chen and Cui2017a). Moreover, muscle larvae excretory-secretory antigens are cross-reactive with other helminths infections (Yera et al. Reference Yera, Andiva, Perret, Limonne, Boireau and Dupouy-Camet2003; Cui et al. Reference Cui, Wang, Sun, Liu, Zhang, Liu, Zhang, Jiang and Wang2015). Since the IgG and IgM antibodies can persist in humans for at least one year after infection, diagnostic procedures dependent on antibody detection are unable to distinguish between acute and past infections (Sun et al. Reference Sun, Wang, Liu, Jiang and Liu2015a). T. spiralis circulating antigen detection is a reliable approach to distinguish between previous and new infection. Therefore, improving the sensitivity of the ELISA assays currently in use for detecting T. spiralis antigen may facilitate accurate early diagnosis.
Nano-based ELISA was developed by combination of conventional ELISA and nanotechnology found to be an early sensitive diagnostic assay in the acute phase of T. spiralis infection in an animal model (Gomaa, Reference Gomaa2020). Molecular detection of the NBL of Trichinella utilizing a repetitive DNA element could be a valuable assay at the early phase of the infection when antibodies are not yet detectable (Krivokapich et al. Reference Krivokapich, Prous, Gatti and Arbusti2019). Several studies have stated that PCR is a highly sensitive method for detection of Trichinella in meat, faeces, and blood (Cuttell et al. Reference Cuttell, Corley, Gray, Vanderlinde, Jackson and Traub2012; Liu et al. Reference Liu, Ren, Shi, Hu, Song, Duan, Zhang, Du, Liu, Jiang, Wang and Cui2017; Ashour et al. Reference Ashour, Aboul Assad, El-Kowrany and Abdel Ghaffar2018; Ojodale et al. Reference Ojodale, Inabo, Ella and Okubanjo2021). In this context, the existing work is concerned with comparing parasitological, serological, and molecular diagnostic methods in early detection of trichinellosis and detecting the sensitivity of Nano-based ELISA and real time PCR in the diagnosis of T. spiralis infection.
In the present study, a statistically significant difference in mean and SD of adult T. spiralis was observed among different subgroups in the mild infection group (G2) (P < 0.001). The highest mean and SD of number of T. spiralis adults was noted in the group sacrificed at 6 dpi, while the lowest mean and SD was in the group sacrificed at 15 dpi. This aligns with Sadaow et al. Reference Sadaow, Intapan, Boonmars, Morakote and Maleewong2013 who found that there is a reduction rate of 33.7% when compared to the number of intestinal T. spiralis adult in mice infected with 100 larvae and sacrificed at 6 dpi and 10 dpi. Another study done on a different species of Trichinella reveled that at 15 dpi, T. spiralis adults count in the intestine was sporadic (0.13 ± 0.35) (Dvorožňáková et al. Reference Dvorožňáková, Hurníková and Kołodziej Sobocińska2010).
Regarding the severe infection group (G3), there was a statistically significant difference among the studied groups (P < 0.001). The highest mean and SD was observed for the group sacrificed at 6 dpi while the lowest mean and SD was in the group sacrificed at 15 dpi. Kołodziej-Sobocińska et al. Reference Kołodziej-Sobocińska, Dvoroznakova and Dzieman2006 reported that, after infection of mice with 400 larvae, the highest number of adult T. spiralis was detected in mouse intestine at 5 dpi with a decline in numbers from the start of the experiment until 20 dpi.
On the other hand, Ding et al. Reference Ding, Bai, Wang, Wang, Shi, Rosenthal, Boireau, Wu, Liu and Liu2016 noticed that the recovery rate of adults was 60% at 1 dpi without any significant change from 5 to 9 dpi. They added that mice infected with a high dose of muscle larvae had eliminated adult worms completely from their gut at 17 dpi. This means that the highest number of adult T. spiralis in mouse intestine was seen at the beginning of the infection at 6 dpis, then the number decreased. This is consistent with the report of Lawrence et al. (Reference Lawrence, Paterson, Wright, Knight and Miller2004) who declared that resistance to the parasite is correlated with expulsion of adults from the intestine via cellular and antibody-dependent mechanisms.
The observed highest means and SDs in groups 2B and 3B, and lowest means and SDs in groups 2E and 3E may possibly indicate that the higher the infective dose, the higher the number of adult worms in the intestine. Our findings were consistent with Xu et al. Reference Xu, Wen, Wang, Hu, Qi, Sun, Liu, Wang and Cui2017—who reported that the recovery of adult worm from their mice infected with 1000 and 2000 muscle larvae was statistically lower than that from mice infected with 3000, 4000, or 5000 muscle larvae—and Dvorožňáková et al. (Reference Dvorožňáková, Hurníková and Kołodziej Sobocińska2010), who noted that mice infected with a low dose of larvae completely eliminated parasite adults from the small intestine until day 20 dpi.
Likewise, Mista et al. (Reference Mista, Piekarska, Houszka, Zawadzki and Gorczykowski2010) reported that the number of adult Trichinella was higher at 7 dpi in mice infected with 500 larvae than those infected with 250 larvae. However, at 10 dpi they observed that the number of adults in their lightly infected group (250 larvae) was higher than in their severely infected group (500 larvae), which was contrary to our finding. They explained their finding by attributing it to the self-cure phenomenon, which occurs as a result of the rapid expulsion of the parasites in the early intestinal phase, as well as the aggregation of inflammatory cells (mostly mast cells), eosinophils, Th1 and Th2 lymphocyte responses, IgE antibody production, and the release of mediators and cytokines. These variable results discouraged us from relying on adult worm count as an assistive method for diagnosis of trichinellosis, both in mild and severe infections.
It is well recognized that antigen detection is a worthy confirmatory test for an early clinical diagnosis since it provides a clear indication of the presence of parasite products in blood (Gomaa Reference Gomaa2020). Therefore, we tested detection of circulating antigen for early diagnosis of T. spiralis infection. In the current work, traditional ELISA could not detect circulating antigen of Trichinella among mild infection subgroups (G2) at 4 dpi and began to detect it at 6 dpi; however, in the severe infection group T. spiralis circulating antigen was detected in serum at 4 dpi with sensitivity of 44.5%, reaching 100% at 8 dpi, 10 dpi and 15 dpi.
Many studies have reported the performance of traditional ELISA in detecting T. spiralis infection. Gomaa (Reference Gomaa2020) found that traditional ELISA begin to detect circulating antigen on 12 dpi with sensitivity of 83.33% and reached 100% at 22 dpi in mice infected with 100 larvae. Also, Wang et al. (Reference Wang, Fu, Jing, Jin, Ren, Jiang and Cui2012) found that T. spiralis circulating antigen was detected as early as 3 dpi in the serum of mice infected with 500 T. spiralis muscle larvae, and its level increased gradually and reached two peaks with detection rate of 40% and 100% at 13 and 24 dpi, respectively. Furthermore, Jing et al. (Reference Jing, Cui, Liu, Liu, Jiang and Wang2014) reported that T. spiralis circulating antigen was detectable as early as 8 dpi then increased radically during 13–15 dpi, peaking at 22 dpi in serum of mice infected with 500 larvae.
The observed statistically significant difference between mild (G2) and severe (G3) infected groups (P = 0.002) means that the level of circulating antigen may relate to the worm burden in the host. This coincides with the study done by Liu et al. (Reference Liu, Jing, Cui, Fu and Wang2013) who found that circulating antigen was detected as early as 4 dpi in the serum of both heavily (300 larvae) and lightly (100 larvae) infected groups. The antigen then increased rapidly, with a peak detection rate of 100% in severely infected mice at 10 dpi and 80% in lightly infected mice at 22 dpi, respectively, using a sandwich ELISA assay based on egg yolk immunoglobulin and IgM monoclonal antibody against T. spiralis muscle larvae excretory-secretory antigens.
Nanotechnology empowers identification of some of microorganisms (Wang et al. Reference Wang, Hu, Jin, Yao and Li2006). The advancement of in vitro diagnostics to the next level of performance is promised by the usage of nanoparticles (1–100 nm size range) (Azzazy et al. Reference Azzazy, Mansour and Kazmierczak2007).
In the current study the performance of Nano-based ELISA was assessed in the early diagnosis of T. spiralis infection. In the mildly infected group (G2), a statistically significant difference was observed between subgroups (P = 0.005). Nano-based ELISA could detect T. spiralis circulating antigen in serum collected at mice sacrificed at 4 dpi; sensitivity and accuracy increased at 6 dpi and reached 100% at 8 dpi, 10 dpi, and 15 dpi. Our results are comparable to those of Gomaa (Reference Gomaa2020), who reported that Nano-based ELISA began to detect circulating antigen at 6 dpi and recorded a statistically significant high sensitivity (58.33%, 91.67%) and accuracy (72.22%, 94.44%) at 8 dpi and 10 dpi, respectively, and reached 100% sensitivity and accuracy at 12 dpi in mice inoculated with 100 muscle larvae.
Regarding the effectiveness of Nano-based ELISA in early diagnosis of T. spiralis in the severe infection group (G3), we observed a statistically non-significant difference between G3 subgroups (P = 0.079). Nano-based ELISA could detect circulating T. spiralis antigen in serum collected at 4 dpi and reached 100% sensitivity and accuracy at 6 dpi, 8 dpi, 10 dpi, and 15 dpi. However, there was a statistically significant difference (P < 0.001) among different subgroups of both the mild (G2) and severe (G3) infection groups. These results signify that Nano-based ELISA has better performance in detecting T. spiralis infection in both the mild (G2) and severe (G3) infection groups.
Our findings are in line with previous studies that used Nano-based ELISA in diagnosis of parasitic infection. Kamel et al. (Reference Kamel, El-Baz, Demerdash, El-Karaksy, El-Gendy, Hassan, Salah, El-Moneem, Hendawy and Aly2016) found that anti-Schistosoma monoclonal antibody conjugated AuNPs exhibited high performance in identifying the circulating Schistosoma mansoni antigen. It was a remarkable finding that AuNPs-immunochromatographic strip test exhibited quicker detection and good performance in diagnosis of brugian filariasis (Makhsin et al. Reference Makhsin, Razak, Noordin, Zakaria and Chun2012).
The advantage of Nano-based ELISA over traditional ELISA in diagnosis of different parasitic infections was previously elucidated by several studies (Moharm et al. Reference Moharm, El Enain, El-Deen, El Aswad, Hendawy and Aly2014; Naser et al. Reference Naser, Younis, Bayoumi, Eraky, Aly and El Attar2017; El-Shafey et al. Reference El-Shafey, Afifi, Oshiba, Aly and Lasheen2018; Khodadadi et al. Reference Khodadadi, Madani, Hoghooghi Rad and Atyabi2021). This promising high performance of Nano-based ELISA was explained by many studies. The immobilized HRP molecules act as signal amplification molecules that catalyze the oxidation of chromogenic substrate into colorful products and detect the presence of the target antigen. The targeted antigen concentration is proportionate to the absorbance value of the emerging-colored yields as determined by the ELISA reader. The traditional ELISA system has a limited sensitivity since it uses enzyme-conjugated antibody and only one antibody molecule can ultimately bind one HRP molecule. Moreover, Nano-based ELISA claims that one secondary antibody can be bonded with several HRP molecules across the nanoparticle bridge, significantly amplifying the signal and increasing the sensitivity of detection (Jia et al. Reference Jia, Zhong, Hua, Liu, Jing, Lou, Yao, Xiang, Jin and Zhao2009). Also, Ding et al. (Reference Ding, Zhao, Peng, He, Zhou, Yuan and Zhang2012) claimed that the use of nanoparticles functionalized with antibodies produces an assay with magnitude sensitivity higher than traditional ELISA because the background noise is reduced by minimizing the non-specific antibodies’ binding. Our finding indicated that obtained high sensitivity of the Nano-based ELISA may potentially be attributable to the use of polyclonal antibody IgG against T. spiralis adult worm circulating antigen given that intestinal worm circulating antigen manifests in blood circulation earlier than muscle larval circulating antigen, and this agrees with Sun et al. (Reference Sun, Wang, Liu, Jiang and Liu2015a, Reference Sun, Liu, Wang, Jiang, Wang, Liu, Liu, Zhang and Cuib), Liu et al. (Reference Liu, Ren, Shi, Hu, Song, Duan, Zhang, Du, Liu, Jiang, Wang and Cui2017), and Wang et al. (Reference Wang, Liu, Sun, Song, Jiang, Zhang and Cui2017b).
During early T. spiralis infection, the NBL enter and circulate in the blood until the adult worms are expelled from the intestine, so PCR may be a suitable tool for diagnosis of Trichinella infection at an early stage in humans and animals who test negative for anti-Trichinella antibodies (Li et al. Reference Li, Wang and Cui2010). Nevertheless, real time PCR provides several benefits over conventional PCR including rapidity, simplicity, reproducibility, and quantitative capability (Yang & Rothman Reference Yang and Rothman2004).
In our study, PCR performance was detected for early diagnosis of T. spiralis infection using Rep primer. Rep was effective in diagnosis of T. spiralis in the immunological ‘silent’ period by SYBR Green real time PCR. Interestingly, it provided an earlier and longer detection of NBL in mice (5–19 dpi) than other nuclear and mitochondrial primers used by Krivokapich et al. (Reference Krivokapich, Prous, Gatti and Arbusti2019).
Regarding the mild infection group (G2), there was a highly significant difference between subgroups (P < 0.001). PCR could detect Rep gene in blood at 4 dpi, with increasing sensitivity at 6 dpi and 8 dpi. These results were near those obtained by Li et al. (Reference Li, Wang and Cui2010), who detected DNA of T. spiralis in blood of mice infected with 20 larvae and 100 larvae at 5–6 dpi and 5–12 dpi, respectively. Also, Attia et al. (Reference Attia, Mahmoud, Othman and Sayed2016), who used PCR to detect DNA of T. spiralis migrating larva in blood samples of mice infected with 200 larvae, showed early detection of infection at 4, 6, and 14 dpi.
In severely infected groups (G3), the Rep gene PCR test showed a statistically non-significant difference between subgroups (P > 0.999). PCR began to detect Rep gene in blood collected at 4 dpi, with sensitivity and accuracy at 100% in all subgroups. These findings imply that PCR may be effective in early detection of Trichinella infection. Likewise, Caballero-Garcia & Jimenez-Cardoso (Reference Caballero-Garcia and Jimenez-Cardoso2001) reported that PCR using the pPRA primers detected T. spiralis DNA in mice infected with 300 larvae from 5–17 dpi. Krivokapich et al. Reference Krivokapich, Prous, Gatti and Arbusti2019 stated that in mice infected with 500 larvae, PCR detected T. spiralis infection from 5 to 19 dpi, and the first day of recognition of the repetitive DNA element by Rep was at 5 dpi. Our findings, however, conflict with some of their findings, as they reported that the detection was intermittent during this period since the used primer gave negative results at days 9, 10, 17, and 18 dpi.
When comparing the performance of PCR among different subgroups of both the mild and severe infection groups, a statistically significant difference was found (P < 0.001). The early detection of Rep gene in both mild and severe infection groups at 4 dpi, but with sensitivity of 100% in the severely infected group, means that the infecting dose of T. spiralis and the time after infection at which the PCR was conducted both affected the diagnostic value of PCR. This agreed with Li et al. (Reference Li, Wang and Cui2010), who indicated that the severity of infection determines the sensitivity of PCR for detecting DNA of the migrating larvae of T. spiralis in mouse blood. They found that T. spiralis DNA was detectable at 5 and 6 dpi in mice infected with 20 larvae with a detection rate of 7.69%. In mice inoculated with 100 larvae, they detected T. spiralis DNA at 5–12 dpi with a maximum rate of 38.46% at 7 dpi, while in mice inoculated with 300 larvae at 5–17 dpi, the rate exceeded 50% from 5 to 10 dpi and attained a peak rate of 61.54% at 7 dpi.
For the first time in the literature, our study compared the validity of traditional ELISA, Nano-ELISA, and PCR in the diagnosis of Trichinella infection. Given that the best results were obtained by PCR, we used PCR as the gold standard technique to which to compare our other tests results. This was done following Beyhan & Tas Cengiz (Reference Beyhan and Taş Cengiz2017), who used real time PCR as the gold standard to test the validity of conventional microscopy and an antigen detection ELISA kit for detection of Giardia intestinalis in human stool specimens. Also, Khalifa et al. (Reference Khalifa, Abdel-Rahman, Bakir, Othman and El-Mokhtar2020) considered nested PCR as the gold standard test in finding the sensitivity and specificity of the Copro-ELISA test to diagnose Capillaria philippinensis infections.
Our finding revealed that PCR was superior to the other tests in early detection of trichinellosis both in mild and severe infection followed by Nano-ELISA. Traditional ELISA gave the lowest results of the three examined tests. This reveals that PCR is best in early diagnosis of mild T. spiralis infection and is considered a valid and reliable test in detecting severe infection. Our finding was consistent with Gomaa (Reference Gomaa2020), who found that circulating antigen was only detected by Nano-based ELISA at 6, 8, and 10 dpi and by both traditional ELISA and Nano-based ELISA at 12–28 dpi. Also, Krivokapich et al. (Reference Krivokapich, Prous, Gatti and Arbusti2019) indicated that molecular techniques for detection of NBL of Trichinella using the repetitive DNA element (Rep) could be beneficial during the early stage of the infection when antibodies are not detectable by traditional ELISA.
Conclusion
We concluded that Rep gene was efficient to detect T.spiralis during the ‘silent’ period by SYBR Green real time PCR with a great role in early diagnosis of mild as well as severe T. spiralis infection. Also, Nano-based ELISA is a promising and sensitive method for early diagnosis of the acute phase of mild and severe T.spiralis, with results as sensitive and early as PCR, so this assay could be used as a potential alternative to PCR. Traditional ELISA had the lowest sensitivity in early diagnosis of T. spiralis mild and severe infection compared to PCR and Nano-ELISA.
Financial support
This research received no specific grant from any funding agency, commercial or not-for-profit sectors.
Competing interest
The authors declare that they have no conflict of interest.
Ethical standard
The study was approved by the Ethics Committee of the Faculty of Medicine, Zagazig University, Egypt (approval number ZU-IACUC/3/F/38/2021).