Introduction
Entomopathogenic nematodes (EPNs) of the family Steinernematidae Travassos, 1927 are lethal obligate pathogens of insects with a worldwide distribution (Poinar, Reference Poinar1979; Adams et al., Reference Adams, Peat, Dillman, Nguyen and Hunt2007; Lis et al., Reference Lis, Sajnaga, Skowronek, Wiater, Rachwał and Kazimierczak2021). Steinernematids have been successfully applied as commercial applications against many insect pests, such as scarab larvae in turf and lawns, insect pests in protected horticulture, mole crickets in lawns and turf, and black vine weevils in nursery plants (Lacey & Georgis, Reference Lacey and Georgis2012; Shapiro-Ilan et al., Reference Shapiro-Ilan, Hazir, Glazer, Kogan and Heinrichs2020).
Steinernematidae comprises two genera: Steinernema and Neosteinernema. To date, more than 100 steinernematid species have been described from all continents except Antarctica, and this number is still growing (Cimen et al., Reference Cimen, Půža, NermuŤ, Hatting, Ramakuwela and Hazir2016; Chaubey & Aasha, Reference Chaubey, Aasha and Omkar2021). Based on phylogenetic analyses, family Steinernematidae can be divided into 12 multispecies clades: ‘Affine’, ‘Bicornutum’, ‘Cameroonense’, ‘Carpocapsae’, ‘Costaricense’, ‘Feltiae’, ‘Glaseri’, ‘Karii’, ‘Khoisanae’, ‘Kushidai’, ‘Longicaudum’ and ‘Monticolum’ (Spiridonov & Subbotin, Reference Spiridonov, Subbotin, Hunt and Nguyen2016).
In recent years, a total of 17 steinernematids have been reported from China, of which two belong to the Affine clade: Steinernema bedding Qiu, Hu, Zhou, Pang and Nguyen 2005 and S. sichuanense Mráček, Nguyen, Tailliez, Boemare and Chen, 2006; one to Bicornutum-clade, namely, S. ceratophorum Jian, Peid and Hunt, 1997; five to Feltiae-clade, namely, S. hebeiense Chen, Li, Yan, Spiridonov and Moens, 2006; S. xueshanense Mráček, Liu and Nguyen, 2009; S. xinbinense Ma, Chen, Clercq, Waeyenberge, Han and Moens, 2012; S. tielingense Ma, Chen, Li, Han, Khatri-Chhetri, Clercq and Moens, 2012 and S. cholashanense Nguyen, Půža and Mráček, 2008; one to Glaseri-clade, S. caudatum Xu, Wang and Li, 1991; two to Karii-clade, namely, S. aciari Qiu, Zhou, Nguyen and Pang 2005 and S. leizhouense Nguyen, Qiu, Zhou and Pang, 2006a; one to Kushidai-clade, namely, S. akhursti Qiu, Hu, Zhou, Mei, Nguyen and Pang, 2005; four to Longicaudum-clade, namely, S. longicaudum Shen and Wang, 1992; S. guangdongense Qiu, Fang, Zhou, Pang and Nguyen 2004; S. pui Qiu, Zhao, Wu, Lv and Pang, 2011 and S. taiwanensis Tseng, Hou and Tang, 2018; and one to Monticolum-clade, namely, S. changbaiense Ma, Chen, Clercq, Han and Moens, 2012.
A recent survey was carried out from 2017 to 2020 in Jilin Province, China, to determine the occurrence and distribution of EPN. The survey resulted in the recovery of more than 40 isolates of EPN, with only one isolate of an unknown Steinernema being described as a new species in the current study. We utilized light microscopy (LM) and scanning electron microscopy (SEM) for morphological observation and morphometric analysis, as well as molecular observations, to fully describe and illustrate this new species.
Materials and methods
Nematode isolation and rearing
The nematode isolate was recovered from soil samples collected from the rhizosphere of a poplar tree (Populus sp.) in Yushu city (44°59′30″N, 126°10′58″E), Jilin Province, China, during a survey in 2019. Collected soil samples (approximately 2 dm3 vol.) were placed into plastic bags. Five last-instar Galleria mellonella (L.) larvae were placed into a small steel mesh pocket and put in these bags with soil, two pockets per plastic bag. These samples were transported to the laboratory.
For taxonomic studies, different life stages of S. populi were obtained from infected last-instar G. mellonella larvae exposed to 100 IJs/insect in a 15 cm-diameter Petri dish lined with moistened filter paper and kept in the dark at 25°C. The G. mellonella larvae died within 48 h after inoculation and insect cadavers turned reddish-brown after 2–5 days. After they died, the insect cadavers were transferred to a modified White trap (Kaya & Stock, Reference Kaya, Stock and Lacey1997) and incubated at 25°C until IJ emerged.
First- and second-generation adults were obtained by dissecting infected insects approximately three days and five days, respectively, after the death of the host. The cadavers were dissected in Ringer's solution.
LM and SEM
For morphometric analysis, measurements were made on specimens fixed in triethanolamine formalin (TAF) (Courtney et al., Reference Courtney, Polley and Miller1955) and mounted in anhydrous glycerine on slides (Seinhorst, Reference Seinhorst1959). Light microphotographs of the fresh individuals and mounted specimens were prepared using a Leica Microsystems TCS SP8 compound microscope (Mannheim, Germany). Micrographs were taken with an automatic camera system (Leica Application Suite X) mounted on a Leica microscope. Drawings and morphological analyses were performed using a drawing tube attached to an Olympus BHA light microscope (Tokyo, Japan) and Adobe Illustrator software version 2017 (Adobe, USA).
Adults of the first generation and IJ were fixed in 4% formalin buffered with 0.1 M sodium cacodylate at pH 7.2 for 24 h at 8°C. They were post-fixed with 2% osmium tetroxide solution for 12 h at 25°C, dehydrated in a graded ethanol series, critical-point dried with liquid carbon dioxide, mounted on SEM stubs and coated with gold (Nguyen & Smart, Reference Nguyen and Smart1995). Specimens were measured and photographed with the aid of a Regulus 8100 (Hitachi, Tokyo, Japan).
Molecular characterization
DNA was extracted from single females, using a modification of a method reported by Nguyen (Reference Nguyen, Nguyen and Hunt2007a). The nematode was placed in 30 μl lysis buffer (50 mm magnesium chloride, 10 mm Dithiothreitol (DTT), 4.5% Tween 20, 0.1% gelatine and 1 μl proteinase K at 60 μg/ml) on the side of a 500 μl microcentrifuge tube, where it was cut into two or more pieces under a dissecting microscope. After being immediately stored at −80°C for at least 15 min, the tube was then incubated at 65°C for 1 h and then at 95°C for 10 min. After centrifugation for 2 min at 12,000 rpm, the supernatant (20 μl) was transferred to a clean microcentrifuge tube, where it was kept at −20°C.
A ribosomal DNA (rDNA) fragment containing the internal transcribed spacer (ITS) regions ITS1 and ITS2, and the 5.8S rRNA gene was amplified by polymerase chain reaction using the forward primer TW81: 5′-GTTTCCGTAGGTGAACCTGC-3′ and the reverse primer AB28: 5′-ATATGCTTAAGTTCAGCGGGT-3′ (Joyce et al., Reference Joyce, Reid, Driver, Curran, Burnell, Ehlers and Masson1994). The other fragment containing D2–D3 expansion segments of the 28S rDNA gene was amplified using the forward primer D2F: 5′-CCTTAGTAACGGCGAGTGAAA-3′ and the reverse primer 536: 5′-CAGCTATCCTGAGGAAAC-3′ (Nguyen, Reference Nguyen, Nguyen and Hunt2007a).
Phylogenetic analysis
The newly obtained ribosomal DNA sequences of the ITS and D2–D3 regions of 28S were deposited in the GenBank (Altschul et al., Reference Altschul, Madden, Schäffer, Zhang, Miller and Lipman1997) (table 1).
Table 1. Details on taxa used in the molecular analyses.

Multiple sequence alignments were produced by the default ClustalW configuration included in MEGA 7 and optimized manually in BioEdit (Hall, Reference Hall1999). Pairwise distances were computed using MEGA 7 (Kumar et al., Reference Kumar, Stecher and Tamura2016). Caenorhabditis elegans (X03680) and Panagrellus redivivus (AF331910) were used as the outgroup taxa for ITS and 28S phylogenetic analyses, respectively.
ModelFinder (Kalyaanamoorthy et al., Reference Kalyaanamoorthy, Minh, Wong, Haeseler and Jermiin2017) was used to select the best-fitting model using the Akaike information criterion. Bayesian inference (BI) analysis of the ITS and D2–D3 regions of 28S under the GTR + F + G4 and GTR + F + I + G4 model was employed to confirm the tree topology using MrBayes 3.2.6 (Ronquist et al., Reference Ronquist, Teslenko and van der Mark2012; Zhang et al., Reference Zhang, Gao, Jakovlić, Zou, Zhang, Li and Wang2020), running four chains for 1 × 107 generations. Burn-in sampled trees (25%) were discarded. The Markov chain Monte Carlo method was used within a Bayesian framework to estimate the posterior probabilities of the phylogenetic trees (Larget & Simon, Reference Larget and Simon1999) and generate a 50% majority-rule consensus tree. The software FigTree version 1.4.3 (Edinburgh, UK)was used to display and edit the trees.
Results
Description of Steinernema populi n. sp.
Measurements
The dimensions of the holotype and paratype specimens are provided in table 2.
Table 2. Morphometrics of Steinernema populi n. sp. All measurements are in μm and take the form: mean ± standard deviation (range).

Description
First-generation male. Body curved posteriorly, mostly J-shaped when heat-relaxed (fig. 1a). First-generation males are larger (average 1378 μm) than second-generation males (average 1185 μm). Cuticle smooth under LM, but striations visible with SEM. Head flattened, almost continuous with body contour, with six lips fused at base; each lip bearing a labial papilla. Outer circle of four cephalic papillae present. Amphidial apertures small, located posterior to lateral labial papillae (fig. 2a). Stoma shallow, narrow, usually with pronounced cheilorhabdions, posterior part funnel-shaped, well cuticularized. Pharynx with cylindrical procorpus and slightly swollen metacorpus. Nerve ring usually surrounding isthmus or anterior part of basal bulb. Excretory pore in centre of ventral side and located at ~2/3 of oesophagus distance from anterior end, rarely slightly posterior to nerve ring (fig. 1b). Testis monorchic, reflexed, consisting of germinal growth zone leading to seminal vesicle (fig. 1a). Spicules paired, symmetrical, slightly curved and yellow in colouration, distally bluntly pointed (fig. 2b, f). Manubrium of spicule, usually elongate (manubrium length/manubrium width of 1.4:1). Calomus distinct, but short. Lamina with two internal ribs, well curved. Velum extending from calomus almost to the end of lamina. Spicule terminus blunt (fig. 2b). Gubernaculum arcuate, c. 76% of spicule length, boat-shaped in lateral view, swollen at middle, with prominent narrow neck. Gubernaculum wings well divided; cuneus pointed (fig. 2c). Tail bluntly conoid, usually concave on ventral side; mucron sometimes absent, if developed, 1.5–2 μm long (figs 1f and 2e). Twenty-three genital papillae comprising 11 pairs and a single ventral papilla located just anterior to cloacal opening of paired papillae, five pairs subventral precloacal, one pair lateral precloacal, two pairs adcloacal subventral and three pairs postcloacal (one pair subdorsal, two pairs subventral terminal) (fig. 2d–f).
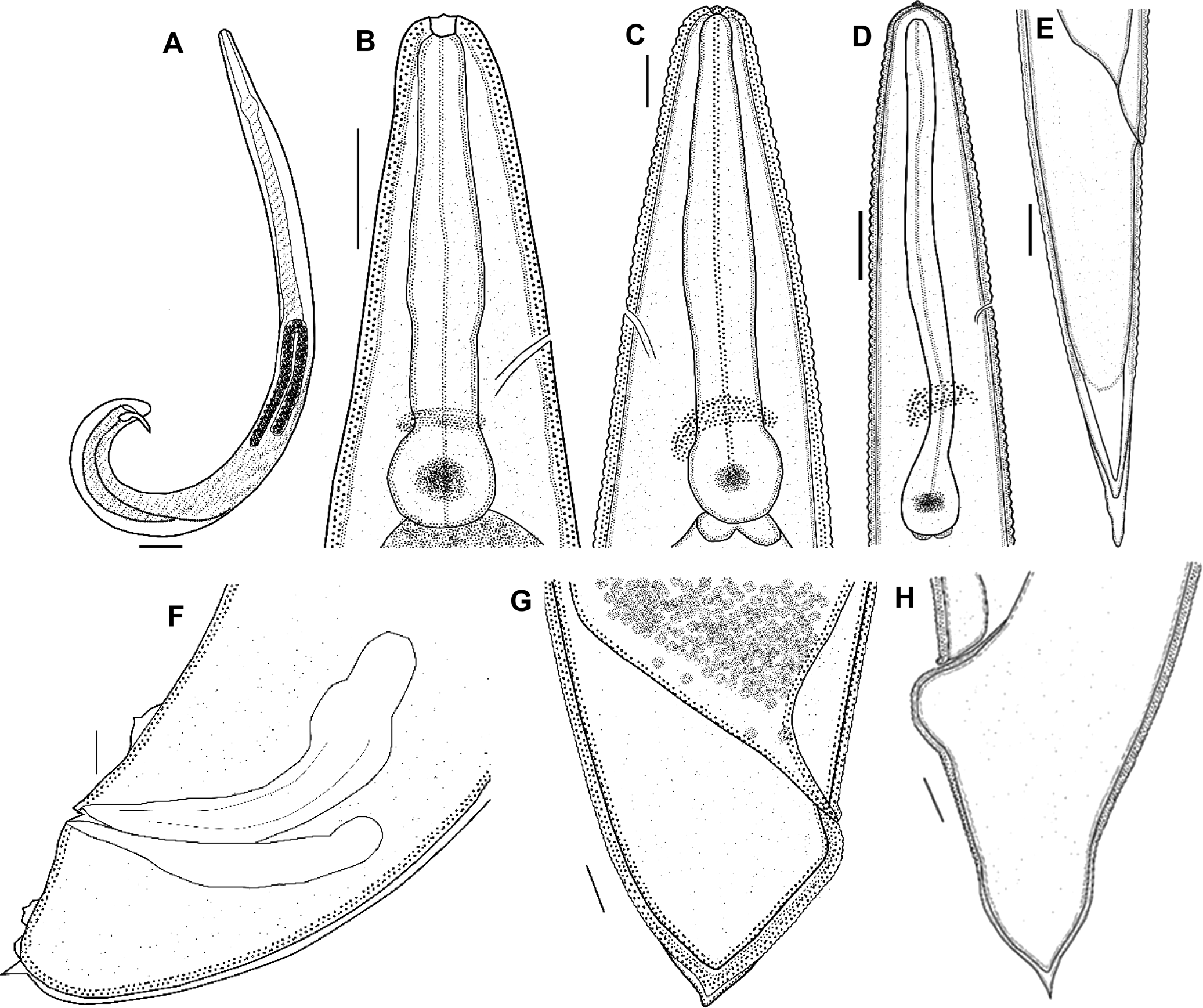
Fig. 1. Steinernema populi n. sp. line drawings. First-generation male: (a) total body, lateral view; (b) anterior end (lateral view), showing stoma region; (f) tail (lateral view), showing part of genital papillae, spicules and gubernaculum. First-generation female: (c) anterior end (lateral view), showing stoma region; (g) tail, lateral view. Second-generation female: (h) tail with mucron. Third-stage infective juvenile: (d) anterior end, lateral view, pharyngeal region, nerve ring and excretory pore; (e) tail, lateral view. Scale bars: (a) 75 μm; (b–d, g) 25 μm; (e, f, h) 10 μm.
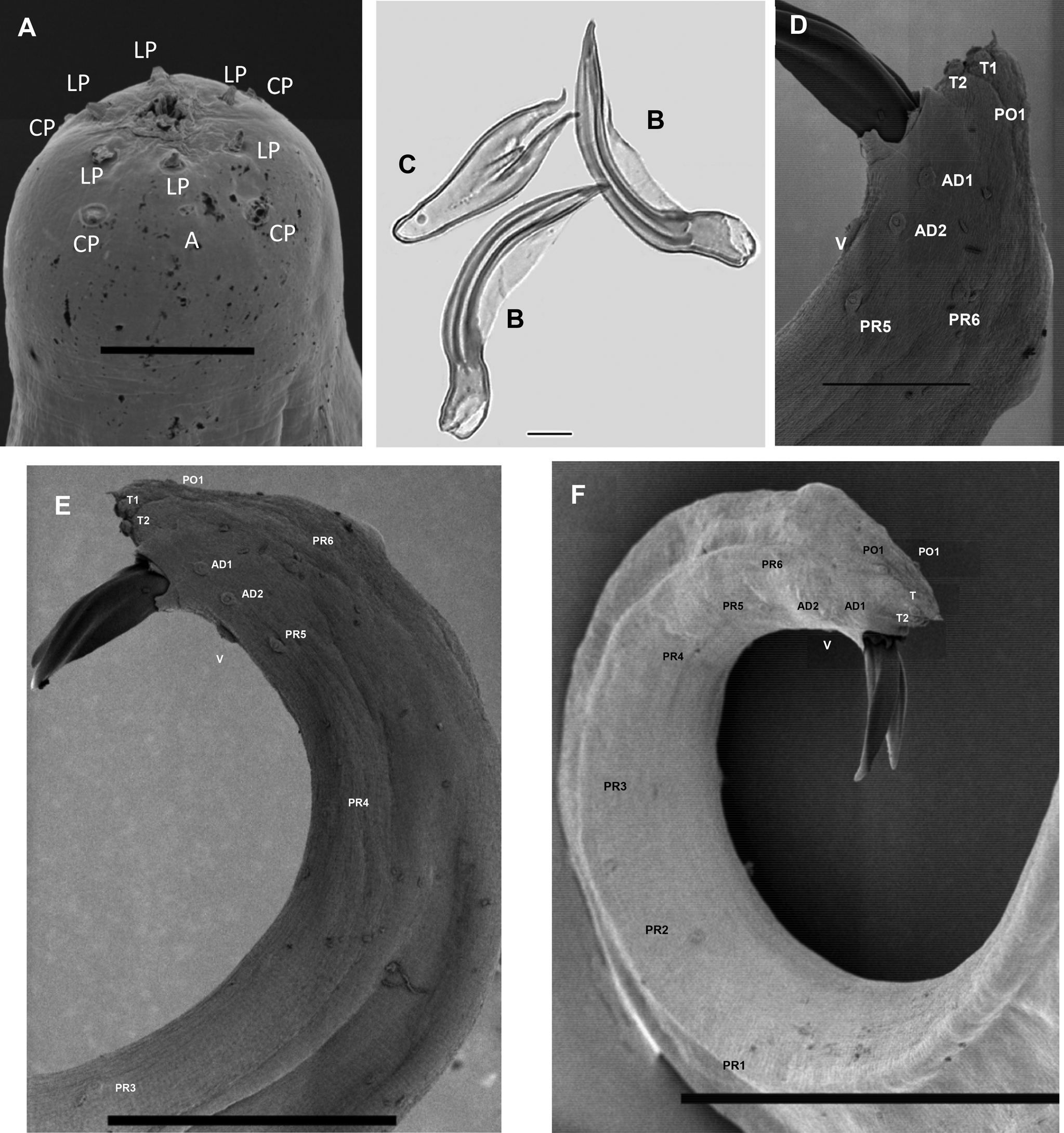
Fig. 2. Steinernema populi n. sp. LM and SEM photographs. First-generation male: (a) en face view, labial papillae (LP), cephalic papillae (CP), amphid (a); (b, c) spicule and gubernaculum; (d–f) tail region, showing 11 pairs of papillae and single ventral papilla, adanal papilla 1 (AD1); adanal papilla 2 (AD2); ventral papilla (V); terminal papillae1 (T1); terminal papillae 2 (T2); post-anal papillae 1 (PO1); post-anal papillae 2 (PO2); pre-anal papillae1–6 (Pr 1–6). Scale bars: (a) 5 μm; (b, c) 10 μm; (d) 20 μm; (e) 50 μm; (f) 100 μm.
Second-generation male. General morphology similar to that of the first-generation male, but slightly smaller in body length and other morphometric values (table 2). Tail with same shape as that of the first-generation male and with or without mucron.
First-generation female. Body size varies significantly, rarely longer than 13,762 μm, usually C-shaped when heat-relaxed. Lip region and stoma region as in males (fig. 2a). Cuticle only faintly striated. First-generation females larger (average = 7026 μm) than second-generation females (average = 3272 μm). Pharynx with procorpus cylindrical and muscular, isthmus distinct, basal bulb enlarged. Nerve ring anterior to basal bulb, surrounding isthmus. Excretory pore located anterior to nerve ring (figs 1c and 3a). Reproductive system didelphic, amphidelphic, ovaries opposed, reflexed, glandular spermatheca, uterus in ventral position. Vulva in the form of transverse slit located slightly posterior to mid-body. Vulval lips slightly protruding, asymmetrical, with larger posterior lip (fig 3b). Tail length much shorter than anal body diameter, with slight postanal swelling. Tail blunt, conoid, lacking mucro. Postanal lips usually protruding, asymmetric with anterior lip smaller than posterior (fig. 1g).

Fig. 3. Steinernema populi n. sp. LM and SEM photographs. First-generation female: (a) anterior region, showing pharynx, excretory pore (EP) and nerve-ring (NR) (arrow), lateral view; (b) vulva, lateral view. Second-generation female: (c) vulva, lateral view. Scale bars: (a, c) 50 μm; (b) 25 μm.
Second-generation female. Similar to first-generation female but smaller and slenderer (table 2). Vulva shape and lips similar to those of first-generation female (fig. 3c). Vulva situated at mid-body (~55%). Pygmy forms observed. Excretory pore located more posteriorly than in first-generation female. Tail tip pointed but not mucronate (fig. 1h), tail longer than body width at anus. Postanal swelling slightly developed.
Third-stage infective juvenile. Body of heat-relaxed specimens almost straight or gently curved and slightly narrowed at the anterior and posterior ends. Head region continuous with body, rounded to slightly truncate, not annulated. Four conspicuous cephalic papillae and a pair of pore-like amphidial apertures laterally (fig. 4a). Lip region smooth, continuous; stoma closed. Cuticle transversely ribbed (fig. 1d). Lateral fields consisting of eight equally spaced and developed ridges in mid-body region (fig. 4b). Lateral field formula: 2, 4, 6, 8, 6, 2. Oesophagus with narrow corpus, slightly swollen metacorpus, isthmus surrounded by nerve ring. Excretory pore in the middle between anterior end and basal bulb (fig. 1d). Hemizonid distinct. Deirids not observed. Phasmid prominent in mid-tail just ventral to lateral field. Tail conoid with pointed terminus (fig. 4d). Hyaline portion occupying c. 35% of tail length.

Fig. 4. Steinernema populi n. sp. SEM photographs. Third-stage infective juvenile: (a) en face view, labial papillae (LP), cephalic papillae (CP), amphid (a); (b) lateral field in mid-body, ridges numbered 1–8; (c) anus, lateral view; (d) tail, lateral view. Scale bars: (a, c) 5 μm; (b) 50 μm; (d) 20 μm.
Taxonomic summary
Type material. Holotype, first-generation male; paratype, third-stage juveniles, males and females of first and second generations mounted on slides and deposited in the Nematode Laboratory, Institute of Plant Protection, Jilin Academy of Agricultural Sciences, China.
Many males and females of the first generation and several third-stage infective juveniles were deposited in the US Department of Agriculture Nematode Collection (USDA NC), Beltsville, Maryland, USA (USDANC numbers are T-7612p to T-7628p).
Several males, females and infective juveniles were deposited in the Shenyang International Nematode Collection (SINC), National Parasitic Resource Center (SINC number is 20210555) (https://www.tdrc.org.cn/), China.
Type host. The natural host is unknown.
Type locality. Steinernema populi n. sp. was recovered by baiting with G. mellonella larvae from soil samples collected from the rhizosphere of a poplar tree (Populus sp.) in Yushu city (44°59′30″N, 126°10′58″E), Jilin Province, China.
Etymology. The specific epithet refers to the Populus.
Diagnoses and relationships
Steinernema populi n. sp. was characterized by the morphology and morphometrics of IJ and males (table 2). The IJ of S. populi n. sp. can be recognized by the largest body length of 1095 μm, oesophagus length of 149 μm, a tail length of 64 μm, ratios b = 7.4 and c = 17, E% = 121 and H% = 35 (table 2). An excretory pore is located in approximately the mid-pharynx region, and lateral field pattern is 2, 4, 6, 8, 6, 2. The male of the first generation is characterized by a curved spicule length of 66 μm, a gubernaculum length of 44 μm, maximum body diameter of 82 μm, D% = 69 and the presence of a short tail mucron in the second generation (sometimes also present in first-generation males) (table 2).
Steinernema populi n. sp. belongs to species of the ‘Feltiae–Kushidai–Monticolum’ superclade, which comprises over 20 species. Phylogenetic analyses also placed S. populi n. sp. in the Kushidai clade (as proposed by Spiridonov and Subbotin, Reference Spiridonov, Subbotin, Hunt and Nguyen2016). Molecular data show that within this clade, S. populi n. sp. is sister to the pair of S. akhursti and S. kushidai.
The IJ of S. populi n. sp. can be distinguished from S. akhursti (Qiu et al, Reference Qiu, Hu, Zhou, Mei, Nguyen and Pang2005) by body length (1095 vs. 812 μm), the distance from head to excretory pore and nerve ring, which is higher, and by excretory pore, which is more posterior (D% 55 vs. 47). The oesophagus length is much longer (149 vs. 119 μm), while the tail is much shorter (64 vs. 73 μm), and it has a higher E% (121 vs. 77) (table 3). The first-generation males of S. populi n. sp. differ from that of S. akhursti by much shorter spicule and gubernaculum (66 vs. 90 μm and 46 vs. 64 μm, respectively), higher D% (69 vs. 56) and lower SW% (129 vs. 180) (table 4).
Table 3. Comparison of morphometrics of third-stage infective juveniles of Steinernema populi n. sp. with other members of clade III. Measurements are in μm and take the form: mean (range).

a Abbreviations as in Table 2; –, Measurements not available.
Table 4. Comparison of morphometrics of first-generation males of Steinernema populi n. sp. with other members of clade III. Measurements are in μm and take the form: mean (range). Data for new species in bold.

a Abbreviations as in table 1.
MUC, mucron; P, present; A, absent; NA, measurements not available.
Steinernema populi n. sp. differs from S. kushidai (Mamiya, Reference Mamiya1988) by its longer body length of IJ (1095 vs. 589 μm), bigger maximum body diameter (36 vs. 26 μm), longer distance from anterior end to excretory pore (77 vs. 46 μm), longer tail length (64 vs. 50 μm) and higher ratio c and higher E% (17 vs. 11.7 and 121 vs. 92, respectively) (table 3). The first-generation males of the new species differ from that of S. kushidai in higher D% (69 vs. 51) and lower SW% (129 vs. 150) (table 4).
The IJ of S. populi n. sp. differs from S. cholashanense, S. hebeiense, S. tielingense, S. xinbinense and S. xueshanense by the longest body length and oesophagus length, the highest ratios b, c and E%, and the shortest tail. The position of the IJ nerve ring at 106 μm is more anterior than that in S. feltiae at 113 μm, and posterior than that in S. puntauvense at 54 μm, S. nguyeni at 80 μm, the tail (64 μm) is longer than S. weiseri (60 μm) and shorter than S. kraussei (79 μm) (table 3). The males of S. populi n. sp. differ from S. cholashanense, S. hebeiense, S. tielingense, S. xinbinense and S. xueshanense by the slenderest maximum body diameter. The SW% is higher at 129 than that of S. cholashanense at 115 and lower than S. tielingense at 191 (table 4).
Molecular characterization and phylogenetic analysis
Steinernema populi n. sp. was characterized genetically by the sequences of the ITS (MZ367621) and D2–D3 (MZ367685.2) regions of 28S rDNA. The BLAST search performed using the ITS-rDNA sequence revealed that the highest similarity with all currently available ITS sequences of Steinernema is less than 90.94% with S. akhursti (accession number DQ375757) and 88.41% with S. kushidai (AB243440). Based on the 28S rDNA sequence, the new species showed 97.61% similarity to S. weiseri (FJ165549) and 96.26% similarity to S. akhursti (KF289902).
Steinernema populi n. sp. is characterized genetically by sequences of ITS and D2–D3 regions of rDNA. The sequence of ITS regions of S. populi n. sp. is 739 bp. Pairwise distances (table 5) showed that the new species differs from S. akhursti, its closest taxon, by 84 bp. Distances from the new species and others are presented in table 5.
Table 5. Pairwise analysis of the differences in base pairs of the ITS regions between closely related Steinernema species and S. populi n. sp. Data for new species in bold.

For ITS regions, BI analysis shows that the alignment resulted in 1493 characters, of which 265 are constant, 493 variable characters are parsimony-uninformative and 735 characters (included) are parsimony-informative. The phylogenetic relationships between 51 species of Steinernema are presented in fig. 5. In this consensus tree, 18 species of the ‘Feltiae–Kushidai–Monticolum’ superclade form a monophyletic assemblage in which the new species, S. kraussei, S. silvaticum, S. xinbinense, S. tielingense, S. xueshanense, S. oregonense, S. sangi, S. cholashanense, S. feltiae, S. ichnusae, S. nguyeni, S. hebeiense, S. kushidai, S. akhursti, S. monticolum, S. changbaiense and S. ashiunense form a monophyletic group, S. populi n. sp. as a member of the Kushidai clade, and sister to a clade (Bayesian posterior probability (BPP): 1.0) that encompasses S. akhursti and S. kushidai (fig. 5).

Fig. 5. Bayesian 70% majority-rule consensus tree inferred from ITS rDNA of Steinernema populi n. sp. under the GTR + F+G4 model. BPPs of more than 70% are given for appropriate clades. The new sequence is in bold font. The scale bar indicates the number of nucleotide substitutions per site.
The sequence of D2–D3 regions of S. populi n. sp. is 890 bp. Pairwise distances (table 6) show that the new species differs from its closest taxa, S. akhursti and S. kushidai, by 11 bp and 20 bp, respectively. These data indicate that the new nematode is a new species when comparing these distances with those of other species in table 6.
Table 6. Pairwise analysis of the differences in base pairs of the D2–D3 regions between closely related Steinernema species and S. populi n. sp. Data for new species in bold.

Note: GS, gubernaculum length divided by spicule length; SW, spicule length divided by anal body width; V%, distance from anterior end to vulva/Body length; a, Body length/Maximum body diameter; b, Body length/Oesophagus base; c, Body length/Tail length; c′, Tail length/Maximum body diameter; D%, Anterior end to excretory pore in % of pharynx length; E%, (Anterior end to Excretory pore/ Tail length) × 100; H, Hyaline tail.
For D2–D3 regions, BI analysis shows that the alignment resulted in 1044 characters, of which 444 are constant, 343 variable characters are parsimony-uninformative and 257 characters (included) are parsimony-informative. The phylogenetic relationships between 40 Steinernema species are presented in fig. 6. The 16 species, S. populi n. sp., S. cholashanense, S. xinbinense, S. kraussei, S. oregonense, S. texanum, S. xueshanense, S. feltiae, S. puntauvense, S. jollieti, S. weiseri, S. akhursti, S. everestense, S. kushidai, S. ashiuense and S. monticolum, form a monophyletic group. In this clade, the new species, S. akhursti and S. everestense (BPP: 0.97), form a monophyletic group; S. monticolum and S. ashiuense form another monophyletic group.

Fig. 6. Bayesian 70% majority-rule consensus tree inferred from D2–D3 regions of 28S rDNA of Steinernema populi n. sp. under the GTR + F+I + G4 model. BPPs of more than 70% are given for appropriate clades. The new sequence is in bold font. The scale bar indicates the number of nucleotide substitutions per site.
Morphological studies and molecular analyses show that the studied nematode is a new species evolving independently from its sister taxa. In general, morphological and molecular data confirm the status of S. populi n. sp. as a new species according to the phylogenetic and evolutionary species concepts (Adams, Reference Adams1998).
Acknowledgements
We thank Dr RC Han, Institute of Zoology, Guangdong Academy Of Sciences, and Dr LH Qiu, School of Life Sciences, Sun Yat-Sen University, for their guidance in morphological descriptions and electron microscopy. We also thank Ilouise B., MA from American Journal Experts for making any language corrections.
Financial support
This work was supported by the Basic Research Funding of Jilin, China (project number KYJF2021ZR014), Jilin Provincial Science and Technology Development Plan (20210202092NC), Innovative Engineering International Scientific and Technological Cooperation Project of Jilin, China (CXGC202109GH) and the National Key R&D Program of China (2019YFE0120400).
Conflicts of interest
None.
Ethical standards
The authors assert that all procedures contributing to this work comply with the ethical standards of the relevant national and institutional guides on the care and use of laboratory animals.
Author contributions
C.L. Tian# and F. Zhu# contributed equally to this work. Conceptualization, Methodology, Software, Validation, Investigation, Writing-original draft, Writing-review & editing, Visualization. X.Y. Li: Writing-review and editing, Supervision. J.H. Zhang : Soil sample collection, Formal analysis. V. Půža: Morphological identification. D. Shapiro-Ilan: Writing-review and editing. D. Zhao: Observation SEM. J.W. Liu: Soil sample collection. J.J. Zhou: Raise Galleria mellonella. Y. Ding: Raise Galleria mellonella. J.C. Wang: Data curation, Visualization, Supervision. J. Ma: Visualization, Supervision. X.F. Zhu: Nematodes drawing. M.H. Li* and J.P. Li* were co-correspondence. Conceptualization, Resources, Writing-review and editing, Supervision, Project administration, Funding acquisition.