Introduction
The fumarole activity of the scoria cones of the Great Tolbachik fissure eruption of 1975–1976 (Fedotov and Markhinin, Reference Fedotov and Markhinin1983) and the Tolbachik fissure eruption 2012–2013 (Karpov et al., Reference Karpov, Krivovichev, Vergasova, Chernyat'eva, Anikin, Moskaleva and Filatov2013) is accompanied by intensive exhalation mineralisation, in which sulfate mineralisation plays one of the leading roles. Nowadays, more than 25 new sulfates are described on the Tolbachik volcano, Kamchatka peninsula, Russia, most of which are found on the Second scoria cone of the Great fissure Tolbachik eruption (Pekov et al., Reference Pekov, Agakhanov, Zubkova, Koshlyakova, Shchipalkina, Sandalov, Yapaskurt, Turchkova and Sidorov2020). Recently, many new sulfates have been discovered, including Na anhydrous sulfates without additional anions: ivsite, Na3H(SO4)2 (Filatov et al., Reference Filatov, Karpov, Shablinskii, Krivovichev, Vergasova and Antonov2013), bubnovaite, K2Na8Ca(SO4)6 (Gorelova et al., Reference Gorelova, Vergasova, Krivovichev, Avdontseva, Moskaleva, Karpov and Filatov2016), puninite, Na2Cu3O(SO4)3 (Siidra et al., Reference Siidra, Nazarchuk, Zaitsev, Lukina, Avdontseva, Vergasova, Vlasenko, Filatov and Karpov2017), saranchinaite, Na2Cu(SO4)2 (Siidra et al., Reference Siidra, Lukina, Nazarchuk, Depmeier, Bubnova, Agakhanov, Avdontseva, Filatov and Kovrugin2018), itelmenite, Na2CuMg2(SO4)4 (Nazarchuk et al., Reference Nazarchuk, Siidra, Agakhanov, Lukina, Avdontseva and Karpov2018), belomarinaite, KNaSO4 (Filatov et al., Reference Filatov, Shablinskii, Vergasova, Saprikina, Bubnova, Moskaleva and Belousov2019), koryakite, NaKMg2Al2(SO4)6 (Siidra et al., Reference Siidra, Nazarchuk, Zaitsev and Vlasenko2020), natroapthitalite, Na3K(SO4)2 (Shchipalkina et al., Reference Shchipalkina, Pekov, Chukanov, Zubkova, Belakovskiy, Koshlyakova, Britvin, Sidorov and Vozchikova2020), metathénardite, Na2SO4 (Pekov et al., Reference Pekov, Shchipalkina, Zubkova, Gurzhiy, Agakhanov, Belakovskiy, Chukanov, Lykova, Vigasina, Koshlyakova, Sidorov and Giester2019) and dobrovolskiite, Na4Ca(SO4)3 (Shablinskii et al., Reference Shablinskii, Filatov, Vergasova, Moskaleva, Avdontseva and Bubnova2020). Reviews on the fumarolic mineralisation of the Tolbachik scoria cones have been provided by Vergasova and Filatov (Reference Vergasova and Filatov2012, Reference Vergasova and Filatov2016).
Petrovite has been approved by the Commission on New Minerals, Nomenclature and Classification of the International Mineralogical Association (IMA2018-149b, Filatov et al., Reference Filatov, Shablinskii, Vergasova, Krivovichev and Moskaleva2020). The mineral is named in honour of Prof. Dr. Tomas Georgievich Petrov (b. 1931), a former chairman of the Crystallogenesis Laboratory of the Department of Crystallography, St. Petersburg State University. The scientific activities of Tomas Petrov were devoted to the modelling of crystal growth of minerals (Petrov et al., Reference Petrov, Treywus and Kasatkin1969). He was the first to develop the industrial technology of fabrication of jewellery malachite back in 1977 (Petrov et al., Reference Petrov, Protopopov and Shuyskiy2013). Tomas Petrov is the author of the two-parameter Alphabet for the Coding of Structural–Chemical Information and RHAT-catalogue of modal mineral compositions of magmatic rocks (Petrov et al., Reference Petrov, Andriyanets-Buyko and Moshkin2012; Petrov, Reference Petrov2014). Type material is stored in the Saint-Petersburg State University Mineralogical Museum, University Emb. 7/9, St. Petersburg 199034, Russia, under catalogue number 1/19696.
Occurrence and association
The holotype material was collected in the fumarole located on the west side of the micrograben of the Second scoria cone of the Northern Breakthrough of the Great Tolbachik fissure eruption that occurred in 1975–1976 on the Tolbachik volcano, Kamchatka, Far-Eastern Region, Russia (55°41'N, 160°14'E, 1200 m asl). The specimen was found in 2000. The temperature of the surface of the fumarole was ~200°C. The mineral is a product of exhalative fumarolic activity. The host rock for the mineral is a basalt scoria. The sample was placed into a glass tube, which was closed and waxed and kept under ambient conditions. When the glass tube was opened, no changes of sample were noted.
The graben at the place of the sample collecting was filled with the pyroclastic material (see Fig. 1). The presence of the gas stream was manifested by relatively high temperatures (up to 200°C), newly formed mineral phases and the development of an oxidation process.

Fig. 1. A view of the fissure of the micrograben on the west side of the Second Cinder cone of Great Tolbachik fissure eruption (photo taken in 1981).
The new mineral in the form of blue cryptocrystalline crusts enveloped fine pyroclastic material. Petrovite occurs in association with tiny black scales of tenorite in close intergrowths with transparent green particles of euchlorine, NaKCu3O(SO4)3 and white particles of dobrovolskiite, Na4Ca(SO4)3. The change of eruptive pyroclastic material was characterised by the wide development of oxidation processes accompanied by the formation of a fine hematite phase. The mineral is relatively stable, which allowed its detailed investigation. The crystallisation of the mineral most likely happened by direct precipitation from volcanic gases.
General appearance and physical properties
The mineral occurs as blue and green globular aggregates of tabular crystals up to 0.2 mm in maximal dimension, generally with gaseous inclusions (Figs 2 and 3). The streak is white and the lustre is vitreous. Fracture is conchoidal. No cleavage or parting was observed. The Mohs’ hardness is 4. The calculated density based on the empirical formula and single-crystal unit-cell parameters is 2.80 g/cm3.
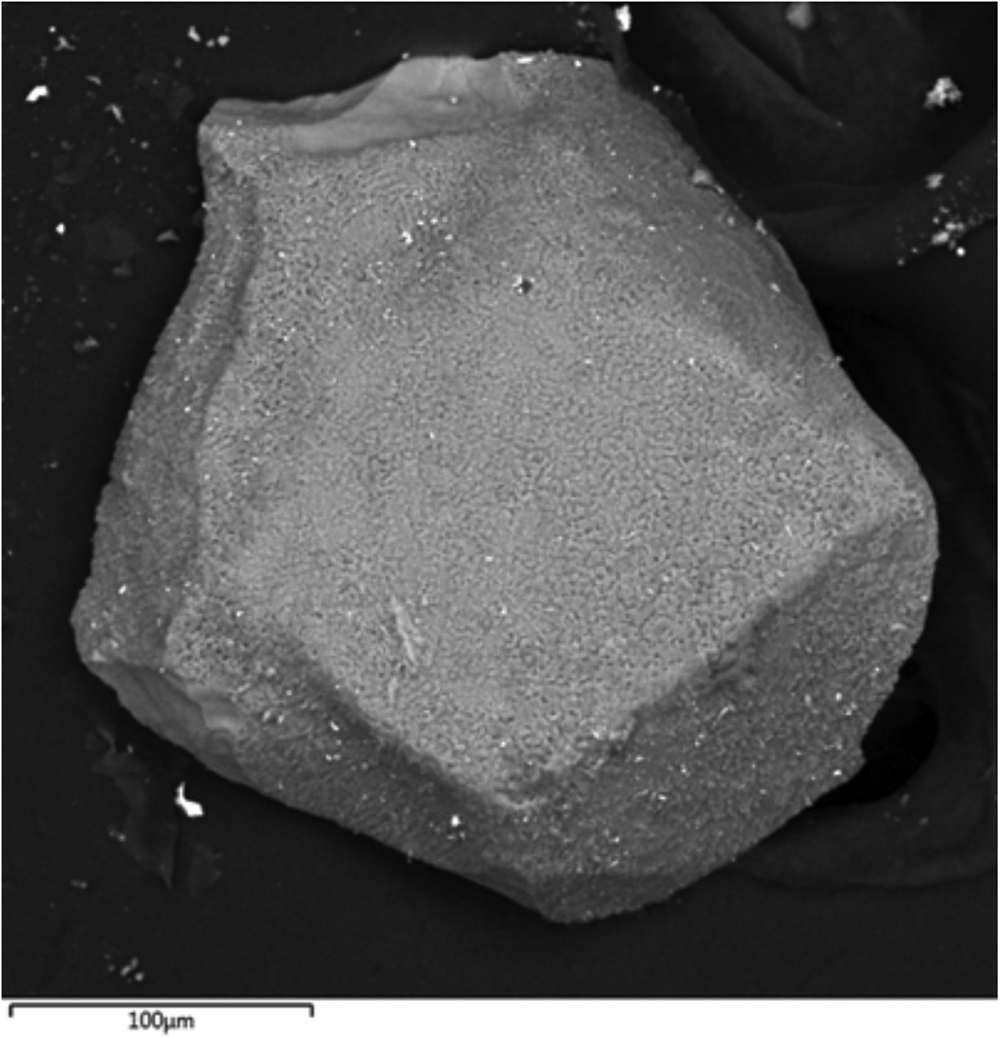
Fig. 2. Scanning electron microscopy image of an individual grain of petrovite. Part of the petrovite sample stored in the Mineralogical Museum of St. Petersburg State University (1/19696).

Fig. 3. Blue cryptocrystalline crusts of petrovite enveloped by fine pyroclastic material. Parts of the petrovite sample stored in the Mineralogical Museum of St. Petersburg State University (1/19696).
The mineral is optically biaxial (+), with α = 1.498(3), βcalc = 1.500, γ = 1.516(3) and 2V = 20(10) (λ = 589 nm). No dispersion or pleochroism was observed. The Gladstone–Dale compatibility index (Mandarino, Reference Mandarino1981) based on empirical formula and unit-cell parameters from powder X-ray diffraction data is calculated as (1 – K P/K C) = –0.0007 (superior).
Chemical composition
The chemical composition of petrovite was studied using a TESCAN “Vega3” electron microprobe equipped with an Oxford Instruments X-max 50 silicon drift energy-dispersive spectroscopy system, operated at 20 kV and 700 pA, with a beam size of 220 nm. Analytical results are given in Table 1. The data processing was done using Aztec software and an X-MAX-80 mm2 detector (Institute of Volcanology and Seismology, Petropavlovsk-Kamchatsky, Russia). The empirical formula calculated on the basis of 32 O atoms per formula unit is Na9.38Ca0.82K0.20Cu1.84Mg0.18S8.12O32. The formula that takes into account the site assignment is Na3(Na,K)(Cu,Mg)(Na,Ca,Mg)(Na,□)(SO4)4. The idealised formula derived from the combination of chemical and structural studies (see below) is Na10Cu2Ca(SO4)8.
Table 1. Chemical composition of petrovite (wt.%).

S.D. – standard deviation
Powder X-ray diffraction
The powder X-ray diffraction data were collected using a Rigaku R-AXIS RAPID II (Gandolfi mode with CoKα) and handled using the domestic software (Britvin et al., Reference Britvin, Dolivo-Dobrovolsky and Krzhizhanovskaya2017). Petrovite is monoclinic, P21/c, a = 12.6346(8), b = 9.0760(6), c = 12.7560(8) Å, β = 110.75(9)°, V = 1385.1(3) Å3 and Z = 2.
The measured and calculated powder-diffraction data are given in Table 2. The seven strongest lines are [d, Å (I, %) (hkl)]: 7.21(27)(110); 6.25(38)(102); 4.47(31)(212); 3.95(21)(30$\bar{2}$); 3.85(17)(121); 3.70(36)(202); and 3.65(34)(22
$\bar{1}$).
Table 2. Powder X-ray diffraction data (d in Å) for petrovite.

The seven strongest lines are given in bold
Single-crystal X-ray diffraction
The single-crystal X-ray diffraction data were collected using a Bruker Smart APEX II diffractometer equipped with a CCD detector using MoKα radiation. A hemisphere of three-dimensional data was collected using a frame width of 0.5° in ω, with 60 s used to acquire each frame. The data were corrected for Lorentz, polarisation and background effects using the Bruker program APEX. A semi-empirical absorption-correction based on the intensities of equivalent reflections was applied in the SADABS program.
The crystal structure of petrovite was solved by charge flipping and refined on the basis of 2470 unique observed reflections using the JANA2006 program suite (Petříček et al., Reference Petříček, Dusek and Palatinus2006). The observed isotropic displacement parameter of the Na6 site was too high (0.327 Å2), so the site was split into two mutually exclusive sites with reasonable displacement parameters. Crystallographic data and refinement parameters, atomic coordinates and isotropic displacement parameters, atomic anisotropic displacement parameters and selected interatomic distances are summarised in Tables 3–5. The crystallographic information files have been deposited with the Principal Editor of Mineralogical Magazine and are available as Supplementary material (see below).
Table 3. Crystal data, data collection information and structure refinement details for petrovite.
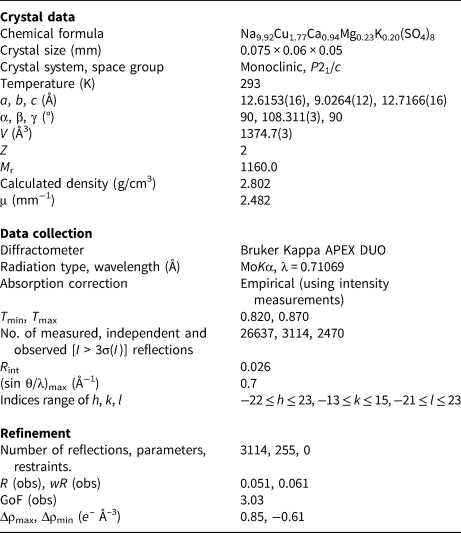
Table 4. Atomic coordinates and displacement parameters (Å2) for petrovite.
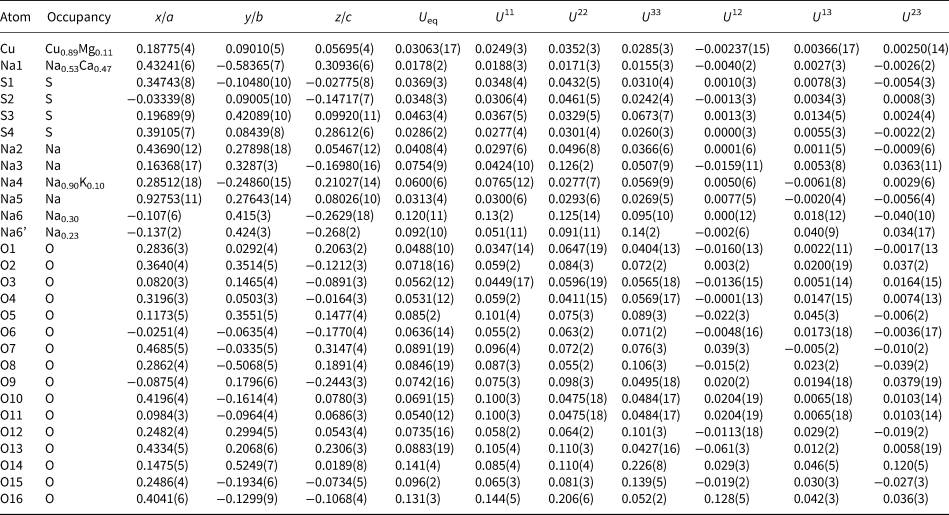
Table 5. Selected interatomic distances (Å) for petrovite.

Crystal structure
The crystal structure of petrovite belongs to a new structure type. It contains four symmetrically independent SO4 tetrahedra with the average <S–O> bond lengths in the range 1.45–1.47 Å, in general agreement with the average value of 1.475 Å given for sulfate minerals (Hawthorne et al., Reference Hawthorne, Krivovichev, Burns, Alpers, Jambor and Nordstrom2000). The short S–O bonds in several tetrahedra can be explained by libration effects, typical for the crystal structures with statistical or dynamical disorder.
There are six Na and one Cu sites with different occupancies. The Cu atom forms five Cu–O bonds in the range 1.980–2.180 Å and two long bonds ≈ 2.9 Å resulting in the formation of the CuO7 polyhedra with [5+2] coordination of Cu (Fig. 4a). The Cu site is predominantly occupied by Cu (89%) with the admixture of Mg (11%). This type of coordination geometry of Cu2+ cations is rather unusual, but was described previously in the crystal structures of saranchinaite, Na2Cu(SO4)2 (Siidra et al., Reference Siidra, Lukina, Nazarchuk, Depmeier, Bubnova, Agakhanov, Avdontseva, Filatov and Kovrugin2018; Kovrugin et al., Reference Kovrugin, Nekrasova, Siidra, Mentre, Masquelier, Stefanovich and Colmont2019), the high pressure phase (II) CuGeO3 (Yoshiasa et al., Reference Yoshiasa, Yagyu, Ito, Yamanaka and Nagai2000) and Cu3(Hbtc)(btc)(bpy)2 (Nadeem et al., Reference Nadeem, Bhadbhade, Bircher and Stride2010). After saranchinaite, petrovite is the second mineral with heptacoordinated Cu2+.
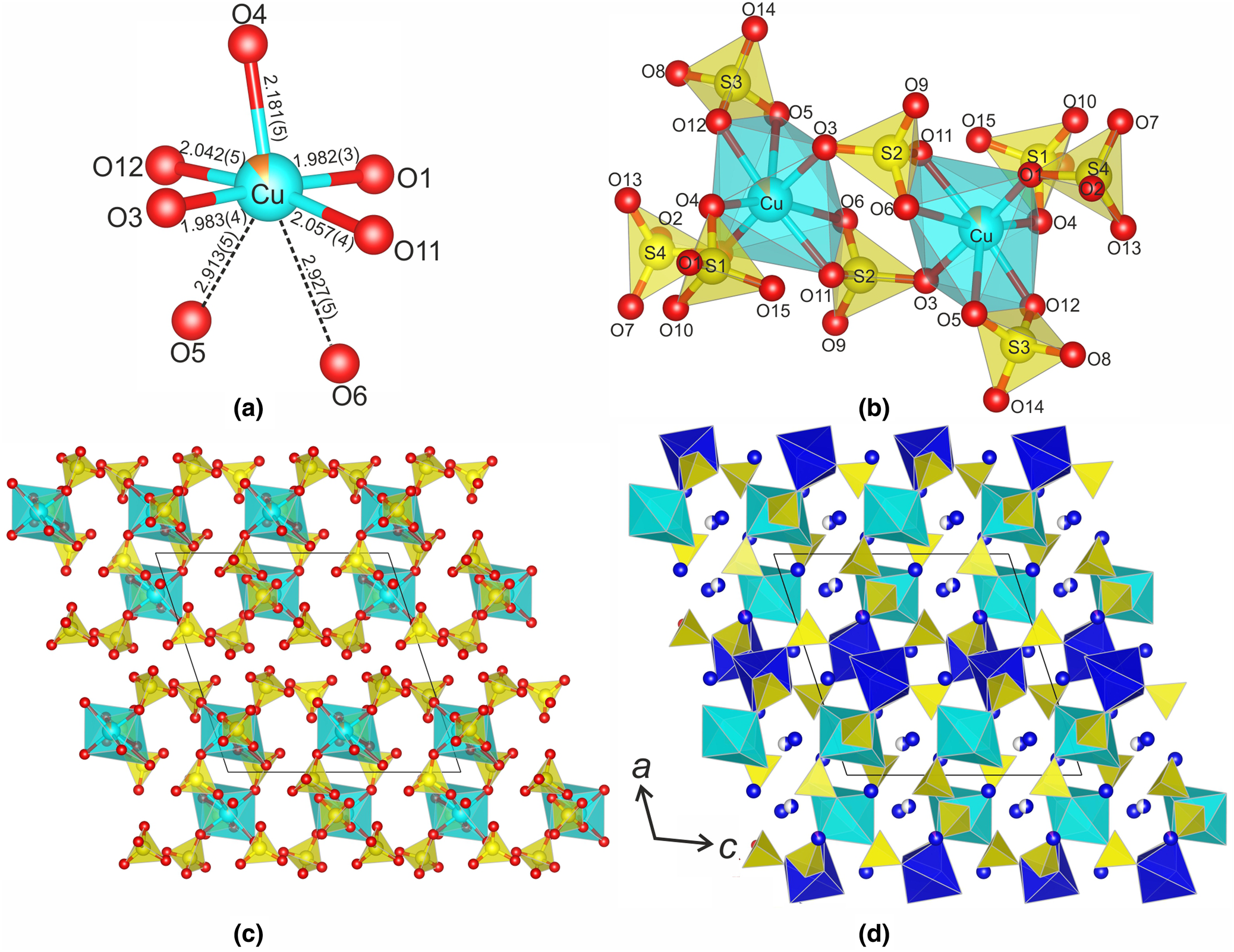
Fig. 4. The crystal structure of petrovite: (a) CuO7 polyhedron; (b) the [Cu2(SO4)8]12– cluster; (c) arrangement of the Cu2(SO4)8 clusters in the structure; and (d) three-dimensional framework of the crystal structure.
The CuO7 polyhedra share corners with SO4 tetrahedra to form isolated [Cu2(SO4)8]12− anionic clusters shown in Fig. 4b, which are the fundamental building blocks for the structure of petrovite. The arrangement of the clusters in shown in Fig. 4c. The four Na sites, Na2, Na3, Na4 and Na5, are fully occupied by Na. In the Na1 site, the essential amount of Ca is present (ca. 47%), which explains its high bond-valence sum (see below). The Na6 site is only partially occupied (53%). The Na1, Na2, Na3, Na5, Na6 and Na6’ atoms are surrounded by six O atoms each, forming distorted octahedra with the average bond lengths equal to 2.29, 2.37, 2.57, 2.35, 2.49 and 2.48 Å, respectively. The Na4 site is coordinated by seven O atoms with the Na4−O bond lengths in the range 2.347–2.993 Å.
The petrovite structure can also be described as a three-dimensional framework, taking into account the essential occupancy of the Na1 site by Ca2+ cations. In this description, the [Cu2(SO4)8]12− clusters are linked by Na1O6 octahedra, forming a porous three-dimensional framework (Fig. 4d) with cavities occupied by Na+ cations.
The bond-valence calculations were performed using empirical parameters taken from Brown and Altermatt (Reference Brown and Altermatt1985). The results are presented in Table 6. The high bond-valence sums of the S sites and the Na1 site are significantly overbonded, whereas the bond valence sum for Na3 is 0.61 valence units (vu). The deviation of the bond-valence sums from the expected values may be explained by the structural and positional disorder as well as the high ionic mobility of the Na atoms (see below).
Table 6. Bond-valence analysis (vu = valence units) for petrovite.
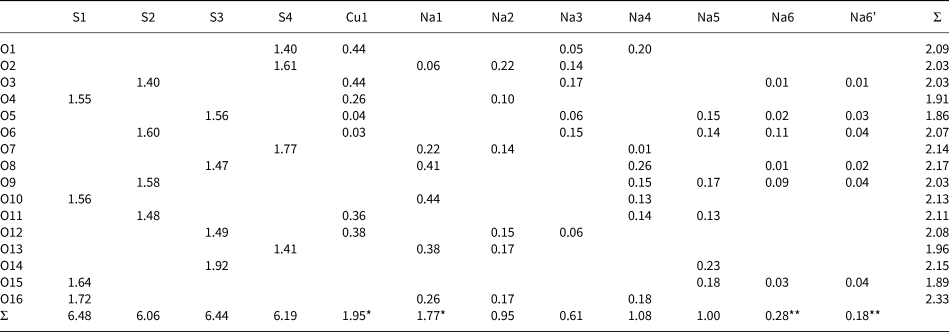
* The site is occupied by two cations
** The site is partially occupied
Discussion
Taking into account the essential admixture of Ca in the Na1 site, and the low occupancy of the Na6 site, the crystal chemical formula of petrovite can be written as Na6−2xCaxCu(SO4)4. For petrovite, x ≈ 0.5, which results in the idealised formula Na10CaCu2(SO4)8. However, at least theoretically, the x parameter may vary, resulting in different mineral species. For instance, for x = 0, 1, 1.5 and 2, the idealised formulae are Na6Cu(SO4)4, Na4CaCu(SO4)4, Na6Ca3Cu2(SO4)8 and Na2Ca2Cu(SO4)4, respectively. The x = 2 member of the series, Na2Ca2Cu(SO4)4, is chemically similar to itelmenite, Na2Mg2Cu(SO4)4, assuming the Mg-for-Ca replacement. However, the structure types of petrovite and itelmenite are different.
From the chemical point of view, petrovite demonstrates chemical and structural similarities to saranchinaite, Na2Cu(SO4)2. The three-dimensional [Cu(SO4)2]2− anionic framework in saranchinaite is formed by corner-sharing SO4 tetrahedra and CuOn polyhedra with the Na+ cations located in the cavities. In the crystal structure of petrovite, there are [Cu2(SO4)8]12− clusters of corner-sharing Cu and S polyhedra.
The chemical formula of petrovite can be obtained from that of saranchinaite through the hypothetical reaction:

The reaction implies the incorporation of the ionic CaSO4 and Na2(SO4) components into the largely covalent [Cu(SO4)2]2− anionic framework with the reduction of the dimensionality of the Cu sulfate polymeric network from three (in saranchinaite) to one (in petrovite). The crystal−chemical behaviour of this kind is known as the dimensional reduction, which has been described previously in oxides and oxysalts (Alekseev et al., Reference Alekseev, Krivovichev, Armbruster, Depmeier, Suleimanov, Chuprunov and Golubev2007; Krivovichev, Reference Krivovichev2009; Kovrugin et al., Reference Kovrugin, Gurzhiy and Krivovichev2012).
It is of interest that both petrovite and saranchinaite contain unusual Cu2+O7 polyhedra. According to Siidra et al. (Reference Siidra, Lukina, Nazarchuk, Depmeier, Bubnova, Agakhanov, Avdontseva, Filatov and Kovrugin2018), the presence of the Cu2+O7 polyhedra is one of the potential reasons for the crystallisation of saranchinaite in the non-centrosymmetric space group P21. Obviously, this kind of reasoning cannot be applied to petrovite, which both contains Cu2+O7 polyhedra and crystallises in the centrosymmetric space group P21/c.
Many chemical compounds and materials have been synthesised based on known mineral species (see, e.g. the recent work on synthetic saranchinaite by Siidra et al., Reference Siidra, Lukina, Nazarchuk, Depmeier, Bubnova, Agakhanov, Avdontseva, Filatov and Kovrugin2018). Therefore, the analysis of the functional properties of new minerals is important from the viewpoint of their material science applications. As the Cu2+/Cu3+ redox potential may provide a high operating voltage (Sun et al., Reference Sun, Rousse, Abakumov, Saubanère, Doublet, Rodríguez- Carvajal, Van Tendeloo and Tarascon2015), we have calculated the bond-valence energy landscape (BVEL) of petrovite using the BondStr software (Rodríguez-Carvajal, Reference Rodríguez-Carvajal2004). As a result, we have found that there are interconnected pathways for the Na+ migration in petrovite (Fig. 5), considering a percolation energy of 1.6 eV reported for the mobility of Na+ in polyanionic compounds (Boivin et al., Reference Boivin, Chotard, Bamine, Carlier, Serras, Palomares, Rojo, Iadecola, Dupont and Bourgeois2017; Kovrugin et al., Reference Kovrugin, Nekrasova, Siidra, Mentre, Masquelier, Stefanovich and Colmont2019). Thus, the petrovite structure type is promising as a cathode material. The theoretical capacity of petrovite based on the oversimplified formula Na6Cu(SO4)4 (x = 0) is 274.5 mAh/g, but the low Cu content decreases the theoretical capacity to ~46 mAh/g.

Fig. 5. The crystal structure of petrovite with the scheme of Na migration pathways. Migration pathways are calculated in BondStr software (Rodríguez-Carvajal, Reference Rodríguez-Carvajal2004).
Acknowledgements
This work was supported financially by the Russian Found of Basic Research, grant no. 18-29-12106. Technical support by the SPbSU X-ray Diffraction Centre is gratefully acknowledged.
Supplementary material
To view supplementary material for this article, please visit https://doi.org/10.1180/mgm.2020.53