Introduction
Depression is a serious chronic psychiatric disorder, and is one of the leading causes of disability worldwide in terms of total years lived with disability (GBD 2017 Disease and Injury Incidence and Prevalence Collaborators, 2018). More than one-third of depressed patients fail to achieve remission despite receiving adequate treatment, and are therefore considered to have treatment-resistant depression (TRD) (Fava & Davidson, Reference Fava and Davidson1996). TRD may be defined as an absence of symptomatic remission after two successive trials of antidepressants of different pharmacological classes. The trials must be managed and monitored in terms of dosage and duration (at least 80% of treatment must be taken over the considered period) (Holtzmann et al., Reference Holtzmann, Richieri, Saba, Allaïli, Bation, Moliere and Haffen2016; Rush, Thase, & Dubé, Reference Rush, Thase and Dubé2003).
It is widely accepted that electroconvulsive therapy (ECT) is an effective treatment for TRD (Jaffe, Reference Jaffe2002; Lisanby, Reference Lisanby2007). However, its response remains partial: patients who are resistant to antidepressants have reported a remission rate of 48% (Heijnen, Birkenhäger, Wierdsma, & van den Broek, Reference Heijnen, Birkenhäger, Wierdsma and van den Broek2010), and response rates ranging from 39% to 85% (Haq, Sitzmann, Goldman, Maixner, & Mickey, Reference Haq, Sitzmann, Goldman, Maixner and Mickey2015). Some drawbacks to ECT include the onset and time course of its antidepressant effect, as well as patients' intolerance to it. The mechanism of action of ECT has only been partially understood (Fosse & Read, Reference Fosse and Read2013; Jiang, Wang, & Li, Reference Jiang, Wang and Li2016). The most robust data points to an increase in neurotrophin (including brain-derived neurotrophic factor and vascular endothelial growth factor) levels as well as in the volumes of both the hippocampus and the amygdala (Camilleri et al., Reference Camilleri, Hoffstaedter, Zavorotny, Zöllner, Wolf, Thomann and Nickl-Jockschat2020; Jiang et al., Reference Jiang, Wang and Li2016). ECT has also been shown to have an effect on the glutamate/gamma-aminobutyric acid (GABA) system (Luo et al., Reference Luo, Min, Wei, Li, Dong and Liu2011; Sartorius, Mahlstedt, Vollmayr, Henn, & Ende, Reference Sartorius, Mahlstedt, Vollmayr, Henn and Ende2007).
Repetitive transcranial magnetic stimulation (rTMS) is an alternative neuromodulation technique to treat TRD patients. Its efficacy has been proven to have a moderate effect on depression (Schutter, Reference Schutter2009; Slotema, Blom, Hoek, & Sommer, Reference Slotema, Blom, Hoek and Sommer2010). Compared to ECT, rTMS produces more transient and milder side effects, is more cost effective, and is more easily accepted by patients (Fitzgibbon et al., Reference Fitzgibbon, Plett, Chan, Hancock-Howard, Coyte and Blumberger2020). However, ECT has been shown to be a more effective treatment for TRD than rTMS, especially when treating major depression with psychotic features (Slotema et al., Reference Slotema, Blom, Hoek and Sommer2010).
rTMS and ECT could share some mechanisms of action. These mechanisms include changes in: neuronal excitability (Hoppenrath, Härtig, & Funke, Reference Hoppenrath, Härtig and Funke2016; Sun et al., Reference Sun, Wang, Wang, Zhang, Yamamoto, Sugai and Kato2011; Tang et al., Reference Tang, Hong, Boddington, Garrett, Etherington, Reynolds and Rodger2016); cortical glutamate/GABA neurotransmissions (Cirillo et al., Reference Cirillo, Di Pino, Capone, Ranieri, Florio, Todisco and Di Lazzaro2017; Croarkin et al., Reference Croarkin, Nakonezny, Wall, Murphy, Sampson, Frye and Port2016); gene expression (Grehl et al., Reference Grehl, Viola, Fuller-Carter, Carter, Dunlop, Hool and Rodger2015; Ikeda, Kurosawa, Uchikawa, Kitayama, & Nukina, Reference Ikeda, Kurosawa, Uchikawa, Kitayama and Nukina2005); neurotrophic protein expression necessary for neuroplasticity (Gersner, Kravetz, Feil, Pell, & Zangen, Reference Gersner, Kravetz, Feil, Pell and Zangen2011; Makowiecki, Harvey, Sherrard, & Rodger, Reference Makowiecki, Harvey, Sherrard and Rodger2014; Rodger, Mo, Wilks, Dunlop, & Sherrard, Reference Rodger, Mo, Wilks, Dunlop and Sherrard2012); activity within brain regions beyond the induced electrical field (Aydin-Abidin, Trippe, Funke, Eysel, & Benali, Reference Aydin-Abidin, Trippe, Funke, Eysel and Benali2008; Seewoo, Feindel, Etherington, & Rodger, Reference Seewoo, Feindel, Etherington and Rodger2018; Seewoo, Feindel, Etherington, & Rodger, Reference Seewoo, Feindel, Etherington and Rodger2019); and non-neuronal cells that may contribute to neuroplasticity events (Clarke et al., Reference Clarke, Penrose, Penstone, Fuller-Carter, Hool, Harvey and Bates2017; Cullen et al., Reference Cullen, Senesi, Tang, Clutterbuck, Auderset, O'Rourke and Young2019). In particular, GABA levels are generally lower in depressed patients (Sanacora et al., Reference Sanacora, Mason, Rothman, Behar, Hyder, Petroff and Krystal1999), and then increase after treatment with selective serotonin reuptake inhibitors (Sanacora, Mason, Rothman, & Krystal, Reference Sanacora, Mason, Rothman and Krystal2002) and ECT (Sanacora et al., Reference Sanacora, Mason, Rothman, Hyder, Ciarcia, Ostroff and Krystal2003). In addition, rTMS induces an increase in the cortical silent period (CSP), which is a supposed marker of GABA-B receptor-mediated inhibition (Daskalakis et al., Reference Daskalakis, Möller, Christensen, Fitzgerald, Gunraj and Chen2006; Romeo et al., Reference Romeo, Gilio, Pedace, Ozkaynak, Inghilleri, Manfredi and Berardelli2000). In depressed patients who responded to high rTMS frequency (20 Hz) over the left dorsolateral prefrontal cortex (DLPFC), both CSP and intracortical inhibition (ICI) increased, the latter being correlated with clinical improvement (Bajbouj et al., Reference Bajbouj, Brakemeier, Schubert, Lang, Neu, Schindowski and Danker-Hopfe2005). ICI could be linked to the involvement of intracortical inhibitory circuits mediated by the GABAergic A (GABA-A) system (Daskalakis et al., Reference Daskalakis, Möller, Christensen, Fitzgerald, Gunraj and Chen2006).
Should the clinical efficacy of rTMS and ECT indeed be based on common mechanisms, especially on the GABAergic system, their combination may be even more effective. In one case report, two TRD patients who were treated with this combination reported improvements (Rothärmel, Quesada, Compere, & Guillin, Reference Rothärmel, Quesada, Compere and Guillin2017). Before receiving ECT, these patients received several high-frequency rTMS (HF-rTMS) sessions over the left DLPFC [20 Hz, 90% of the motor threshold (MT), 20 trains of 2 s with an intertrain interval of 60 s]. Their subsequent clinical improvement seemed to occur earlier than usual. Indeed, for one patient, improvements were observed after only three ECT sessions. Seizure duration was also longer, and the electrical charges were lower than expected, reflecting a lower seizure threshold. The lowering of the seizure threshold through rTMS was reported in another case report (Albrecht et al., Reference Albrecht, Buday, Mareš, Kališová, Raboch and Anders2019) and a randomized double-blind study (Buday et al., Reference Buday, Albrecht, Podgorná, Mareš, Le, Čapek and Anders2020). These two studies administered only one rTMS session before each ECT session compared to the several sessions administered by Rothärmel et al. (Reference Rothärmel, Quesada, Compere and Guillin2017). The sample size of these data is too small to draw any conclusions.
The main objective of this randomized double-blind study was to evaluate the efficacy of priming rTMS sessions before ECT as treatment for TRD patients. The evaluation took place after five ECT sessions; a standard for measuring the onset of clinical response (Kellner et al., Reference Kellner, Knapp, Husain, Rasmussen, Sampson, Cullum and Petrides2010). Our hypothesis was that the active rTMS group would show more significant clinical improvement at an earlier stage than the sham group. The secondary objective was to study the impact of this combination on side effects such as cognitive disorders, and on ECT treatment parameters such as seizure duration, seizure threshold, the probability of having postictal electro-encephalography (EEG) suppression, and drug dosage (curare and anesthetics). We hypothesized that, at five ECT sessions, there would be no difference between either group regarding side effects, cognitive effects and most ECT parameters. However, we also hypothesized that postictal EEG suppression or ‘burst suppression’, which is induced by the activation of GABA-A receptors (Jäntti & Sloan, Reference Jäntti and Sloan2008) would be more frequent in the active group than in the sham group. A significant relationship between the degree of postictal suppression and likelihood of clinical improvement had been observed (Suppes et al., Reference Suppes, Webb, Carmody, Gordon, Gutierrez-Esteinou, Hudson and Pope1996).
Materials and methods
Participants
Patients met inclusion criteria if they: (i) were 18–70 years old; (ii) had a current DSM-IV diagnosis of major depressive episode, based on the Mini International Neuropsychiatric Interview (MINI) with a 21-item Hamilton Depression Rating Scale (HDRS) score of at least 15; (iii) had failed to achieve a clinical response to three separate trials of antidepressants from different classes (at least one of which was a tricyclic) at a sufficient dose, for at least 6 weeks, according to stage III of Thase and Rush TRD classification criteria (Thase & Rush, Reference Thase and Rush1997); (iv) had received a stable dose of antidepressants and/or mood stabilizers for at least 15 days before the protocol onset; (v) had sufficient knowledge of the French language; and (vi) gave their informed written consent.
Patients with at least one of the following criteria were excluded from the study if they: (i) had already received rTMS treatment in their lifetime; (ii) had already received ECT treatment for the current episode; (iii) had any contraindication for rTMS, ECT, or Magnetic Resonance Imaging (MRI); (iv) had any contraindication for propofol (or one of its excipients) or curare; (v) were suffering from a neurological disorder (dementia, brain damage); (vi) had a history of seizures; (vii) suffered from severe hearing disorders; (viii) were pregnant or lactating women, or women of childbearing age who did not use contraception; and (ix) were already participating, or had participated within the 30 days prior to the inclusion visit, in an interventional clinical trial.
This research was performed in accordance with the Declaration of Helsinki and was approved by the local ethic review committee (CPP Nord-Ouest I) on 28 January 2016. The study was registered on the website http://clinicaltrials.gov/ (NCT02830399).
Study design and overview
This was a prospective, multicenter, randomized, double-blind study. Participants were recruited in four French clinical centers that are specialized in neurostimulation treatment: (i) Le Rouvray Hospital in Sotteville-lès-Rouen; (ii) Caen University Hospital; (iii) Henri Laborit Hospital in Poitiers and (iv) Pitié-Salpêtrière University Hospital in Paris. This study was conducted from July 2016 to October 2019, and it comprised of several phases, as illustrated in Fig. 1.

Fig. 1. Schedule of enrollment, interventions, and assessments of the study.
ECT, electroconvulsive therapy; rTMS, repetitive transcranial magnetic stimulation; MRI, Magnetic Resonance Imaging; MINI, Mini-Mental State Examination; HDRS, Hamilton Rating Scale for Depression; UKU, modified version of the UKU side effects rating scale; SSMQ, Squire Subjective Memory Questionnaire; CFQ, Cognitive Failures Questionnaire.
Initially, patients underwent screening over a period of 1 month, to ensure that they were medically stable and that they satisfied the recruitment criteria. During this period, an oral assessment by a stomatologist, an electrocardiogram, a standard biological assessment, a preanesthetic consultation and a brain MRI (for neuronavigation) were performed. The only cases where benzodiazepines and antiepileptics were not stopped at least 15 days before randomization were cases where they could not be gradually discontinued. For patients who had an addictive comorbidity, most had been hospitalized before starting ECT, and therefore had refrained from alcohol consumption for at least 2 weeks (time to start ECT).
Following the screening period, patients were randomly assigned to active or sham rTMS, with an allocation ratio of 1:1 (Day1). Blocked randomization with stratification by clinical center was used (block size = 6). The Department of Biostatistics at Rouen University Hospital generated the allocation sequence, which was uploaded on the Ennov Clinical website (https://fr.ennov.com/gestions-essais-cliniques/). Five active or sham rTMS sessions occurred from Day1 to Day4 in the first week of treatment. Then, all patients received five sessions of active ECT over 3 weeks (from Day5 to Day18). Assessments occurred before treatment (Month−1 and/or Day1), during treatment (Day4: after rTMS, Day12: after three ECT sessions) and after treatment (Day19: after five ECT sessions). Patients, neuropsychologists and psychiatrists assessing the patients were blinded to treatment allocation. Only the nurse administering the rTMS sessions was aware of the allocated arm.
Treatment protocol
rTMS
rTMS was administered using a MagPro X100 with a figure-8 coil (Tonica ElektroniK; MagVenture, Denmark). The TMS coil targeted the left DLPFC through Syneika (www.syneika.fr) or Visor (Visor, ANT, Enschede, The Netherlands) neuronavigation systems. The left DLPFC was determined as the middle part of the middle frontal gyrus, at the intersection between Brodmann areas 9 and 46 (Nauczyciel et al., Reference Nauczyciel, Hellier, Morandi, Blestel, Drapier, Ferre and Millet2011). A sham coil that was identical to the real coil in terms of acoustic sensation and visual impact, although it didn't produce any magnetic field, was used for the placebo group. The sham coil did not, however, generate the cutaneous sensation of magnetic stimulation. The resting motor threshold (RMT) was defined as the lowest intensity required to evoke motor-evoked potential (MEP) with a peak-to-peak amplitude of ⩾50 μV in at least 5 out of 10 consecutive trials in the relaxed first dorsal interosseous muscle of the right hand (Groppa et al., Reference Groppa, Oliviero, Eisen, Quartarone, Cohen, Mall and Siebner2012). The parameters of the rTMS were defined in accordance with French guidelines on the use of rTMS (Lefaucheur et al., Reference Lefaucheur, André-Obadia, Poulet, Devanne, Haffen, Londero and Garcia-Larrea2011): 20 two-second trains of 20 Hz, 90% RMT, 60 s inter-train intervals, 800 pulses/session. Each rTMS session lasted 20 min. In total, five rTMS sessions were scheduled over four days (Day 1 to Day 4 of the first week of treatment). At least 2 h separated two rTMS sessions when they occurred the same day. The number of sessions and the parameters were deliberately suboptimal so as not to induce a specific antidepressant effect, while allowing a priming effect to occur. A single 20 Hz rTMS session with 90% of RMT was indeed enough to induce a significant increase in the duration of the CSP (Daskalakis et al., Reference Daskalakis, Möller, Christensen, Fitzgerald, Gunraj and Chen2006).
Electroconvulsive therapy (ECT)
ECT was delivered using either a MECTA Spectrum 5000Q (MECTA Corp, Tualatin, Oregon) or a Thymatron System IV device (Somatics Inc., Lake Bluff, Illinois). Patients received five bitemporal ultrabrief-pulse ECT sessions, with one session during the first week (D 5) followed by two sessions a week. The seizure threshold was determined by an individual titration method during the first ECT session (Sackeim et al., Reference Sackeim, Long, Luber, Moeller, Prohovnik, Devanand and Nobler1994). The initial stimulation was administered at twice the seizure threshold, according to a review by Loo, Schweitzer, and Pratt (Reference Loo, Schweitzer and Pratt2006). The seizure threshold may increase during the course of treatment. If the stimulation was ineffective (if the seizure duration was less than 20 s, without producing postictal suppression), another stimulation with a one-step increase of 50% was delivered. The general anesthetic propofol (2,6-di-isopropylphenol) was administered at doses of 1–2 mg/kg, with suxamethonium chloride (curare) at doses of 0.3–0.8 mg/kg for short-term paralysis. Despite its anticonvulsant effect, propofol is not thought to interfere with the efficacy of ECT if used at low dose (Kronsell et al., Reference Kronsell, Nordenskjöld, Bell, Amin, Mittendorfer-Rutz and Tiger2021) and is not thought to affect the quality of the seizure when compared to methohexital (Geretsegger, Rochowanski, Kartnig, & Unterrainer, Reference Geretsegger, Rochowanski, Kartnig and Unterrainer1998). An ECT was recorded as being effective if, when measured using an electroencephalogram, it induced either a seizure lasting more than 20 s or a seizure lasting 15–20 s followed by postictal suppression. In center 1 (Sotteville-lès-Rouen) all the patients' EEG were assessed by two different blind investigators, with particular attention paid to seizure duration and postictal suppression.
Assessments
Measuring the primary outcome
For the primary outcome, the Hamilton Depression Rating Scale (HDRS) was used to compare the relative improvement of depressive symptoms ((Post-treatment HDRS score minus Pre-treatment HDRS score)/ Pre-treatment HDRS score) between arms after five ECT sessions (Hamilton, Reference Hamilton1980). In addition, the percentage of patients who achieved a 50% or greater reduction in their HDRS scores (responder rate) were compared between groups. As illustrated in Fig. 1, the HDRS was administered at baseline (Day 1), at the end of rTMS sessions (Day 4), after three ECT sessions (Day 12), and after five ECT sessions (Day 19).
Measuring secondary outcomes
Global cognitive functioning was measured using the Mini-Mental State Examination (MMSE; Folstein, Folstein, and McHugh, Reference Folstein, Folstein and McHugh1975). Verbal and visual memory performances were assessed using the RL/RI-16 (Grober, Buschke, Crystal, Bang, & Dresner, Reference Grober, Buschke, Crystal, Bang and Dresner1988) and the Doors test (Baddeley, Wilson, & Kopelman, Reference Baddeley, Wilson and Kopelman1995) respectively. Attention was measured with the D2 test of attention (Brickenkamp, Reference Brickenkamp1966). The Rey–Osterrieth complex figure test assessed visuospatial/constructional ability, planning and organization (Osterrieth, Reference Osterrieth1944). Cognitive tests were administered before treatment (Month−1) and after five ECT sessions (Day 19, Fig. 1).
Cognitive functioning was subjectively assessed via self-administered questionnaires, such as the Squire Subjective Memory Questionnaire (SSMQ) (Squire, Wetzel, & Slater, Reference Squire, Wetzel and Slater1979) and the Cognitive Failures Questionnaire (CFQ) (Broadbent, Cooper, FitzGerald, & Parkes, Reference Broadbent, Cooper, FitzGerald and Parkes1982).
Side effects were evaluated with an adapted version of the UKU scale (Lingjaerde, Ahlfors, Bech, Dencker, & Elgen, Reference Lingjaerde, Ahlfors, Bech, Dencker and Elgen1987). SSMQ, CFQ and UKU scales were administered at the end of rTMS sessions (Day 4) and after five ECT sessions (Day 19, Fig. 1).
The Mini-International Neuropsychiatric Interview (MINI) (Sheehan et al., Reference Sheehan, Lecrubier, Sheehan, Amorim, Janavs, Weiller and Dunbar1998) was used to confirm the diagnosis of unipolar or bipolar depression according to DSM-IV criteria, and to assess other psychiatric comorbidities, as well as the risk of suicide.
Sample size
Initial calculations in the study protocol were based on a clinical study about ECT (Kellner et al., Reference Kellner, Knapp, Husain, Rasmussen, Sampson, Cullum and Petrides2010) and on the hypothesis that adding rTMS would lead to an additional 25% clinical improvement compared to patients on ECT only, which was not supported by clinical data. These calculations yielded 84 patients overall (42 patients per arm) but were revised for pragmatic reasons given the difficulty of recruiting patients. New calculations were based on a pilot open-label study (Quesada, Reference Quesada2015). In that study, the mean [standard deviation (s.d.)] HDRS relative improvements were 59.3% (24.0%) among 11 depressive patients who were treated by rTMS and ECT, and 27.1% (12.2%) among 16 depressive patients only treated by ECT. To guard against overoptimism from the pilot study, revised sample size calculations were based on a smaller difference, i.e. 80% of the observed difference in the pilot study, 59.0% v. 33.5%, with 24.0% common s.d. To obtain 90% power for Mann–Whitne' nonparametric test at the two-sided 0.05 level with respect to this difference, 24 patients per arm were needed, hence 48 patients overall. To account for an expected 5% drop-out rate, the sample size was increased to 26 patients per arm (52 patients overall). When the sample size was recalculated, 56 patients had already been included, thus recruitment was halted at that point.
Statistical analysis
Our analysis of the primary outcome (relative improvement of the Hamilton Rating Scale for Depression 21-items score between Day1 and Day19) relied on the intention-to-treat (ITT) principle and Mann–Whitney' non parametric test. In case of a missing primary outcome, simple imputation would be performed with the worst-case scenario: (i) for patients in the active rTMS group, the missing outcome would be replaced by the lowest improvement observed among all patients (active and sham arms) and (ii) for patients in the sham rTMS group, the missing outcome would be replaced by the greatest improvement observed among all patients. Two sensitivity analyses were performed: one based on the last observation carried forward (LOCF) approach in case of a missing primary outcome, the other a per protocol approach, which included only treatment completers with no missing primary outcome. Multiple linear regression was used to adjust treatment comparisons for each center.
The same approach to analysis was applied to secondary quantitative outcomes. For categorical outcomes, comparisons between both treatment arms relied on Pearson' χ2 test or Fisher' exact test as appropriate. Size effects were estimated with eta squared (η 2) (Fritz, Morris, & Richler, Reference Fritz, Morris and Richler2012) or odds ratio. A Bonferroni correction was applied to multiple exploratory comparisons. The analyses were conducted using SPSS, version 27 (IBM, Armonk, NY) and RStudio (1.3.1093).
Results
Among the 58 screened patients, 56 patients were included and randomized in the four participating centers: Le Rouvray Hospital (n = 44); Caen University Hospital (n = 5); Henri Laborit Hospital, Poitiers (n = 6); and Pitié-Salpêtrière University Hospital (n = 1). Figures 1 and 2 (flow-chart) depict the enrollment, randomization, follow-up and data analysis.
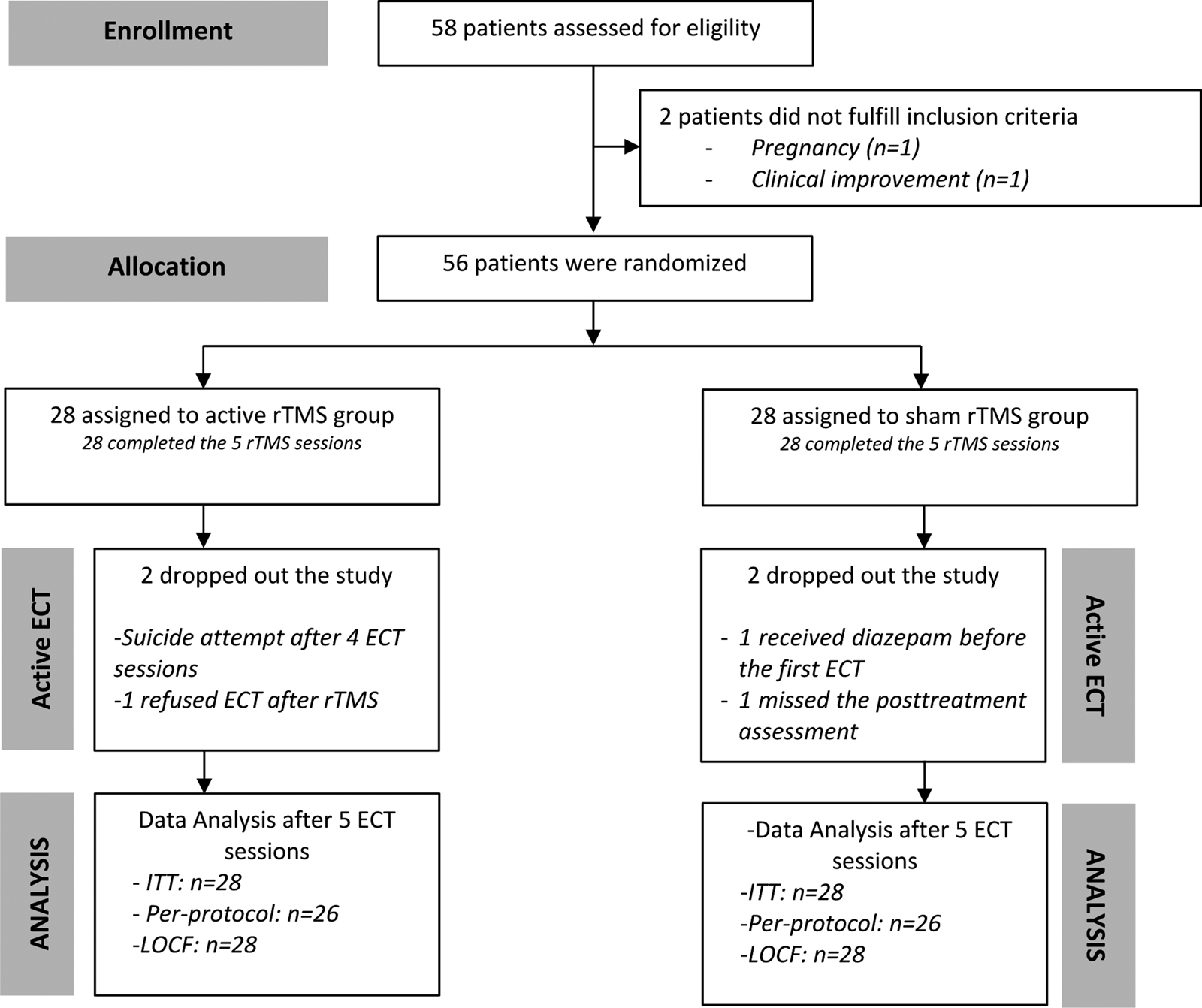
Fig. 2. Flow chart of the study. ECT, electroconvulsive therapy; rTMS, repetitive Transcranial Magnetic Stimulation; ITT, intention-to-treat analysis; LOCF, Last Observation Carried Forward method.
Study sample characteristics
There were no significant differences between groups in baseline demographic and clinical characteristics (Table 1). In particular, there was no difference between groups in the HDRS score at the baseline (p = 0.16, Table 2). In the whole sample, the mean (s.d.) baseline HDRS was 27.23 (5.24), 28.6% of patients suffered from bipolar disorder, and 80.4% had a comorbid anxiety disorder. The psychiatric comorbidities were: panic disorder (37.5%), social phobia (32.1%), agoraphobia (28.6%), generalized anxiety (23.21%), alcohol dependence (12.5%), post-traumatic stress disorder (7.1%), agoraphobia with panic disorder and bulimia nervosa (5.4% each), obsessive compulsive disorder, substance dependence and psychotic disorder (3.6% each) and alcohol and substance abuse (1.8% each). The suicidal risk was: absent (16.1%), low (30.4%), moderate (8.9%), and high (44.6%).
Table 1. Clinical and demographic characteristics of the sample

1 p values are results of Mann–Whitney tests.
2 p values are results of χ2 statistics.
Table 2. HDRS scores, relative improvement (Day1–Day19/Day1) and responder rate between groups

LOCF, Last Observation Carried Forward; Relative improvement = (Day1–Day19/Day1).
Results in bold are significant at the threshold of 0.05.
1 p values are results of Mann-Whitney tests.
2 p values are results of χ2 statistics.
The patients had resisted on average to 6.79 (2.46) different treatments at the time of inclusion and all were at stage three or above on the Thase and Rush classification. There was no significant difference between the groups regarding treatment history (p = 0.803). All the patients were taking at least one psychotropic drug during the study. There was no difference between the groups regarding use of benzodiazepines (active group: 14.8%; sham group: 22.2%; p = 0.484), lithium (active group: 42.3%; sham group: 44.4%; p = 0.875), antipsychotic drugs (active group: 59.3%; sham group: 48.1%; p = 0.413), antidepressant drugs (active group: 66.7%; sham group: 85.2%; p = 0.111), or antiepileptic drugs (active group: 11.1%; sham group: 11.1%; p = 1.00).
Clinical efficacy
Efficacy of five rTMS sessions before ECT
After five ECT sessions, the ITT analysis indicated that there was a greater relative improvement, expressed as percentage improvement in HDRS, in the active compared to the sham rTMS group [median (min; max) in the active group = 46.1% (−55.6; 81.5)], median [min; max] in the sham group = 26.6% (−4.5; 81.5)), p = 0.04, η 2 = 0.076). The LOCF and per protocol analysis led to the same result (Table 2). This significant result was unchanged when adjusted for each clinical center. Among the patients who completed the study (per-protocol analysis, n = 52), the mean (s.d.) relative improvement was 43.4% (28.6%) in the active rTMS group and 25.4% (17.2%) in the sham rTMS group.
The proportion of patients who clinically responded to treatment (i.e. achieving a 50% or greater reduction in their HDRS scores) was higher in the active group compared to the sham group, irrespective of the type of analysis (Table 2). Among the patients who completed the study, 46.2% of patients responded to the treatment in the active group compared to 7.7% in the sham group.
However, after only three ECT sessions (Day12), the relative improvement was not significantly different between the two groups, with ITT (p = 0.422; η 2 = 0.012), per-protocol (p = 0.186; η 2 = 0.032) and LOCF (p = 0.201; η 2 = 0.029) analyses. Likewise, there was no difference between groups just after the five active/sham rTMS sessions (Day4) for ITT (p = 0.278; η 2 = 0.02), per-protocol (p = 0.182; η 2 = 0.03) and LOCF (p = 0.1611; η 2 = 0.035) analyses. The changes in HDRS scores are illustrated in Fig. 3.

Fig. 3. Evolution of HDRS scores during treatment. Means and standard deviations are plotted; ECT, electroconvulsive therapy; HDRS, Hamilton Rating Scale for Depression.
Given that psychotropic drugs can affect rTMS response, the effect of pharmacological treatment was assessed in the active rTMS group. The relative improvement was not different depending on benzodiazepines (p = 1.00), lithium (p = 0.223), typical and atypical antipsychotic drugs (p = 0.188), or antidepressant drugs (p = 0.156). The effect of antiepileptic drugs was not assessed due to the small number of patients receiving this kind of treatment (n = 3).
Cognitive effects
The evolution [after ECT (D 19) minus baseline (M−1)] between groups did not show any significant differences on any cognitive tests assessing attention, memory and visuospatial abilities (online Supplementary Table S1).
Similarly, the SSMQ scores were not significantly different between groups: after rTMS sessions [D 4 scores: meanactive_group(s.d.) = −0.81 (9.79) and meansham_group(s.d.) = −1.52 (6.25); p = 0.230] and after five ECT sessions [D 19 scores: meanactive_group (s.d.) = −3.73 (11.54) and meansham_group (s.d.) = −4.24 (12.31); p = 0.392].
Regarding the CFQ scores, there was no difference immediately after active/sham rTMS [D 4 scores: meanactive_group (s.d.) = 12.67 (22.71) and meansham_group (s.d.) = 8.07 (14.21); p = 0.726]. However, after ECT, cognitive complaints were higher in the sham rTMS group compared to the active rTMS group [D 19 scores: meanactive_group (s.d.) = 5.54 (11.93) and meansham_group (s.d.) = 15.64 (18.97); p = 0.009; η 2 = 0.140].
Tolerability
Side effects
Regarding side effects, as assessed by the UKU scale, there was no significant difference between the groups after rTMS (Day 4) and after five ECT sessions (Day 19) (online Supplementary Table S2).
Serious adverse events
There was one serious adverse event among the 56 patients who were included in the study. One patient in the active rTMS group attempted suicide after four ECT sessions. Suffering from severe late-onset depression, his clinical state improved rapidly after the second ECT session. He was then discharged from hospital, inducing an anxiety attack which led him to make the suicide attempt. The patient presented no sequelae.
ECT parameters
Exploratory analyses were conducted in the coordinating center (Le Rouvray Hospital, n = 44). Anesthetic drug doses (propofol and curare), the amount of ECT administered per session, the electrical charge during the first and fifth sessions, and the probability of having postictal EEG suppression were compared between groups (online Supplementary Table S3). During the fifth ECT session, the electrical charge differed significantly between groups (p = 0.026; η 2 = 0.117): medians [min; max] were 154 [100; 461] in the active group and 252 [115; 907] in the sham group.
Effect of ECT on cognition
This exploratory analysis compared cognitive performance before and after ECT in the whole sample (i.e. the two groups were pooled) with Bonferroni correction for multiple comparisons (online Supplementary Table S4). After ECT (Day 19), we observed a significant increase in the D2 test of attention scores (p = 0.001, η 2 = 0.297), reflecting an improvement in patient concentration. Moreover, delayed free recall scores and percentage of patients with pathological delayed total recall on the RL/RI-16 (assessing memory performances) significantly worsened (respectively, p < 0.001, η 2 = 0.495 and p = 0.002, OR 8.00), reflecting a decrease in verbal memory storage capacity. Regarding MMSE, 40% of patients had a decreased score after ECT [mean (s.d.) = 10.28% (9.18)], 44% had an increased score [mean (s.d.) = 13.28% (9.45)], while 16% reported no change.
Patients' follow-up
An open-label clinical data collection was carried out at the end of the study in the main clinical center (Le Rouvray Hospital, Rouen). Based on patients' clinical records (n = 42, 16 patients by group), the total number of ECT sessions were: meanactive_group (s.d.) = 18 (4.1) and meansham_group(s.d.) = 16.2 (5.6). After eight ECT sessions, 75% of the patients (n = 12/16) responded to the treatment in the active group compared to 62.5% in the sham group (n = 10/16). After 12 ECT sessions, 75% of the patients (n = 12/16) responded to the treatment in the active group compared to 76.9% in the sham group (n = 10/13). No patient died during the study.
Discussion
To our knowledge, this is the first randomized, double-blind, sham-controlled study assessing the clinical efficacy of five priming rTMS sessions before ECT in patients with TRD. After five ECT sessions, the clinical improvement in the group that received active rTMS sessions was significantly higher (70% more) than in the sham rTMS group, with a responder rate between three (ITT) and six times higher (per-protocol and LOCF analyses). This significant difference was achieved not only in per protocol analysis but also in ITT analysis, which included all randomized patients by replacing missing data with the worst-case imputation. This improvement in depressive symptoms was clinically meaningful, with treatment effect sizes ranging from medium (η 2 = 0.076 in the ITT analysis) to large (η 2 = 0.208 in the per-protocol analysis and η 2 = 0.193 in the LOCF analysis). In our stimulation paradigm, rTMS was not chosen to induce its own antidepressant effect (no difference between groups after five rTMS sessions, at Day 4), but to obtain a ‘priming’ effect before ECT (Bortolomasi et al., Reference Bortolomasi, Minelli, Fuggetta, Perini, Comencini, Fiaschi and Manganotti2007; Quesada, Reference Quesada2015), thus making ECT effective more quickly (46.2% of patients already responding after only five ECT sessions), which is essential because of the high risk of suicide (84%) among patients. However, it is difficult to compare our rates of improvement with those of other studies in the literature because the vast majority of them reported responder rates after more than five ECT sessions (Haq et al., Reference Haq, Sitzmann, Goldman, Maixner and Mickey2015).
Only one other team has used HF-rTMS in combination with ECT in patients with TRD (Albrecht et al., Reference Albrecht, Buday, Mareš, Kališová, Raboch and Anders2019; Buday et al., Reference Buday, Albrecht, Podgorná, Mareš, Le, Čapek and Anders2020). While we used rTMS as an intensive treatment before starting ECT, they administered one HF-rTMS session over the left DLPFC in a time interval of 30–80 min before each ECT session (Buday et al., Reference Buday, Albrecht, Podgorná, Mareš, Le, Čapek and Anders2020). Their rTMS/ECT paradigm showed a significant reduction in seizure threshold of about a third at the first ECT session, but did not improve patients' symptomatology, which was assessed at the end of hospitalization. In the present study, the electrical charge of the fifth ECT session was lower in the active group than in the sham group. As suggested by Buday et al. (Reference Buday, Albrecht, Podgorná, Mareš, Le, Čapek and Anders2020), this decrease in electrical charge might be due to rTMS administration, which resulted in increased cortical excitability, lowering the energy needed to induce seizures. This hypothesis should be investigated in a future study using brain activity measures.
However, unlike Buday et al.' study (2020), the difference in electrical charge did not appear from the first ECT session in the present study. This difference could be explained by the differing rTMS protocols (one rTMS session before each ECT v. five rTMS sessions before ECT). We can assume that these protocols did not induce the same effect on cortical excitability because they had different stimuli frequencies, different duration of application periods, and different total number of stimuli (Arai et al., Reference Arai, Okabe, Furubayashi, Terao, Yuasa and Ugawa2005).
In our study, rTMS/ECT combination might involve neurobiological mechanisms underlying long-term potentiation (Cirillo et al., Reference Cirillo, Di Pino, Capone, Ranieri, Florio, Todisco and Di Lazzaro2017). Contrary to our initial hypothesis, the effect of this combination might not initially involve the GABA pathway, because there was no difference between groups for postictal EEG suppression, which is linked to the GABAergic activity (Jäntti & Sloan, Reference Jäntti and Sloan2008), and we observed a decrease in seizure threshold (and therefore a probable increase in neuroexcitability) at five ECT sessions. However, the GABA hypothesis of ECT would predict GABA outpouring in the dorsal nexus, within the dorsal medial prefrontal cortex, as the locus for efficacy (Sackeim, Reference Sackeim2004). Combining the anticonvulsant and neuroplasticity hypotheses could explain how ECT works (Seymour, Reference Seymour2021). We can assume that the GABA system is involved later in the ECT treatment, but for now, we cannot explain the mechanisms underlying the priming effect of rTMS on ECT.
By reducing the stimulus and the number of ECT sessions required, the rTMS-ECT combination could also improve ECT tolerance, particularly regarding its cognitive side effects. The CFQ indeed showed a better tolerance among patients in the active group. This could be explained by the more significant mood improvement, CFQ scores correlating positively with the BDI scores (Wagle, Berrios, & Ho, Reference Wagle, Berrios and Ho1999). However, between the groups, there were no differences in side effects (UKU scale) or cognitive performances (attention, memory and visuospatial abilities). In the whole sample, concentration was significantly improved after ECT sessions, which might be related to an improvement in depression symptoms (Jaeger, Berns, Uzelac, & Davis-Conway, Reference Jaeger, Berns, Uzelac and Davis-Conway2006). On the other hand, verbal memory storage capacity decreased after ECT. This might be related to the bitemporal placement of electrodes which promotes memory alterations (Sackeim et al., Reference Sackeim, Prudic, Devanand, Kiersky, Fitzsimons, Moody and Settembrino1993; Semkovska & McLoughlin, Reference Semkovska and McLoughlin2010) or to the mechanisms underlying ECT effects (Joshi et al., Reference Joshi, Espinoza, Pirnia, Shi, Wang, Ayers and Narr2016). Indeed, ECT induces neuroplasticity in the hippocampus and amygdala. These volume increases, in relation to clinical response, were observable within 72 h of treatment initiation (Joshi et al., Reference Joshi, Espinoza, Pirnia, Shi, Wang, Ayers and Narr2016). Anterior regions of the hippocampus being associated with episodic memory, their structural modifications could explain the transient alterations in verbal episodic memory (Zammit et al., Reference Zammit, Ezzati, Zimmerman, Lipton, Lipton and Katz2017).
Despite novel results, our study has some noteworthy limitations. First, our study aimed to assess short-term efficacy of priming ECT with rTMS until the effect of ECT begins to be described (Kellner et al., Reference Kellner, Knapp, Husain, Rasmussen, Sampson, Cullum and Petrides2010), which does not allow us to conclude on its effectiveness in the long term. Clinical data collected in one of the centers at the end of the double-blind study seem to indicate that the priming effect wears off over the course of sessions. Although methodologically limited, this outcome suggests that rTMS sessions should be continued during ECT to maintain the effect. The second limitation is the lack of evaluation of the mechanisms of action underlying ECT and rTMS. The electroencephalographic criteria did not allow us to understand the mechanisms of this potentiation and further measures would be necessary, such as functional imaging. Third, the rTMS parameters could be considered as suboptimal compared to current guidelines. It would be relevant to reproduce this study with, for example, intermittent theta burst stimulation. Fourth, the choice of bitemporal electrode placement could be questioned in a future study, given the cognitive advantage in a right unilateral ECT placement described in a recent study (Su et al., Reference Su, Jia, Liang, Shi, Mellor and Xu2019). Similarly, the choice of ECT parameters such as pulse width could be studied, with findings that the efficacy of ultrabrief bilateral ECT may be reduced compared to brief pulse width ECT (Loo, Katalinic, Martin, & Schweitzer, Reference Loo, Katalinic, Martin and Schweitzer2012). Fifth, the revision of the sample size from 84 to 52 patients overall was based on findings from the study by Quesada (Reference Quesada2015) which, being a small open-label study, may have yielded an inflated effect size estimate, leading therefore to the present study being somewhat underpowered. In order to alleviate this problem, we allowed for some margin in the revised sample size calculations relative to the findings reported by Quesada (Reference Quesada2015), and were able to show a significant difference in favor of active rTMS on the main outcome. However, we cannot completely rule out the possibility of a false positive result favored by the reduced sample size. Finally, the success of blinding was not evaluated, which constitutes a substantial limitation, especially since the sham coil did not mimic the magnetic sensation. As the sensations of real rTMS have not been adequately mimicked in rTMS research, intervention allocation might not be appropriately concealed (Broadbent et al., Reference Broadbent, van den Eynde, Guillaume, Hanif, Stahl, David and Schmidt2011). However, in a meta-analysis focused on blinding success in rTMS studies for major depression, Berlim, Broadbent, and Van den Eynde (Reference Berlim, Broadbent and Van den Eynde2013) concluded that commonly used sham rTMS methods lead to acceptable levels of blinding integrity.
In summary, this is the first study to demonstrate a potentiating effect of sessions of HF-rTMS on ECT efficacy in patients with TRD. This effect consisted of a 70% improvement in depressive symptoms after five ECT sessions and a reduction of the energy needed to induce an epileptiform seizure. Furthermore, this combination was well tolerated, with less subjective cognitive complaints. Further studies are required to assess the efficacy of this combination over time and the mechanisms underlying its action.
Supplementary material
The supplementary material for this article can be found at https://doi.org/10.1017/S0033291721003810
Acknowledgements
We thank all the participants. We thank Jocelyne Halley and Valérie Falconieri for assistance in rTMS and ECT administration; Chloé Modzelewski and Frédérique Caillot for assistance in data management; and Owen Thomas for proofreading.
Financial support
This study was supported by DGOS (PHRC-Inter-Régional 2014, Number 14-082) and ‘Fondation Pierre Deniker’. These organizations had no role in the design and conduct of the study.
Conflict of interest
All authors report no biomedical financial interests or potential conflicts of interest.