Introduction
The Greek island of Rhodes is situated in the southeastern part of the Aegean Sea, an embayment of the Mediterranean Sea (Fig. 1A). Because of substantial Late Pleistocene and Holocene vertical tectonic movements, the Pleistocene, mostly characterized by marine deposits, largely crop out (Fig. 1B) along its eastern coast (e.g., Mutti et al., Reference Mutti, Orombelli and Pozzi1970; Hanken et al., Reference Hanken, Bromley and Miller1996; van Hinsbergen et al., Reference van Hinsbergen, Krijgsman, Langereis, Cornée, Duermeijer and van Vugt2007; Cornée et al., Reference Cornée, Quillévéré, Moissette, Fietzke, López-Otálvaro, Melinte-Dobrinescu and Philippon2019). Especially during the last two decades, intensive research has provided a great amount of information about the sedimentology, stratigraphy, and paleontology of these richly fossiliferous strata.
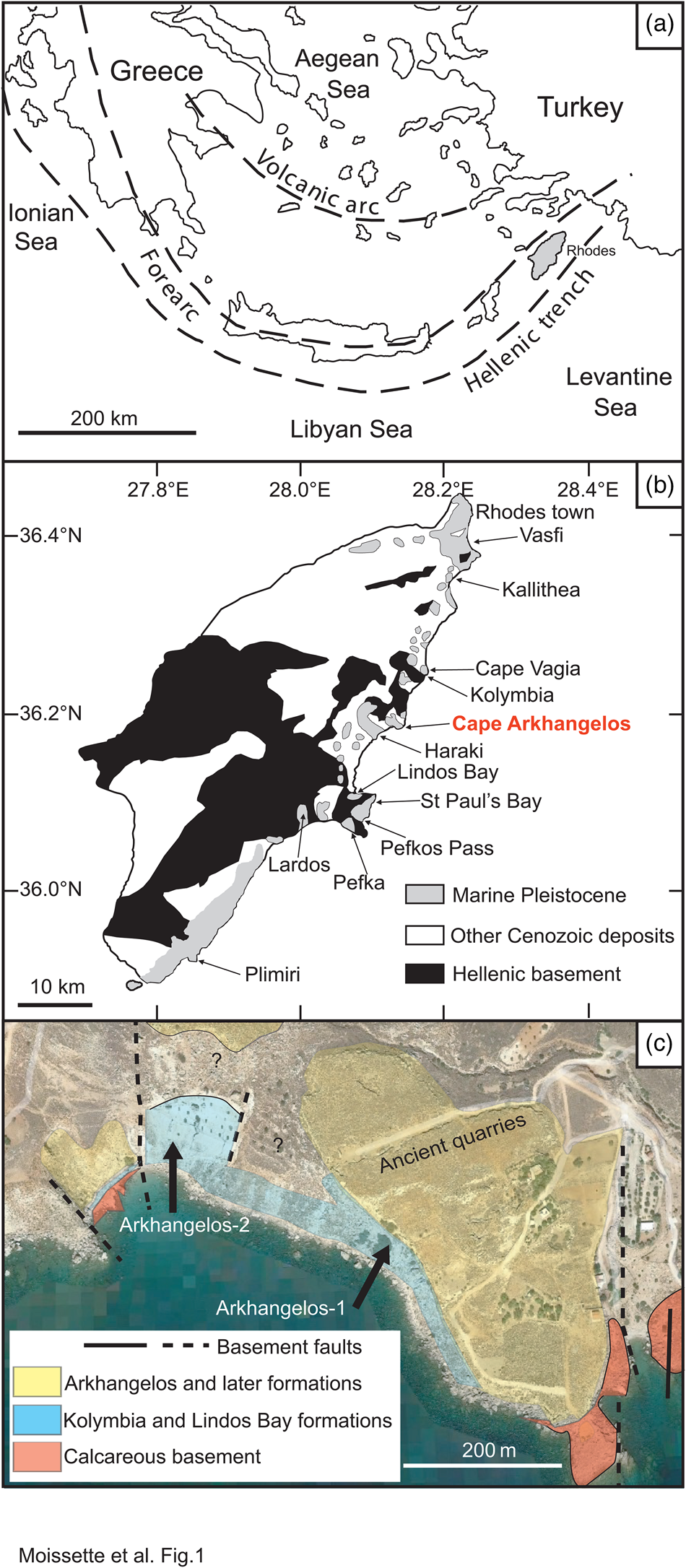
Figure 1. Simplified maps of the study area. (a) Eastern Mediterranean with simplified tectonic setting (modified from Meulenkamp et al., Reference Meulenkamp, De Mulder and Van De Weerd1972; Quillévéré et al., Reference Quillévéré, Cornée, Moissette, López-Otálvaro, van Baak, Münch, Melinte-Dobrinescu and Krijgsman2016). (b) Geology of Rhodes (modified from Mutti et al., Reference Mutti, Orombelli and Pozzi1970; Quillévéré et al., Reference Quillévéré, Cornée, Moissette, López-Otálvaro, van Baak, Münch, Melinte-Dobrinescu and Krijgsman2016). (c) Schematic geological map of the study area (modified from Google Earth).
The Pleistocene sediments of Rhodes were emplaced in steep-flanked paleovalleys of the deformed Mesozoic basement of the Aegean forearc and consequently comprise a variety of facies (e.g., Meulenkamp et al., Reference Meulenkamp, De Mulder and Van De Weerd1972; Hanken et al., Reference Hanken, Bromley and Miller1996; Titschack et al., Reference Titschack, Joseph, Fietzke, Freiwald and Bromley2013). They were deposited at water depths between the sea surface (and even in rivers and lagoons) and the upper bathyal. A revised stratigraphic subdivision of the Pleistocene has been proposed by Titschack et al. (Reference Titschack, Joseph, Fietzke, Freiwald and Bromley2013), Quillévéré et al. (Reference Quillévéré, Cornée, Moissette, López-Otálvaro, van Baak, Münch, Melinte-Dobrinescu and Krijgsman2016), and Cornée et al. (Reference Cornée, Quillévéré, Moissette, Fietzke, López-Otálvaro, Melinte-Dobrinescu and Philippon2019). Ten sedimentary formations (included in five synthems) have thus been defined. Among these formations, the deep-water Lindos Bay Formation, within the Rhodes Synthem, offers a unique sedimentary archive available inland to study the rich upper bathyal foraminiferal and invertebrate communities and to reconstruct the faunal and paleoenvironmental changes that occurred in the eastern Mediterranean during the Early Pleistocene.
Cape Arkhangelos is located in the central-eastern region of the island, about 30 km south of the capital city (Fig. 1B). Two new sections situated very near the seashore have been found and studied in this region (Fig. 1C), because they exhibit facies and fossil contents that appear atypical at Rhodes. The hemipelagic sedimentary deposits of the Lindos Bay Formation usually exhibit predominant but rare upper bathyal organisms, deposited in mostly calm conditions at as much as several hundred meters water depths within homogenous clays (Moissette and Spjeldnaes, Reference Moissette and Spjeldnaes1995; Hanken et al., Reference Hanken, Bromley and Miller1996; Thomsen et al., Reference Thomsen, Rasmussen and Hastrup2001; Milker et al., Reference Milker, Weinkauf, Titschack, Freiwald, Krüger, Jorissen and Schmiedl2017; Quillévéré et al., Reference Quillévéré, Nouailhat, Joannin, Cornée, Moissette, Lécuyer, Fourel, Agiadi, Koskeridou and Escarguel2019). In the Cape Arkhangelos area, however, deposits of the Lindos Bay Formation are characterized by rich invertebrate faunas often associated with coralline algae and gravels (even pebbles) that are always encrusted by calcareous epibionts and bioeroded.
In this paper, we study for the first time these atypical deep-sea deposits enriched in transported allochthonous shallow marine components by deciphering their biostratigraphy and by analyzing their paleontological content. We further provide a paleoenvironmental reconstruction that mostly relies on foraminifera and invertebrate faunas (mollusks, bryozoans, and to a lesser degree brachiopods). The emphasis is on deep-water communities since they can be considered as autochthonous, contrary to transported elements (benthic faunas and gravels).
Geological and stratigraphical background
The island of Rhodes, which structurally constitutes the easternmost part of the Aegean sedimentary forearc (Fig. 1A), is made up of nappes that have been accreted as a result of the subduction of Africa beneath Eurasia. Because of the increasing curvature of the forearc along the African/Eurasian plate boundary, the nappes have undergone severe counterclockwise rotations, stretching, faulting, and multiple tilting and vertical motions since the Pliocene (Duermeijer et al., Reference Duermeijer, Nyst, Meijer, Langereis and Spakman2000; ten Veen and Kleinspehn, Reference ten Veen and Kleinspehn2002; van Hinsbergen et al., Reference van Hinsbergen, Krijgsman, Langereis, Cornée, Duermeijer and van Vugt2007; Cornée et al., Reference Cornée, Quillévéré, Moissette, Fietzke, López-Otálvaro, Melinte-Dobrinescu and Philippon2019). In addition, the concomitant opening of the >4000 m deep Rhodes Basin just east of the island (Woodside et al., Reference Woodside, Mascle, Huguen and Volkonskaia2000; Hall et al., Reference Hall, Aksu, Yaltırak and Winsor2009) triggered rapid drowning episodes. Altogether, these tectonics resulted in deposition of Pleistocene marine sediments that have been uplifted and are now patchily exposed inland along the eastern coast of Rhodes (Fig. 1B). There, the Pleistocene marine sediments rest upon a deformed and deeply eroded, mainly calcareous Jurassic to Paleocene basement (Mutti et al., Reference Mutti, Orombelli and Pozzi1970; Meulenkamp et al., Reference Meulenkamp, De Mulder and Van De Weerd1972; Lekkas et al., Reference Lekkas, Danamos, Skourtsos and Sakellariou2001). This basement was widely faulted prior to the Pleistocene, generating fault-bounded paleovalleys interpreted as grabens or micro-basins that affected the nature and patchy distribution of the marine Pleistocene deposits (e.g., Hanken et al., Reference Hanken, Bromley and Miller1996; Cornée et al., Reference Cornée, Quillévéré, Moissette, Fietzke, López-Otálvaro, Melinte-Dobrinescu and Philippon2019).
The most severe tectonically driven transgressive–regressive cycle that led to deposition and then uplift of marine sediments now exposed onshore occurred during the Early and Middle Pleistocene. The resulting sedimentary succession of this transgressive–regressive cycle has been qualified as the Rhodes Synthem (Hanken et al., Reference Hanken, Bromley and Miller1996; Titschack et al., Reference Titschack, Joseph, Fietzke, Freiwald and Bromley2013; Cornée et al., Reference Cornée, Quillévéré, Moissette, Fietzke, López-Otálvaro, Melinte-Dobrinescu and Philippon2019). Deposition of the Rhodes Synthem (~420 m maximum thickness) started diachronously during the Early Pleistocene (late Gelasian) between ca. 2.0 Ma and ca. 1.9 Ma (Cornée et al., Reference Cornée, Münch, Quillévéré, Moissette, Vasiliev, Krijgsman, Verati and Lécuyer2006b; Moissette et al., Reference Moissette, Koskeridou, Drinia and Cornée2016; Quillévéré et al., Reference Quillévéré, Cornée, Moissette, López-Otálvaro, van Baak, Münch, Melinte-Dobrinescu and Krijgsman2016) with the littoral to lower-offshore transgressive bioclastic clayey limestones of the Kolymbia Formation (Moissette and Spjeldnaes, Reference Moissette and Spjeldnaes1995; Steinthorsdottir et al., Reference Steinthorsdottir, Lidgard and Hakansson2006). Deposition of the Rhodes Synthem ended during the Middle Pleistocene (Chibanian), ca. 0.46 Ma ago (Cornée et al., Reference Cornée, Quillévéré, Moissette, Fietzke, López-Otálvaro, Melinte-Dobrinescu and Philippon2019), with the regressive red-algal/bryozoan limestones and siliciclastic shallow water deposits of the Cape Arkhangelos Formation, which indicate a major tectonic uplift of Rhodes (van Hinsbergen et al., Reference van Hinsbergen, Krijgsman, Langereis, Cornée, Duermeijer and van Vugt2007).
Between the Kolymbia and the Cape Arkhangelos formations, the calcareous to silty hemipelagic blue-gray clays of the Lindos Bay Formation (sometimes associated with deep-sea coral boundstones of the Saint Paul's Bay Formation) constitute the most pronounced tectonic drowning of Rhodes (Hanken et al., Reference Hanken, Bromley and Miller1996; van Hinsbergen et al., Reference van Hinsbergen, Krijgsman, Langereis, Cornée, Duermeijer and van Vugt2007). Deposition of the Lindos Bay Formation occurred at upper bathyal depths between ~200 and ~600 m during the Early and Middle Pleistocene with maximum flooding recorded during the Calabrian between ca. 1.9 Ma and ca. 1.0 Ma (Moissette and Spjeldnaes, Reference Moissette and Spjeldnaes1995; Rasmussen and Thomsen, Reference Rasmussen, Thomsen, Rasmussen, Hastrup and Thomsen2005; van Hinsbergen et al., Reference van Hinsbergen, Krijgsman, Langereis, Cornée, Duermeijer and van Vugt2007; Quillévéré et al., Reference Quillévéré, Cornée, Moissette, López-Otálvaro, van Baak, Münch, Melinte-Dobrinescu and Krijgsman2016, Reference Quillévéré, Nouailhat, Joannin, Cornée, Moissette, Lécuyer, Fourel, Agiadi, Koskeridou and Escarguel2019; Milker et al., Reference Milker, Weinkauf, Titschack, Freiwald, Krüger, Jorissen and Schmiedl2017; Agiadi et al., Reference Agiadi, Girone, Koskeridou, Moissette, Cornée and Quillévéré2018; Cornée et al., Reference Cornée, Quillévéré, Moissette, Fietzke, López-Otálvaro, Melinte-Dobrinescu and Philippon2019). The Lindos Bay Formation clays are predominantly massive and homogenous. However, they often appear bioturbated and sometimes exhibit laminated layers that have been interpreted as shallower extensions of the eastern Mediterranean deep-sea sapropels (Rasmussen and Thomsen, Reference Rasmussen, Thomsen, Rasmussen, Hastrup and Thomsen2005; Quillévéré et al., Reference Quillévéré, Nouailhat, Joannin, Cornée, Moissette, Lécuyer, Fourel, Agiadi, Koskeridou and Escarguel2019).
Exposures of the Lindos Bay Formation along the eastern coast of Rhodes have been shown to be highly diachronous from section to section, because of deposition in independent steep and irregular, inherited micro-basins developed at different altitudes (Hanken et al., Reference Hanken, Bromley and Miller1996; Titschack et al., Reference Titschack, Joseph, Fietzke, Freiwald and Bromley2013; Quillévéré et al., Reference Quillévéré, Cornée, Moissette, López-Otálvaro, van Baak, Münch, Melinte-Dobrinescu and Krijgsman2016). Nevertheless, the Lindos Bay Formation has long constituted a key target to resolve the chronostratigraphy of the Lower and Middle Pleistocene of Rhodes. Indeed, the hemipelagic nature of its deposits allowed research on calcareous plankton biostratigraphy, cyclostratigraphy, and magnetostratigraphy of several sections along the eastern coast of the island (Fig. 1B), notably from north to south at Vasfi, Kallithea, Cape Vagia, Kolymbia, Haraki, Lindos Bay, Pefka, Lardos, and Plimiri (Løvlie et al., Reference Løvlie, Støle and Spjeldnaes1989; Thomsen et al., Reference Thomsen, Rasmussen and Hastrup2001; Cornée et al., Reference Cornée, Moissette, Joannin, Suc, Quillévéré, Krijgsman and Hilgen2006a, b; Titschack et al., Reference Titschack, Joseph, Fietzke, Freiwald and Bromley2013; Quillévéré et al., Reference Quillévéré, Cornée, Moissette, López-Otálvaro, van Baak, Münch, Melinte-Dobrinescu and Krijgsman2016, 2019; Milker et al., Reference Milker, Weinkauf, Titschack, Freiwald, Krüger, Jorissen and Schmiedl2017, 2019; Eichner et al., Reference Eichner, Schmiedl, Titschack, Triantaphyllou, Andersen, Forster and Milker2024; Porz et al., Reference Porz, Zuschin, Strotz, Koskeridou, Simoens, Lukic, Thivaiou, Quillévéré and Agiadi2024). The chronostratigraphy of these deposits has also benefited from radioisotope dating of (1) a volcaniclastic layer cropping out in its lower part at Kolymbia, Haraki, Pefkos Pass, and Lindos Bay (Cornée et al., Reference Cornée, Münch, Quillévéré, Moissette, Vasiliev, Krijgsman, Verati and Lécuyer2006b; Quillévéré et al., Reference Quillévéré, Cornée, Moissette, López-Otálvaro, van Baak, Münch, Melinte-Dobrinescu and Krijgsman2016); and (2) specimens of the deep-water coral Desmophyllum pertusum collected in its upper part at Lardos (Titschack et al., Reference Titschack, Joseph, Fietzke, Freiwald and Bromley2013; Agiadi et al., Reference Agiadi, Quillévéré, Nawrot, Sommeville, Coll, Koskeridou, Fietzke and Zuschin2023).
Material and methods
Sampling and sample processing
During field work in 2019, two sections, termed Arkhangelos-1 (Fig. 2; 36°11'17.31“N, 28°7'28.07”E; 29 m thickness) and Arkhangelos-2 (Fig. 3; 36°11'12.94“N, 28°7'37.15”E; 22 m thickness) were logged and sampled after the outcrop surfaces were refreshed. Thirty-five samples (about 500–1000 g each) were collected, with an average sample spacing of 1.30 m (18 and 17 samples from sections Arkhangelos-1 and Arkhangelos-2, respectively). For micro- and macrofossil analyses, each bulk sample was first oven-dried, then soaked in a dilute solution of 3% hydrogen peroxide (H2O2) for about 24 h, and finally washed over a column of sieves (1, 0.5, 0.25, and 0.125 mm). The residues were oven-dried and stored in plastic containers.
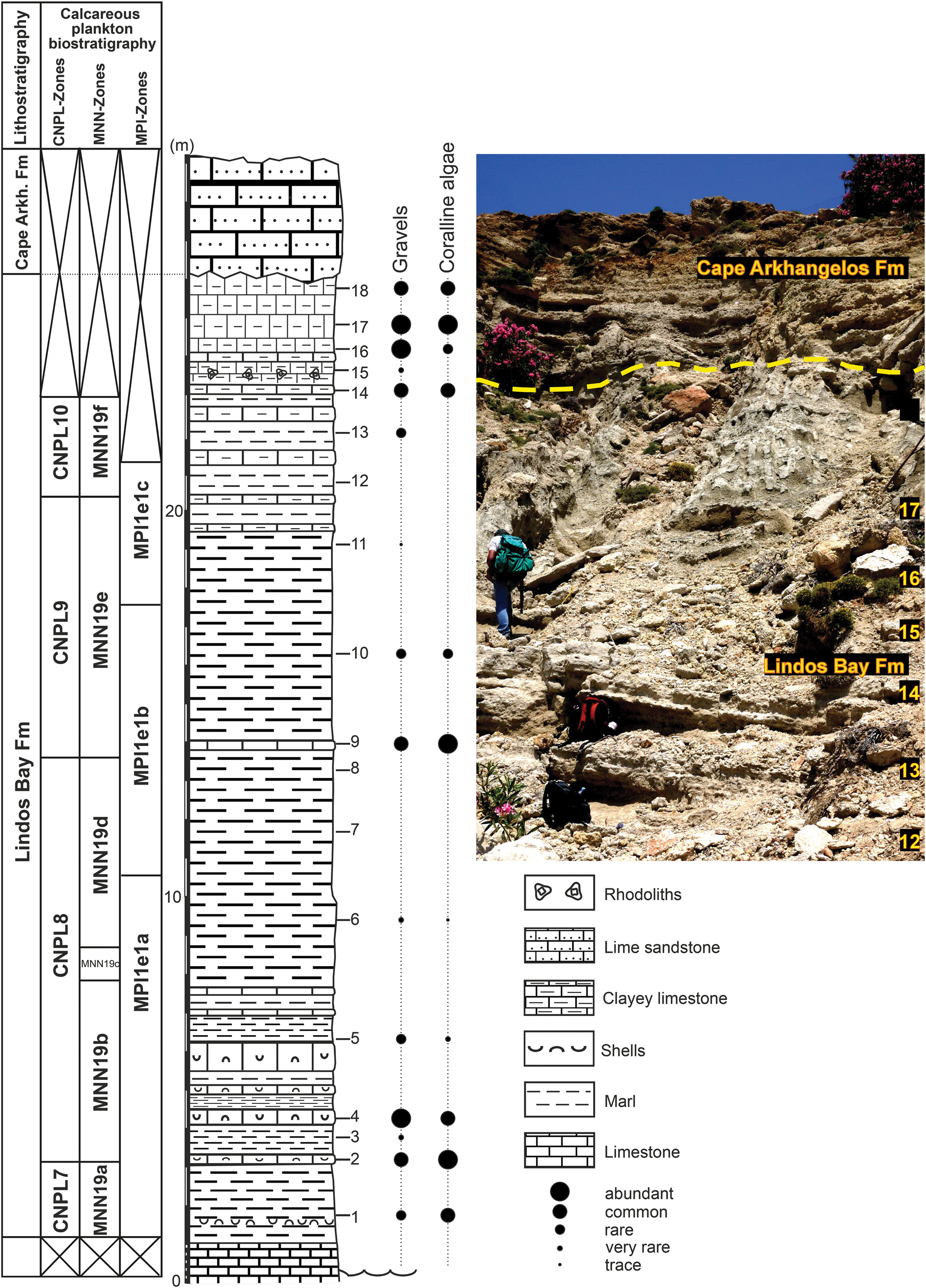
Figure 2. Lithostratigraphy and calcareous plankton (nannofossils and planktonic foraminifera) biostratigraphy of the Cape Arkhangelos-1 section. Tick marks show the locations of collected samples. Points of different sizes indicate the relative abundances of the transported gravels and coralline algae. Calcareous nannoplankton CNPL and MNN zonal schemes by Backman et al. (Reference Backman, Raffi, Rio, Fornaciari and Pälike2012) and Rio et al. (Reference Rio, Raffi, Villa, Kastens, Mascle, Auroux, Bonatti, Broglia, Channell, Curzi, Emeis and Glaçon1990), respectively. Planktonic foraminiferal MPle zonal scheme by Lourens et al. (Reference Lourens, Hilgen, Laskar, Shackleton, Wilson, Gradstein, Ogg and Smith2004).
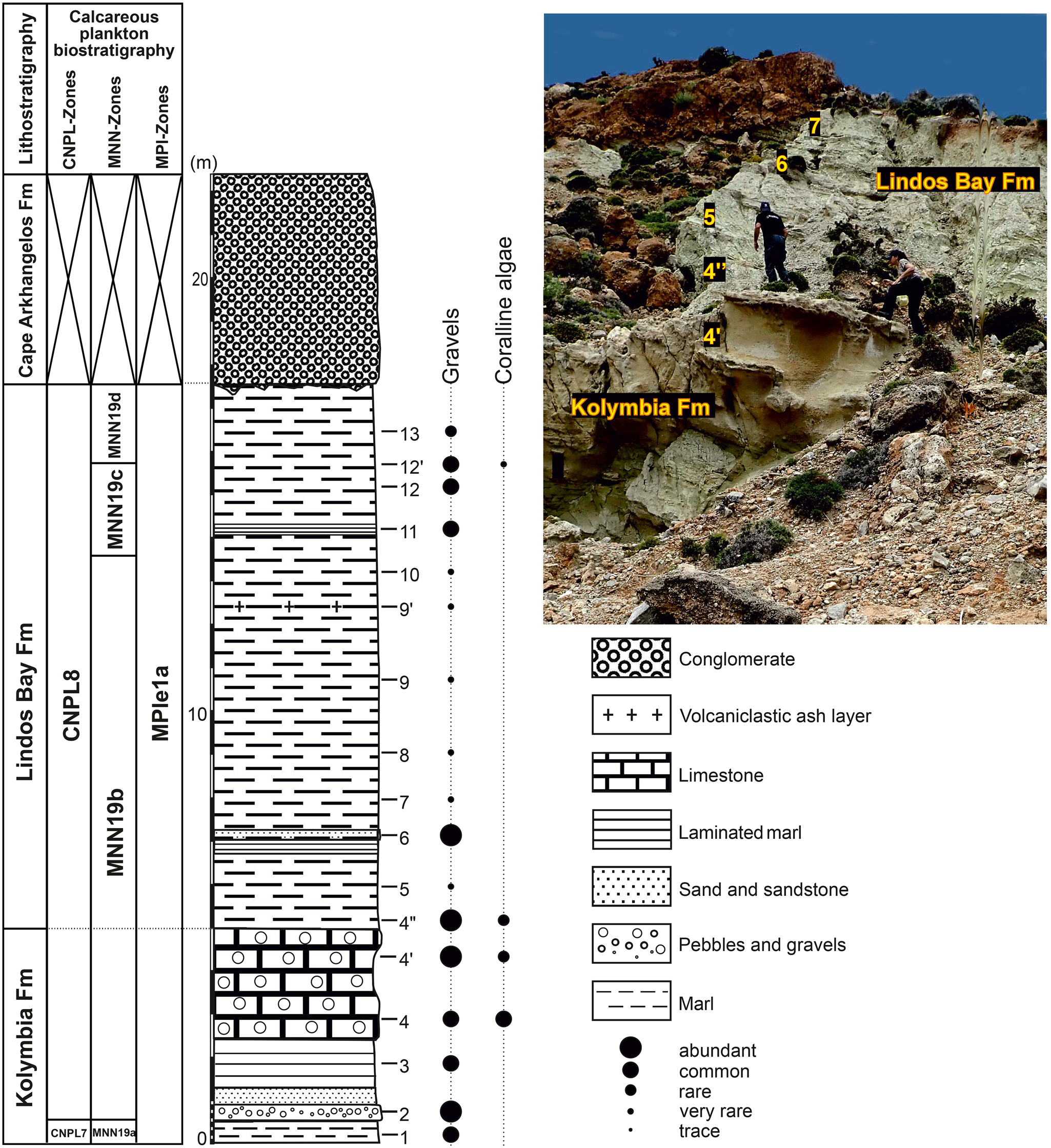
Figure 3. Lithostratigraphy and calcareous plankton (nannofossils and planktonic foraminifera) biostratigraphy of the Cape Arkhangelos-2 section. Tick marks show the locations of collected samples. Points of different sizes indicate the relative abundances of the transported gravels and coralline algae. Calcareous nannoplankton CNPL and MNN zonal schemes by Backman et al. (Reference Backman, Raffi, Rio, Fornaciari and Pälike2012) and Rio et al. (Reference Rio, Raffi, Villa, Kastens, Mascle, Auroux, Bonatti, Broglia, Channell, Curzi, Emeis and Glaçon1990), respectively. Planktonic foraminiferal MPle zonal scheme by Lourens et al. (Reference Lourens, Hilgen, Laskar, Shackleton, Wilson, Gradstein, Ogg and Smith2004).
Using a stereomicroscope, the gravels and coralline algae were first counted in each residue and their relative abundances were calculated to detect the horizons and samples influenced by the contribution of allochthonous, shallow-water material (Figs. 2 and 3). The micro- (planktonic and benthic foraminifera, bryozoans) and macro- (mollusks, brachiopods) fossils were then observed, identified, counted, and picked. For foraminiferal analyses, a minimum of 300 undamaged or complete specimens of both planktonic and benthic foraminifera from the 125-μm-size fraction were picked per residue (Supplementary Table 1). If a sample contained fewer than 300 undamaged specimens, then all available specimens were counted following the procedure of Schönfeld et al. (Reference Schönfeld, Alve, Geslin, Jorissen, Korsun and Spezzaferri2012). For the bryozoans, all specimens available from the residues were picked and identified (Supplementary Table 4). For the mollusks, all complete shells were picked from the >250-μm-size fractions, as well as fragments when they were identifiable to the family, genus, or species levels. Unique fragments were considered (e.g., the apex designated a single specimen for gastropods and scaphopods, and each umbo was assigned to 0.5 specimens for bivalves), following Kowalewski et al. (Reference Kowalewski, Gürs, Nebelsick, Oschmann, Piller and Hoffmeister2002). Some samples did not contain any identifiable material (small-sized fragments).
Qualitative and semi-quantitative analyses of the invertebrate and foraminiferal assemblages were ultimately performed in order to reconstitute the fossil content of these atypical depositional environments, to separate between deep-dwelling and transported taxa, and to obtain a first approximation of the overall conditions that occurred in the water column and the depth of deposition. The qualitative analysis involves identification and description of the species present in a sample, providing insights into diversity and distribution patterns. The faunal analysis adopted a semi-quantitative approach, categorizing occurrences per species as trace, very rare, rare, common, or abundant (Supplementary Tables 2–4), thereby offering a nuanced understanding of species abundance within the assemblages.
The bathymetric zonation used in this study is from van Morkhoven et al. (Reference van Morkhoven, Berggren and Edwards1986), with the approximate equivalent from Pérès and Picard (Reference Pérès and Picard1964) for the Mediterranean: inner neritic (= infralittoral; 0–50 m), middle neritic (= upper circalittoral; 50–100 m), outer neritic (= lower circalittoral; 100–200 m), upper slope (= upper bathyal; 200–600 m). Thirty-one deep-water indicators (9 benthic foraminifera, 12 bivalves, 1 scaphopod, and 9 bryozoans) selected from the literature (Fig. 4) among the 336 species identified in this study constitute the basis for the bathymetric subdivisions (Figs. 4 and 5).
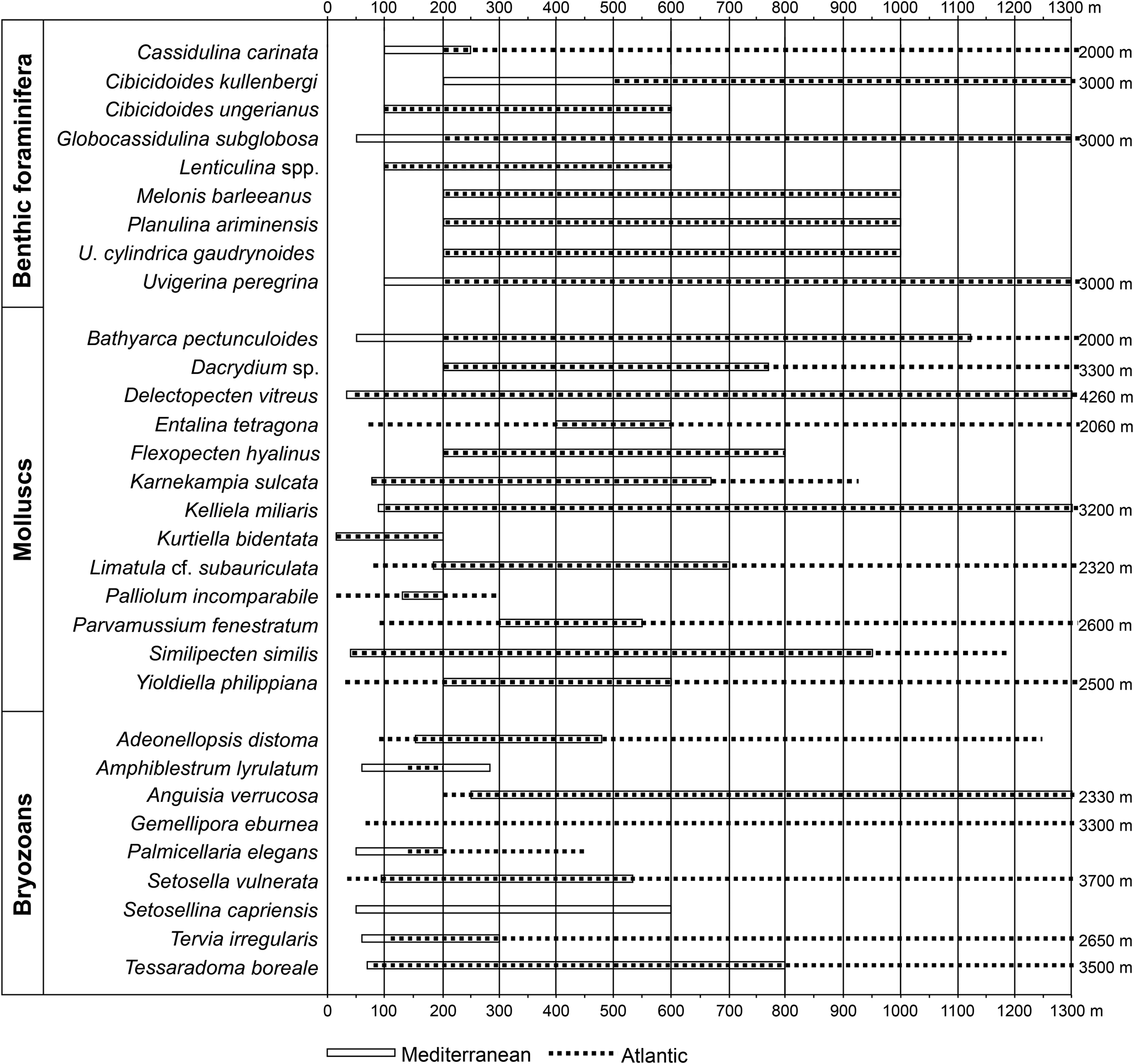
Figure 4. Bathymetric ranges of the deep-water extant species observed in the studied Cape Arkhangelos sections. For benthic foraminifera, bathymetric ranges originate from Parker (Reference Parker1958), Blanc-Vernet (Reference Blanc-Vernet1969), Wright (Reference Wright, Hsu, Montadert, Garrison, Fabricius, Mueller, Cita and Bizon1978), Parisi (Reference Parisi1981), Jorissen (Reference Jorissen1987), Sprovieri and Hasegawa (Reference Sprovieri, Hasegawa, Kastens, Mascle, Auroux, Bonatti, Broglia, Channell, Curzi, Emeis and Glaçon1990), Sgarrella and Moncharmont Zei (1993), de Stigter et al. (Reference de Stigter, Jorissen and Van der Zwaan1998), De Rijk et al. (Reference De Rijk, Jorissen, Rohling and Troelstra2000), Seidenkrantz et al. (Reference Seidenkrantz, Kouwenhoven, Jorissen, Shackleton and van der Zwaan2000), Kouwenhoven et al. (Reference Kouwenhoven, Hilgen and van der Zwaan2003), Drinia et al. (Reference Drinia, Antonarakou, Tsaparas and Dermitzakis2007), and Milker and Schmiedl (Reference Milker and Schmiedl2012). For mollusks, data are from Oliver and Allen (Reference Oliver and Allen1980), Warén (Reference Warén1989), Oschmann (Reference Oschmann1991), Peacock (Reference Peacock1993), Poppe and Goto (Reference Poppe and Goto1993), Salas (Reference Salas1996), Salas and Gofas (Reference Salas and Gofas1997), Koutsoubas et al. (Reference Koutsoubas, Tselepidis and Eleftheriou2000), Demir (Reference Demir2003), Petersen (Reference Petersen2004), Steiner and Kabat (Reference Steiner and Kabat2004), Dijkstra et al. (Reference Dijkstra, Warén and Gudmundsson2009), Mastrototaro et al. (Reference Mastrototaro, D'Onghia, Corriero, Matarrese, Maiorano, Panetta and Gherardi2010), Studencka et al. (Reference Studencka, Prysyazhnyuk and Ljul'eva2012), Negri and Corselli (Reference Negri and Corselli2016), and Krylova et al. (Reference Krylova, Sahling and Borowski2018). Records for bryozoans include the research of Harmer (Reference Harmer1957), Gautier (Reference Gautier1962), Prenant and Bobin (Reference Prenant, Bobin and Lechevallier1966), Harmelin (Reference Harmelin1976, Reference Harmelin1977), Ryland and Hayward (Reference Ryland and Hayward1977), Hayward and Ryland (Reference Hayward and Ryland1979), Harmelin and d'Hondt (Reference Harmelin and d'Hondt1982), and Rosso (Reference Rosso2002).

Figure 5. Early Pleistocene deep-water benthic foraminifera, bryozoans, and mollusks collected from the Cape Arkhangelos sections. (a) SEM photomicrographs of benthic foraminifera (scale bars = 100 μm): 1. Lenticulina sp., 2. Cassidulina carinata, 3. Globocassidulina subglobosa, 4. Uvigerina peregrina, 5. Uvigerina cylindrica cylindrica, 6. Melonis barleeanus, 7. Cibicidoides kullenbergi, 8. Planulina ariminensis, 9. Cibicidoides ungerianus. (b) SEM photomicrographs of erect bryozoans (scale bars = 200 μm): 1. Adeonellopsis distoma, 2. Amphiblestrum lyrulatum, 3. Anguisia verrucosa, 4. Gemellipora eburnea, 5. Tervia irregularis, 6. Palmicellaria elegans, 7. Tessaradoma boreale. (c) Photographs and SEM photomicrographs of mollusks (scale bars = 500 μm): 1. Palliolum incomparabile, 2. Entalina tetragona, 3. Limatula sp., 4. Dacrydium sp., 5. Yoldiella philippiana, 6. Keliella miliaris.
Biostratigraphy
Twenty-five unprocessed sediment samples, 12 from Arkhangelos-1 and 13 from Arkhangelos-2, have been investigated for calcareous nannofossil biostratigraphy. Calcareous nannofossils were analyzed in the size fraction 2–30 μm. Smear-slides were studied at 1200× magnification on an Olympus transmitting light microscope, both in crossed-nicols and polarized light. Semi-quantitative analyses of the calcareous nannofossils were achieved by counting 300 specimens per sample in randomly distributed transverses, in order to indicate the abundance patterns and the lowest occurrence (LO), lowest common occurrence (LCO), highest occurrence (HO), and highest common occurrence (HCO) of the stratigraphically significant taxa.
Pleistocene calcareous nannofossil assemblages are dominated by gephyrocapsids and reticulofenestrids. Consequently, due to the taxonomic complexity of the genus Gephyrocapsa (Raffi et al., Reference Raffi, Backman, Rio and Shackleton1993, 2006; Young, Reference Young and Bown1998), lengths of the coccolith outlines were measured to discriminate between medium (>3.5 to 5.5 μm) and large (>5.5 μm) morphotypes of this genus. The calcareous nannofossil biostratigraphy follows Rio et al. (Reference Rio, Raffi, Villa, Kastens, Mascle, Auroux, Bonatti, Broglia, Channell, Curzi, Emeis and Glaçon1990) and Backman et al. (Reference Backman, Raffi, Rio, Fornaciari and Pälike2012), whose zonation schemes have proven applicable for the Pleistocene deposits of the eastern Mediterranean, including the island of Rhodes (Thomsen et al., Reference Thomsen, Rasmussen and Hastrup2001; Quillévéré et al., Reference Quillévéré, Cornée, Moissette, López-Otálvaro, van Baak, Münch, Melinte-Dobrinescu and Krijgsman2016). Calibrations of bio-event ages in the eastern Mediterranean are according to Raffi et al. (Reference Raffi, Backman, Fornaciari, Pälike, Rio, Lourens and Hilgen2006).
Additional biostratigraphic constraints based on planktonic foraminifera rely on a complete inventory of morphospecies and morphotypes in all 35 washed samples in the >125-μm size fraction, following the taxonomic concepts and nomenclature of Kennett and Srinivasan (Reference Kennett and Srinivasan1983) and Iaccarino et al. (Reference Iaccarino, Premoli Silva, Biolzi, Foresi, Lirer, Turco and Petrizzo2007). Planktonic foraminiferal bio-event calibrations for the Pleistocene of the Mediterranean Sea come from Lourens et al. (Reference Lourens, Hilgen, Laskar, Shackleton, Wilson, Gradstein, Ogg and Smith2004), refined by Lirer et al. (Reference Lirer, Foresi, Iaccarino, Salvatorini, Turco, Cosentino, Sierro and Caruso2019).
Foraminiferal-based paleodepth estimates
Among the collected samples, only those with no to very rare amounts of gravels (Figs. 2 and 3) were selected for quantitative estimates of the depositional depth since the corresponding levels should contain very few transported (shallow water) benthic foraminifera. This fauna was analyzed using representative splits of the >125-μm size fraction. All foraminifera were picked from the split, mounted on faunal slides, and identified. The depositional depth of the sediments of both sections was then assessed by counting the number of planktonic foraminifera (P) relative to the total number of planktonic and benthic foraminifera (P+B), expressed as %P/(P+B) (van der Zwaan et al., Reference van der Zwaan, Jorissen and de Stigter1990). Several limiting factors play a significant role in controlling benthic and/or planktonic foraminiferal depth habitat, including sensitivity to changes in oxygen levels, food availability (e.g., Jorissen et al., Reference Jorissen, de Stigter and Widmark1995), and preferential dissolution of the planktonic foraminifera (e.g., Berger, Reference Berger1970; Honjo and Erez, Reference Honjo and Erez1978; Thunell and Honjo, Reference Thunell and Honjo1981; Murray and Alve, Reference Murray and Alve1999; Conan et al., Reference Conan, Ivanova and Brummer2002; Zarkogiannis et al., Reference Zarkogiannis, Iwasaki, Rae, Schmidt, Mortyn, Kontakiotis, Hertzberg and Rickaby2022), thus leading to potentially over- or under-estimated paleodepths. To overcome these limitations, we followed the methodology for collection of specimens originally proposed by van der Zwaan et al. (Reference van der Zwaan, Jorissen and de Stigter1990), later modified by van Hinsbergen et al. (Reference van Hinsbergen, Kouwenhoven and van der Zwaan2005) and Zachariasse et al. (Reference Zachariasse, Kontakiotis, Lourens and Antonarakou2021). Van der Zwaan et al. (Reference van der Zwaan, Jorissen and de Stigter1990) discarded the benthic genera Bolivina, Bulimina, Fursenkoina, Globobulimina, and Uvigerina as characteristic of organic-rich and oxygen-deficient environments. Van Hinsbergen et al. (Reference van Hinsbergen, Kouwenhoven and van der Zwaan2005) excluded Uvigerina hispida, U. peregrina, U. proboscidea, and U. semiornata from the stress markers and used them as depth markers. Moreover, the genera Cancris, Chilostomella, Rectuvigerina, Stainforthia, and Valvulineria were added as stress markers. Even though Hoeglundina elegans is an epifaunal to shallow-infaunal species, it also was discarded, because water depth is not a factor that affects the occurrence of this species (Pérez-Asensio et al., Reference Pérez-Asensio, Aguirre, Schmiedl and Civis2012). The distribution of these species is conceptualized as a function of organic flux and oxygenation, regulating the microhabitats of benthic foraminifera, following the TROX (trophic oxygen) model of Jorissen et al. (Reference Jorissen, de Stigter and Widmark1995, Reference Jorissen, Fontanier and Thomas2007). Infaunal species thrive with increased organic flux, especially in oxygen-depleted bottom waters (Jorissen, Reference Jorissen1999). Conversely, high percentages of epifaunal species generally indicate well-ventilated, oligotrophic marine conditions.
According to Murray (Reference Murray1991), the inner neritic is characterized by %P/(P+B) values up to 20%, the middle neritic by 20–40%, the outer neritic by 40–70%, and the upper bathyal by more than 70%. The quantitative calculation of foraminiferal data based on %P/(P+B) was checked with qualitative data based on depth-specific benthic marker species, considering their typical habitats and preferred depths in the Mediterranean region (Fig. 4).
Results
Lithological and faunal characteristics of the sections
In the Cape Arkhangelos area, the Kolymbia Formation rests upon a tectonized basement (Cornée et al., Reference Cornée, Quillévéré, Moissette, Fietzke, López-Otálvaro, Melinte-Dobrinescu and Philippon2019), which consists of folded and metamorphosed limestones of the Aegean nappes. The basement was crosscut by dominantly N–S trending normal faults that created horst and graben structures prior to the Pleistocene. As a consequence, the Kolymbia and Lindos Bay formations exhibit abrupt facies and thickness changes. In the westernmost part of the investigated area (Fig. 1C), these formations display sandy and clayey facies with debris flows that are 4–5 m thick (Cornée al., 2019) and are bounded to the east by a N–S trending fault (Fig. 1C). East of the fault, the formations display marly facies and reach a thickness of about 30 m, indicative of a graben infilling.
The Cape Arkhangelos-1 section (Fig. 2) mostly consists of the clayey and marly deposits of the Lindos Bay Formation. Several limestone (generally clayey) beds occur, notably near the base and at the top where gravels and coralline algae are overall more abundant. Samples 3, 6, 7, 8, 11, 12, and 15 exhibit no to very rare amounts of gravels and/or coralline algae (Fig. 2), and consequently were selected for %P/(P+B) based paleodepth estimates. Bivalve shell accumulations occur in the lowest part of the section and one rhodolith level was found in the upper part. The entire sequence is unconformably overlain by lime sandstones of the Cape Arkhangelos Formation. The benthic foraminifera are represented by about 90 species, which are less abundant and diverse in samples 14, 17, and 18. Forty-two mollusk species have been recognized: 21 bivalves, 20 gastropods (among them 7 holoplanktonic taxa), and 1 scaphopod. The bryozoans are generally abundant and diverse (total: 120 species; average: 25 species/sample), excepted in level 12 where only a few fragments belonging to 2 taxa have been found.
The Cape Arkhangelos-2 section (Fig. 3) first exposes sediments of the Kolymbia Formation: marl (massive or laminated), limestone (with pebbles and gravels), and sandstone beds. The overlying Lindos Bay Formation comprises predominantly massive marly deposits, which are intercalated with a few thin beds of laminated marls and sands. A volcaniclastic ash layer was also observed near the top of the marls of the Lindos Bay Formation. The uppermost conglomerates of the Cape Arkhangelos Formation are separated from the underlying beds by a strong erosional surface. Gravels and/or coralline algae are more abundant in the lower and upper parts of the section. Only samples 5, 7, 8, 9, 9′, and 10 yielded no to very rare amounts of such transported elements (Fig. 3), and consequently were selected for %P/(P+B) based paleodepth estimates. Benthic foraminiferal species diversity is almost like that of the Cape Arkhangelos-1 section, with high numbers of transported components such as Elphidium spp. and Cibicides lobatulus, accompanied by fewer bathyal elements such as Cibicidoides spp., Planulina ariminensis, and Melonis barleeanus (Fig. 5), together with agglutinated forms. The transport of typical shallow-water foraminifera into deep-sea settings is indicated by the identification of shallow-water foraminiferal species in bathyal sediments and the preservation state of the foraminifera, as well as the examination of sediment grain sizes and assemblage changes. A mismatch between the grain sizes of bathyal sediments and the typical littoral foraminiferal habitat, along with the sudden appearance of shallow-water species in the bathyal assemblage, may indicate transport. Among the 26 mollusk species found in this section, 18 bivalves have been identified, along with 7 gastropods, and 1 scaphopod. Mostly calcitic shells are preserved in this section and thus pectinid species are predominant, together with a few brachiopods occurring in 5 samples. Bryozoans, totaling 103 species, also were found. Their abundance and average diversity (28 species/sample) are also relatively high, except for samples 5 and 7 (7 and 9 species, respectively).
Apart from the above, various groups of marine organisms are represented in both sections: calcareous algae, sponges (siliceous spicules), solitary corals, tubeworms (ditrupids and serpulids), crustaceans (decapods, ostracods, and barnacles), echinoderms (echinoids mostly, but also ophiuroids and crinoids), and fish (otoliths, teeth, and bone fragments).
Biostratigraphy
Ten out of the 12 analyzed samples from the Arkhangelos-1 section yielded calcareous nannofossils with preservation ranging from poor to good (samples 14 and 16, collected at the top of the section, were barren). From bottom to top in this section, we identified a biostratigraphic succession encompassing zones and subzones CNPL7–CNPL8 and MNN19a–MNN19f (Fig. 2) based on the LO of medium Geophyrocapsa (1.73 Ma), the HO of Calcidiscus macintyrei (1.664 Ma), the LO of large Gephyrocapsa (1.617 Ma), the HO of Helicosphaera sellii (1.256 Ma), the HO of large Gephyrocapsa (1.245 Ma), the LCO of Reticulofenestra asanoi (1.078 Ma), and the re-entrance datum of medium Gephyrocapsa (0.956–0.985 Ma). Additional key information results from the absence of Discoaster brouweri (HO at 1.95 Ma) at the base of the section and the continuous common occurrence of R. asanoi (HCO at 0.91 Ma) in the uppermost samples that yielded nannofossils.
All samples from the Arkhangelos-2 section yielded calcareous nannofossils with preservation ranging from poor to moderate. From bottom to top in this section, we identified a biostratigraphic succession encompassing zones and subzones CNPL7–CNPL8 and MNN19a–MNN19d (Fig. 3) based on the LO of medium Gephyrocapsa (1.73 Ma), the HO of Calcidiscus macintyrei (1.664 Ma), and the LO of large Gephyrocapsa (1.617 Ma). Additional key information results from the absence of Discoaster brouweri (HO at 1.95 Ma) at the base of the section and the continuous occurrence of Helicosphaera sellii (HO at 1.256 Ma) at the top. Besides biostratigraphic important taxa (e.g., Calcidiscus macintyrei, Helicosphaera sellii, Reticulofenestra asanoi, along with large and medium-sized Gephyrocapsa), some long-ranging nannofossils (e.g., Calcidiscus leptoporus, Helicosphaera carteri, Pontosphaera japonica, P. multipora, Pseudoemiliania lacunosa, Rhabdosphaera clavigera, and Syracosphaera spp.) are common throughout the studied successions (Fig. 6).
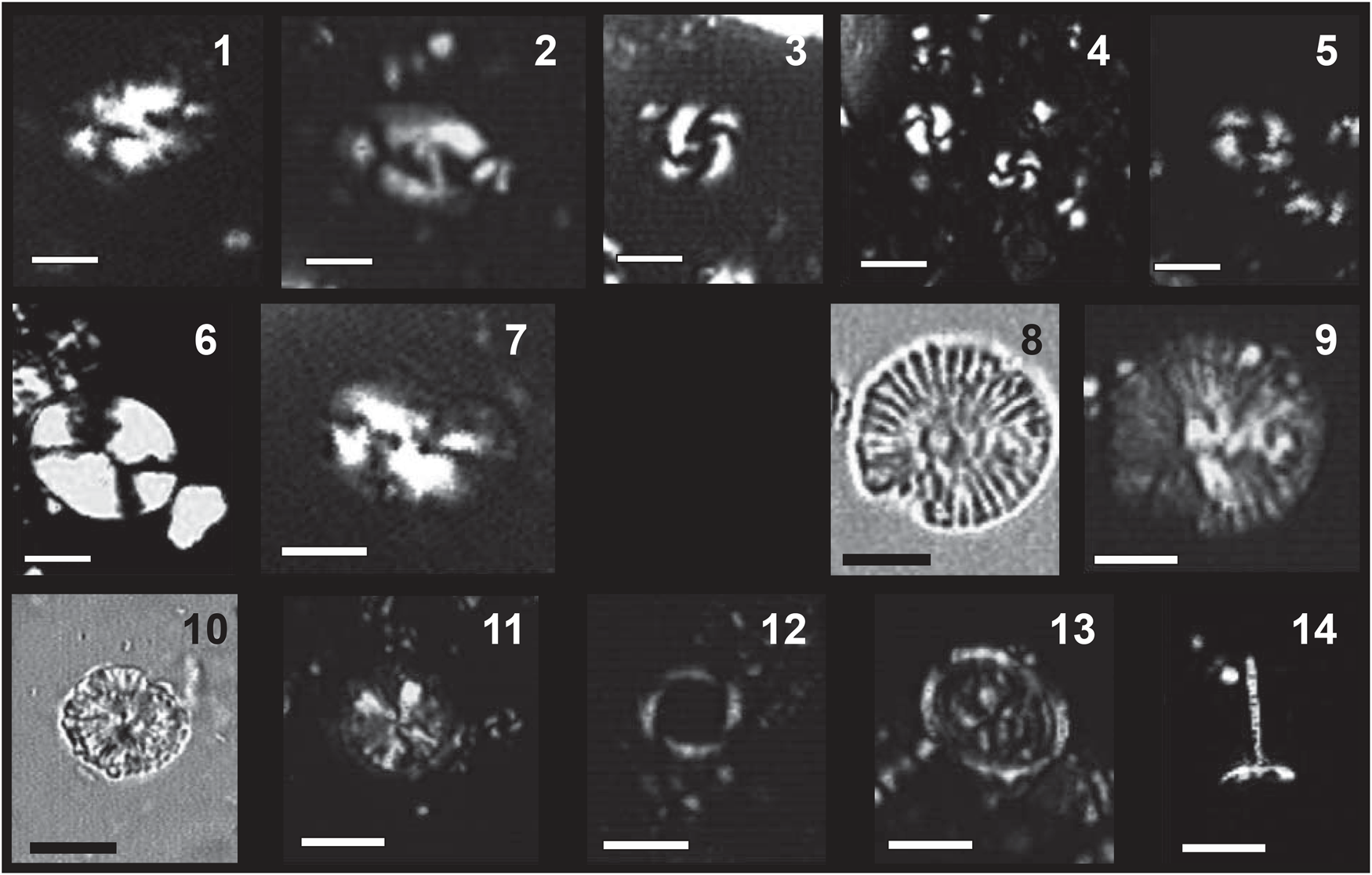
Figure 6. Biostratigraphic important nannoplankton taxa from the studied Arkhangelos sections. (1, 2) Helicosphaera sellii. (3) Large Gephyrocapsa sp. (4) Medium Gephyrocapsa sp. (5) Reticulofenestra asanoi. (6) Pontosphaera japonica. (7) Helicosphaera carteri. (8, 9) Calcidiscus macintyrei. (10, 11) Calcidiscus leptoporus. (12) Pseudoemiliania lacunosa. (13) Syracosphaera pulchra. (14) Rhabdosphaera clavigera. All microphotographs taken with LM (light microscope) in N+ (crossed-nicols) except (8) and (10) which are in NII (crossed-polarized light); scale bars = 5 μm.
Planktonic foraminifera are abundant in all analyzed samples and their preservation in the entire Lindos Bay Formation succession of both sections is very good. The base of the Arkhangelos-1 section is characterized by the common occurrence of sinistral Neogloboquadrina spp., indicating an age younger than 1.79 Ma (Lourens et al., Reference Lourens, Hilgen, Laskar, Shackleton, Wilson, Gradstein, Ogg and Smith2004). This correlates the base of the section with Subzone MPle1a. The absence of sinistral Neogloboquadrina spp. in the middle part of the section (samples 7 to 10) correlates with the paracme interval of these morphotypes, which has been calibrated between 1.37 and 1.21 Ma within the Mediterranean Sea (Subzone MPle1b; Lourens et al., Reference Lourens, Hilgen, Laskar, Shackleton, Wilson, Gradstein, Ogg and Smith2004). The FCO of Globorotalia truncatulinoides excelsa was also identified in sample 12, indicating an age of 0.934 Ma at the top of Subzone MPle1c (Lourens et al., Reference Lourens, Hilgen, Laskar, Shackleton, Wilson, Gradstein, Ogg and Smith2004). In the Arkhangelos-2 section, the common occurrence of sinistral Neogloboquadrina spp. is indicative of the interval between the FCO and paracme base (PB) of these morphotypes, corresponding to Subzone MPle1a (part). Other biostratigraphically important taxa found in the Arkanghelos-1 and Arkhangelos-2 sections include Globoconella inflata (LO = 2.09 Ma) and Globorotalia truncatulinoides (LO = 2.0 Ma).
Overall, the Lindos Bay Formation succession in the study area spans calcareous nannofossil subzones MNN19b–MNN19f (Rio et al., Reference Rio, Raffi, Villa, Kastens, Mascle, Auroux, Bonatti, Broglia, Channell, Curzi, Emeis and Glaçon1990), which correlate with zones CNPL8–CNPL10 (Backman et al., Reference Backman, Raffi, Rio, Fornaciari and Pälike2012) and planktonic foraminiferal Zone MPle1 (Lourens et al., Reference Lourens, Hilgen, Laskar, Shackleton, Wilson, Gradstein, Ogg and Smith2004; Lirer et al., Reference Lirer, Foresi, Iaccarino, Salvatorini, Turco, Cosentino, Sierro and Caruso2019). The Arkhangelos-1 sediments were deposited during the Calabrian, between ca. 1.8 Ma and ca. 0.9 Ma, and those of the Arkhangelos-2 section were deposited during the early Calabrian, probably between ca. 1.8 Ma and ca. 1.6 Ma. The altitude (12.5 m, sample 9′) of the volcaniclastic ash layer found in the Arkhangelos-2 section (Fig. 3) is not in full agreement with the biostratigraphic results. Indeed, a single volcaniclastic ash layer has been found and dated in other Lower Pleistocene sections of the Lindos Bay Formation of Rhodes, namely at Haraki (1.89 ± 0.09 Ma; Cornée et al., Reference Cornée, Münch, Quillévéré, Moissette, Vasiliev, Krijgsman, Verati and Lécuyer2006b) and at Lindos Bay (1.85 ± 0.08 Ma; Quillévéré et al., Reference Quillévéré, Cornée, Moissette, López-Otálvaro, van Baak, Münch, Melinte-Dobrinescu and Krijgsman2016). In section Arkanghelos-2, the volcaniclastic ash layer occurs in the 1.73–1.664 Ma interval. Consequently, either its age is slightly younger (at least 70 ka) than previously suspected, or it correlates with another pyroclastic fallout that occurred later in the Early Pleistocene.
Foraminiferal-based paleodepth estimates
In the 12 selected samples containing no or almost no gravels and coralline algae, all from the marls of the Lindos Bay Formation, %P/(P+B) values range between 16% and 67% in the Arkhangelos-1 section, and between 55% and 75% in the Arkhangelos-2 section (Table 1). Following Murray (Reference Murray1991), the lowermost part of the Arkhangelos-1 section was deposited at upper bathyal depths (around 385 m), then deposition occurred in the outer neritic (between ~185 m and ~115 m), and later in the middle neritic (between ~87 m and ~65 m) in the uppermost part of the section (Fig. 7). The middle neritic paleodepths may be underestimated in the upper part of the section and deposition may have occurred deeper because of the occurrence of deep-water indicators (for example, the deep-sea mytilid bivalves Dacrydium sp. found in sample 11). In the Arkhangelos-2 section, the Lindos Bay Formation sediments were deposited in the upper bathyal zone with a shallowing-upward trend from ~513 m to ~254 m (Fig. 8).
Table 1. Approximate paleodepth estimates based on planktonic/benthic foraminiferal %P/(P+B) values measured in samples with scarce gravel and coralline algae contents. Paleodepth estimates based on %P/(P+B) values need to be taken cautiously (van der Zwaan et al., Reference van der Zwaan, Jorissen and de Stigter1990; van Hinsbergen et al., Reference van Hinsbergen, Kouwenhoven and van der Zwaan2005) and are combined in this study with qualitative data based on depth-specific benthic foraminiferal, mollusk, and bryozoan marker species.

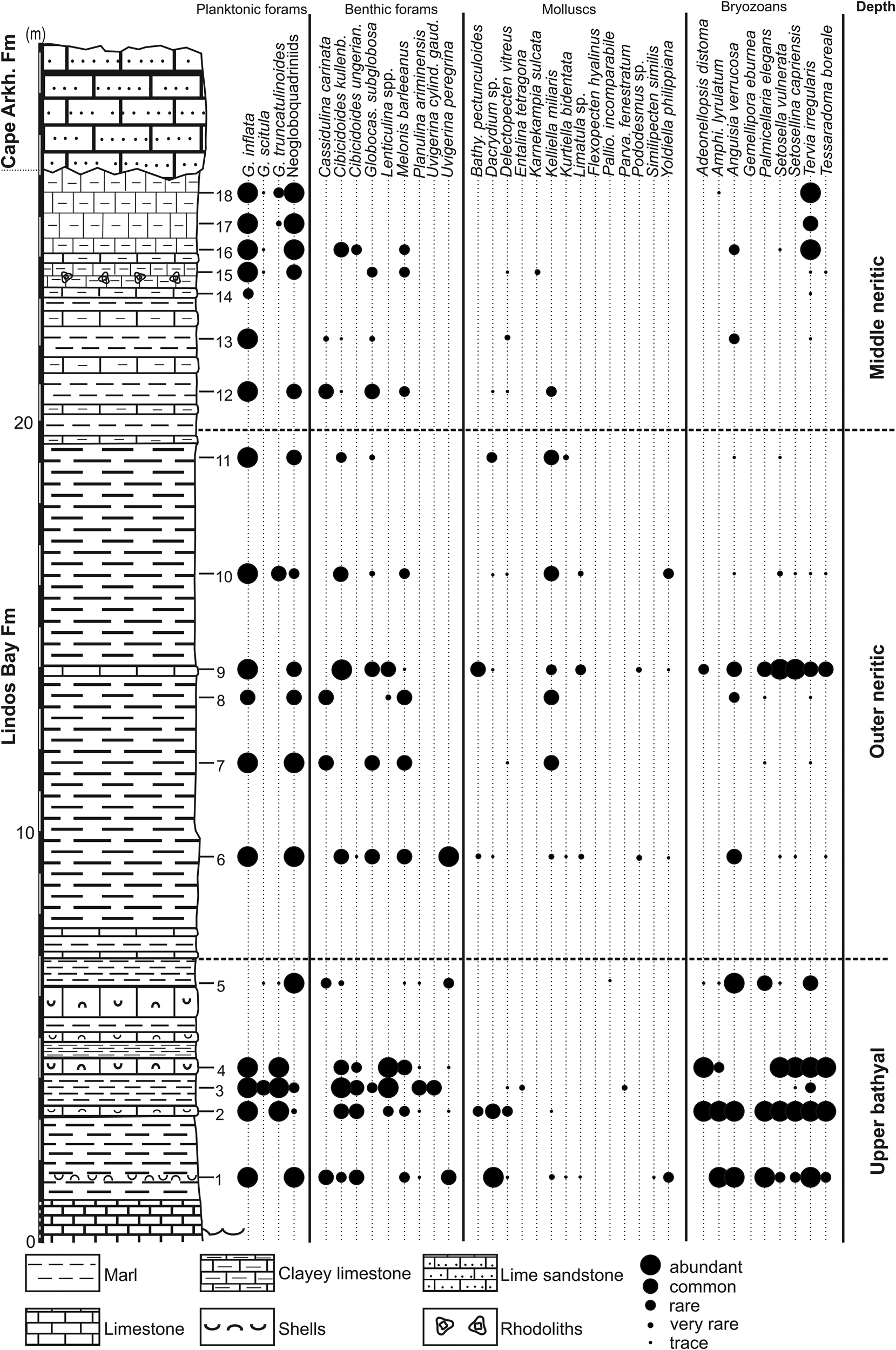
Figure 7. Cape Arkhangelos-1 section with semi-quantitative abundances of deep-dwelling planktonic foraminifera and deep-water indicators (benthic foraminifera, mollusks, and bryozoans).
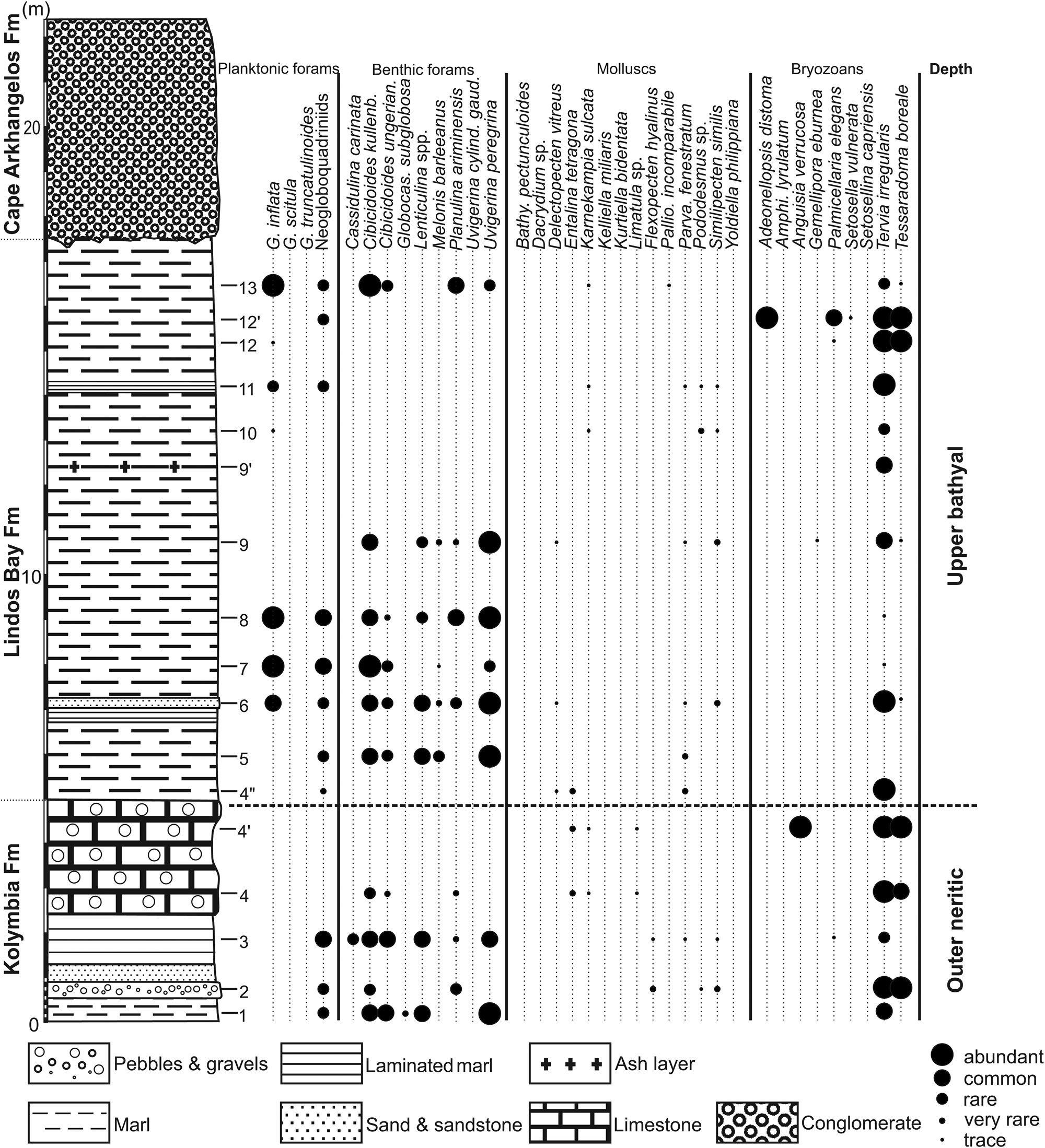
Figure 8. Cape Arkhangelos-2 section with semi-quantitative abundances of deep-dwelling planktonic foraminifera and deep-water indicators (benthic foraminifera, mollusks, and bryozoans).
Biota
Planktonic foraminifera
Planktonic foraminiferal assemblages consist of a well-diversified fauna containing 14 species and morphotypes: Globigerinoides ruber, Trilobatus trilobus, Globigerinoides conglobatus, Orbulina universa, Globigerina bulloides, Globorotalia scitula, Globorotalia truncatulinoides, Globoconella inflata, Turborotalita quinqueloba, Globoturborotalita apertura, Globigerinella siphonifera, Globigerinita glutinata, and sinistral and dextral Neogloboquadrina spp. Among them, Globigerinoides ruber, Globoconella inflata, and Globigerina bulloides are the most abundant species. Although no significant temporal changes in the planktonic foraminiferal assemblages are observed, Globoconella inflata, Globorotalia scitula, Globorotalia truncatulinoides, and Neogloboquadrina spp. highlight distinctive paleoceanographic settings (Figs. 7 and 8). All these deep-dwelling subtropical to subpolar (Hemleben et al., Reference Hemleben, Spindler and Anderson1989) taxa are usually considered as indicative of relatively cool, mesotrophic water conditions in temperate regions such as the eastern Mediterranean (Rohling et al., Reference Rohling, Jorissen, Vergnaud-Grazzini and Zachariasse1993; Pujol and Vergnaud-Grazzini, Reference Pujol and Vergnaud-Grazzini1995; Rohling et al., Reference Rohling, Jorissen and Stigter1997; Giamali et al., Reference Giamali, Koskeridou, Antonarakou, Ioakim, Kontakiotis, Karageorgis, Roussakis and Karakitsios2019, Reference Giamali, Kontakiotis, Koskeridou, Ioakim and Antonarakou2020, Reference Giamali, Kontakiotis, Antonarakou and Koskeridou2021). Their abundance in this setting is mainly controlled by winter convection and vertical mixing (Pujol and Vergnaud-Grazzini, Reference Pujol and Vergnaud-Grazzini1995), while, particularly for Neogloboquadrina spp., their abundance is mostly associated with the development of the Deep Chlorophyl Maximum (DCM) at the base of the euphotic layer (Rohling and Gieskes, Reference Rohling and Gieskes1989). During early summer, when stratification begins and DCM is absent, they live at the bottom or below the thermocline (Hemleben et al., Reference Hemleben, Spindler and Anderson1989) and therefore they can be used as indicators of the overall position of the subsurface thermocline (Faul et al., Reference Faul, Ravelo and Delaney2000). The co-existence of Globoconella inflata and Globorotalia truncatulinoides in both sections indicates an isothermal water column throughout the euphotic zone because of the strong seasonal convective mixing (Pujol and Vergnaud-Grazzini, Reference Pujol and Vergnaud-Grazzini1995), possibly indicating the presence of intermediate water masses, with the intense action of Levantine Intermediate Waters (LIW) in the south Aegean Sea (Rohling et al., Reference Rohling, Jorissen and Stigter1997; Kontakiotis, Reference Kontakiotis2016).
Benthic foraminifera
The total benthic foraminiferal data set (Supplementary Table 2) comprises 90 taxonomic units (genera, species, and species groups). The ‘Cibicides lobatulus group’ (C. lobatus and C. refulgens) is indicative of inner to middle neritic epifaunal habitats in high-energy settings (Schönfeld, Reference Schönfeld2002; Louvari et al., Reference Louvari, Drinia, Kontakiotis, Di Bella, Antonarakou and Anastasakis2019). In the two sections, common neritic taxa such as Miliolidae (Pyrgo depressa, Quinqueloculina lamarckiana, Quinqueloculina padana, Quinqueloculina spp.), Reusella spinulosa, and Elphidium spp. (Elphidium aculeatum, Elphidium advenum, Elphidium crispum, Elphidium macellum) were most likely transported downslope by turbidity currents (Murray, Reference Murray2006). Furthermore, sporadic occurrences of taxa with presumed epiphytic growth, such as Cibicides lobatulus, asterigerinids, and Rosalina globularis, may be attributed to turbidity-current transport or attachment to floating plant material, which then decayed and deposited them (Drinia, Reference Drinia2009). Agglutinated foraminifera, including Bigenerina nodosaria, Textularia agglutinans, Textularia conica, Textularia gramen, Textularia pseudotrochus, and Textularia saggitula, are sporadically present in both sections with no specific environmental preferences identified.
Bathyal taxa such as Cibicidoides spp. (including C. kullenbergi and C. ungerianus/pseudoungerianus), Planulina ariminensis, Melonis barleeanus, and Lenticulina spp. (grouping L. peregrina and L. calcar) suggest an affinity with finer-grained sediments (e.g., Linke and Lutze, Reference Linke and Lutze1993; Pérez-Asensio and Aguirre, Reference Pérez-Asensio and Aguirre2010). Cibicidoides spp. exhibit an upper depth limit within the middle bathyal zone in the Gulf of Mexico (Pflum and Frerichs, Reference Pflum and Frerichs1976). Furthermore, C. ungerianus and C. kullenbergi are notably abundant deep-water taxa below 100–120 m (Jorissen, Reference Jorissen1988; Sgarrella and Moncharmont Zei, Reference Sgarrella and Moncharmont Zei1993). This species group, commonly classified as epifaunal (Jorissen, Reference Jorissen1987), shows a preference for well-oxygenated bottoms with high organic carbon fluxes (Woodruff, Reference Woodruff and Kennett1985; Murgese and De Deckker, Reference Murgese and De Deckker2005). Melonis barleeanus, which is considered an intermediate-/deep-infaunal species linked to moderately organic-rich sediment (Pflum and Frerichs, Reference Pflum and Frerichs1976; Jorissen, Reference Jorissen1987), primarily feeds on degraded organic detritus buried in sediments (Fontanier et al., Reference Fontanier, Jorissen, Licari, Alexandre, Anschutz and Carbonel2002). The genus Uvigerina thrives in low-energy, shallow-infaunal microhabitats rich in organic carbon, often linked to reduced bottom-water oxygen content (Thomas and Gooday, Reference Thomas and Gooday1996; Fariduddin and Loubere, Reference Fariduddin and Loubere1997). Uvigerina becomes more abundant with depth, particularly in fine-grained sediments near shelf breaks with local upwelling (Gupta and Srinivasan, Reference Gupta and Srinivasan1990; Sen Gupta and Marchain-Castillo, Reference Sen Gupta and Marchain-Castillo1993). Among the uvigerinids, Uvigerina peregrina is common throughout both sections, with percentages fluctuating between 4% and 30%.
In the lower part of the Arkhangelos-1 section (Fig. 7), the dominance of Cibicidoides spp. along with species that typically inhabit deep-water slope settings (e.g., Planulina ariminensis and Siphonina reticulata), suggests prevailing deep-water conditions (samples 1–4 and 6). This is supported by the common occurrence in sample 3 of Uvigerina cylindrica gaudrynoides, a species that inhabits oxygen-deficient and nutrient-rich environments (van der Zwaan, Reference van der Zwaan1982; Drinia et al., Reference Drinia, Antonarakou, Tsaparas and Dermitzakis2007). Uvigerina cylindrica gaudrynoides typically ranges from the upper to the middle bathyal (200–1000 m), and occasionally occurs as shallow as 150 m and as deep as 1200 m (Haake, Reference Haake1980; Lutze, Reference Lutze1980; Schiebel, Reference Schiebel1992; Schweizer, Reference Schweizer2006). In sample 1, the presence of the Cibicides lobatulus group and high percentages of Miliolidae and Elphidium spp. are attributed to transportation from middle to outer neritic settings by turbidity currents, because they co-occur with multiple species of deep-sea invertebrates. Samples 9–10, 13, and 15–18 display high abundances of shallow-water taxa, such as Asterigerinata mamila, A. planorbis, Neoconorbina terquemi, and Rosalina globularis. These species, commonly found at greater depths in Mediterranean sediments (e.g., van der Zwaan, Reference van der Zwaan1983; Jorissen, Reference Jorissen1987), are considered allochthonous.
In the Arkhangelos-2 section (Fig. 8), Elphidium species dominate the benthic foraminiferal assemblages, accompanied by lesser amounts of Cibicidoides spp. and Planulina ariminensis. The shallow-water preferences of Elphidium spp. (Hayward et al., Reference Hayward, Grenfell, Pullin, Reid and Hollis1997), coupled with the presence of species typical of deep-water habitats, even in small percentages, can be attributed to sediment displacement (e.g., Phleger et al., Reference Phleger, Parker, Peirson and Pettersson1953) or rafting of plant material, which carried epiphytes into pelagic environments after storms (Sprovieri and Hasegawa, Reference Sprovieri, Hasegawa, Kastens, Mascle, Auroux, Bonatti, Broglia, Channell, Curzi, Emeis and Glaçon1990). Common to abundant occurrences of Uvigerina peregrina, along with fewer Cibicidoides spp. and Lenticulina spp. in samples 1, 3, 5–6, and 8–9, support deposition in deep environments (Pflum and Frerichs, Reference Pflum and Frerichs1976; Schnitker, Reference Schnitker1979, Reference Schnitker1980; Qvale and van Weering, Reference Qvale and van Weering1985; Gupta and Srinivasan, Reference Gupta and Srinivasan1990). The inner to middle neritic epifaunal Cibicides lobatulus group is found in samples 3, 5, 6, and 13. High percentages of Textularia agglutinans (>40%) and Elphidiidae (>40%) in samples 2 and 4 suggest either a shallower outer neritic zone or transportation. In sample 10 they indicate transportation. Finally, abundant Cibicidoides in samples 7 and 13 indicate an upper bathyal environment.
Mollusks and brachiopods
The total number of mollusk species for the two sections amounts to 83. Bivalves have the highest diversity (38 species; shallow and deep), followed by shallow-water gastropods (35 species), holoplanktonic gastropods (7 species), and deep-water gastropods (2 species). The scaphopods are represented by only 1 (deep-water) species, Entalina tetragona. Almost all samples contain fragments of bivalve shells, which are not identifiable at a low taxonomic level; nevertheless, they are mostly fragments of pectinids and anomiids.
The Arkhangelos-1 section (Fig. 7) is characterized by a higher taxonomic diversity than the Arkhangelos-2 section, with a total of 21 species characteristic of deep-water environments: 11 bivalves, 7 holoplanktonic gastropods, 2 bathyal gastropods, and 1 species of scaphopod. Many bivalves (Yoldiidae, Mytilidae, Arcidae or Kelliellidae) are found with preserved aragonitic shells. Minute pectinid bivalves, represented by small fragments and juvenile specimens, are found in most samples. In sample 1, an association of Dacrydium sp., Yoldiella philippiana, and Kelliella miliaris (Fig. 5C) indicates the upper bathyal zone. A second association, recovered in sample 6 and containing Bathyarca pectunculoides, Delectopecten vitreus, and Limatula cf. L. subauriculata, is characteristic of outer neritic to upper bathyal depths (180–600 m). In sample 11, the mollusk association is dominated by Kelliella miliaris, which co-occurs with Dacrydium sp. and Kurtiella bidentata, indicating outer neritic depths (up to 200 m). Yoldiella philippiana (samples 1, 6, 9, 10) and Bathyarca pectunculoides (samples 2, 6, 9) are commonly found at bathyal depths (e.g., Di Geronimo and La Perna, Reference Di Geronimo and La Perna1997; La Perna, Reference La Perna2003), but in the Atlantic, Y. philippiana has been documented from the lower bathyal up to the middle neritic, and in the Mediterranean, B. pectunculoides is known from the middle neritic zone and deeper (e.g., Janssen and Krylova, Reference Janssen and Krylova2014). A varied fauna of holoplanktonic gastropods (Pteropoda and Littorinimorpha) in samples 1–2 and 6–8 includes Clio pyramidata, Creseis sp., Thielea cf. T. helicoides, Atlanta sp., and Hyalocyclis sp. Holoplanktonic gastropods are known from deep environments of 200 m or more (e.g., Berger, Reference Berger1978; Janssen, Reference Janssen2012; Janssen et al., Reference Janssen, Sessa and Thomas2016). Since all the recovered specimens are well preserved, we consider that they have not been transported. The deep-water gastropods Anatoma crispata (samples 3, 6, 9, and 10) and Cornisepta rostrata (sample 6) further highlight the deep-water depositional settings (Berger, Reference Berger1978). Sample 3 yielded the scaphopod Entalina tetragona, which is known to thrive at depths between 400 and 600 m in the Mediterranean (Fig. 4) (Negri and Corselli, Reference Negri and Corselli2016).
In the Arkanghelos-2 section (Fig. 8), eight deep-water mollusk species were found (7 bivalves and 1 scaphopod). The associations of pectinids are characteristic of deep-water environments. Here, six species of deep-water pectinids were recovered, indicating slightly deeper environments than the other taxa, as well as one species of Limiidae (Limatula sp.) and the scaphopod Entalina tetragona. Similipecten similis and Parvamussium fenestratum are found together with Delectopecten vitreus in upper bathyal environments (sample 6) or with Flexopecten hyalinus in outer neritic to upper bathyal settings (sample 3). The association of Karnekampia sulcata and Limatula sp. in sample 4 also indicates depths corresponding to the outer neritic or upper bathyal. Sample 4″ recorded a deeper environment (upper bathyal) with the association of P. fenestratum, D. vitreus, and the scaphopod Entalina tetragona. Karnekampia sulcata is also found associated with Palliolum incomparabile (Fig. 5C) in the uppermost part of the section (sample 13), characterizing an outer neritic to upper bathyal environment.
Numerous samples exhibit transported elements from shallower depths that have been deposited in deeper water. For the Arkhangelos-1 section (samples 6, 9, 10, and 12), holoplanktonic gastropods, such as Limacina trochiformis and Atlanta sp., are also present. The transported assemblages include the gastropods Bittium reticulatum, Jujubinus sp., Alvania spp., and bivalves of the families Cardiidae (Papillicardium papillosum, Acanthocardia echinata) and Timoclea ovata. The richest assemblage is that of sample 9, followed by sample 6. For sample 12 of the Arkanghelos-2 section, the transported assemblage is dominated by the gastropods Bittium reticulatum and Bittium lacteum, with other relatively abundant species such as Clanculus cruciatus, Jujubinus sp., and species of Alvania. Shallow-water bivalves include Arca noae, Striarca lactea, Hiatella arctica, Glycymeris glycymeris, Timoclea ovata, Ostrea edulis, and juveniles of the family Veneridae. The deep-water elements of this sample are fragments of characteristic thin-shelled pectinids.
In addition to mollusks, four deep-water brachiopod species are present in the Arkhangelos-2 section, notably in sample 12. These species are Terebratulina retusa and Megerlia truncata, both living in outer neritic to upper bathyal environments (Logan et al., Reference Logan, Bianchi, Morri and Zibrowius2004; Bitner et al., Reference Bitner, Zágoršek and Hladilová2013). Other brachiopods (Macadrevia cranium and Platidia anomioides) also occur in samples 2, 3, 4, 6, and 12. The presence of deep-water brachiopods confirms that the littoral mollusks of sample 12 were transported and deposited into a middle to outer neritic environment.
Bryozoans
About 160 bryozoan species have been identified in the Arkhangelos-1 and Arkhangelos-2 sections. This species richness is far beyond those evinced in other sections of the Lindos Bay Formation, which yielded fewer than 20 species (Moissette and Spjeldnaes, Reference Moissette and Spjeldnaes1995). At Cape Arkhangelos, bryozoans are represented by encrusting (94 species), erect rigid cylindrical (29 species), erect rigid bilaminate (8 species), erect rigid fenestrate (3 species), erect articulated (9 species), nodular (11 species), and free-living discoidal (2 species) morphotypes (Moissette, Reference Moissette2000). The predominance of encrusting forms throughout (with the exception of two species living on small skeletal fragments in deeper, muddy settings) indicates a certain transport from relatively shallow waters (Moissette, Reference Moissette2000), which is confirmed by the occurrence of remains of erect morphotypes often fragmented, some of them also being worn and yellowish.
Apart from numerous eurybathic species, most of the bryozoans are characteristic of shallow to moderate depths (infralittoral to upper circalittoral). Examples of shallow-water inner neritic species are: Caberea boryi, Calpensia nobilis, Chaperia annulus, Hagiosynodos latus, Margaretta cereoides, and Savignyella lafontii. Species living at moderate depths (circalittoral) are more abundant—notably represented by Cleidochasmidra portisi (40–130 m), Hornera frondiculata (30–130 m), Microporella verrucosa (50–120 m), and Smittina cervicornis (30–120 m). Only a few species (7 erect and 2 encrusting) indicate deeper water environments (lower circalittoral to upper bathyal; Figs 7 and 8). They have been chosen as bathymetric indicators (Fig. 4). Scarce accessory taxa (Adeonellopsis multiporosa, Pyripora catenularia, Smittoidea marmorea, and the fossil Ybselosoecia typica) also belong to this last group (Moissette and Spjeldnaes, Reference Moissette and Spjeldnaes1995; Moissette et al., Reference Moissette, Antonarakou, Kontakiotis, Cornée and Karakitsios2021).
Extant species constitute the vast majority of the bryozoans found in the Cape Arkhangelos sections. They live in the present-day Mediterranean and eastern Atlantic. Only 12 species are considered as fossil, including Anoterora persimplex, Celleporaria palmata, Phoceana pliocenica, Smittina canavarii, Teuchopora castrocarensis, and Ybselosoecia typica. A few of the shallow-water taxa mostly live in warm-water settings (e.g., Biflustra savartii, Emballotheca, and Metrarabdotos).
Nearly all deeper-water indicators, together with some accessory taxa, occur in abundance at the base of the Arkhangelos-1 section (samples 1–5; Fig. 7), indicating upper bathyal settings (although numerous littoral species/fragments and calcareous algae are also present). The succeeding samples (except sample 9) are much poorer in deeper-water species and contain more abundant shallow-water bryozoans. The upper part of the section is typified by scarce deep-water species, associated with a moderately diverse (except in sample 12 where only 3 species occur) assemblage of shallow-water species. However, the species Anguisia verrucosa (Fig. 5), which lives only in the deep sea in both the Mediterranean and the Atlantic (Fig. 4), occurs in multiple samples of the section, up to sample 16. Although the depth of deposition greatly decreased from the base to the top of the section, transportation of shallow-water bryozoans has consequently highly influenced the bryozoan content of the collected samples, with fauna transported from middle-inner neritic depths, even inner neritic near the top of the succession where gravels and coralline algae are fairly abundant. The preservation state of the relatively small number of fragments is generally poor and indicates transportation. Outer neritic to middle neritic environments are therefore inferred for the middle and upper parts of the section.
The Arkhangelos-2 section (Fig. 8) is characterized by the presence of relatively few deep-water indicators found only as relatively rare fragments in a few samples. Even the accessory taxa are represented only by the fossil Ybselosoecia typica, which gives barely approximate information on paleobathymetry. Shallow to moderate depths are otherwise indicated by the remaining species and the bryozoans are consequently not especially useful for the detailed study of this section.
Discussion
Essentially deep-water soft bottoms (clayey, more or less silty to sandy) are recorded in the studied material of the Arkhangelos-1 and Arkhangelos-2 sections. The quality of preservation of deep-water skeletal remains is generally high (Fig. 5), whereas allochthonous elements are commonly weathered and fragmented. Following tempests and seismic waves, nearshore sediments received abundant fossils and rock fragments (pebbles, gravels, and sands) from nearby cliffs or rocky bottoms. Most pebbles and gravels are rounded and show borings by clionaid sponges.
Moreover, transported gravels and rare pebbles, together with shells and other skeletal remains such as coralline algae are used as hard substrates (benthic islands) by invertebrates and calcareous algae. Seagrass and seaweeds were probably abundant in shallow-water environments. This is notably indicated by the occurrence of herbivorous gastropods in a number of beds. This coastal marine vegetation also plays an important role in the transport (horizontal and vertical) of skeletal organisms by floating and rafting.
%P/(P+B) values versus depth taxonomic indicators
Benthic species (i.e., benthic foraminifera) can be used for water depth estimates via transfer functions (e.g., Rossi and Horton, Reference Rossi and Horton2009; Milker et al., Reference Milker, Weinkauf, Titschack, Freiwald, Krüger, Jorissen and Schmiedl2017), although the error is relatively high in deeper waters. In addition, depth reconstructions based on the distribution of benthic organisms can be problematic because many species have a wide bathymetric range over which they can dwell. However, this study aims to overcome these challenges by combining species or groups of species with restricted depth ranges together with ratios between planktonic and benthic foraminifera, %P/(P+B), to estimate water depths. One of the main problems is differentiating between transported (allochthonous) and in-situ (autochthonous) fossil remains. The best criteria are to distinguish pristine from abraded specimens and to assess the ecological requirements of extant species. Eurybathic species predominate throughout both the Arkhangelos-1 and Arkhangelos-2 sections, but are accompanied by stenobathic species, which may have lived either in shallow- or deep-water environments. Moreover, the distinction between true bathyal and circalittoral–bathyal species is often difficult (a number of species having upper depth limits within the neritic zone), and most species show wider and deeper bathymetric ranges in the Atlantic Ocean than in the Mediterranean Sea (Fig. 4).
Consequently, bathymetric variations in the Cape Arkhangelos area appear difficult to estimate precisely because the proportion of transported specimens is notably high throughout most of the deposits and identifying in-situ deep-sea fauna is difficult. In addition, some of the bathymetric indicators lead to contradictory indications. Notably, bryozoan deep-water taxa remain generally uniformly rare, even in levels that are shown to have been deposited in the upper bathyal based on both %P/(P+B) values and benthic foraminiferal and mollusk taxa. The bathymetric variations are also complicated by the fact that while paleodepth estimates based on %P/(P+B) are consistent with the occurrences of deep-water fauna in the lower part of the Arkhangelos-1 section and throughout the Arkhangelos-2 section, the situation appears different in the middle and upper parts of the Arhangelos-1 section. There, paleodepths estimated based on %P/(P+B) values (Table 1) appear likely underestimated, probably because of transportation of shallow-water benthic foraminifera, even in sedimentary levels that were devoid of gravels and coralline algae, and which were therefore the least affected by transportation of allochthonous material.
Consequently, our study confirms that paleodepth estimates based on counts of planktonic foraminifera relative to the total number of foraminifera present issues when applied to deep-sea sediments deposited too close to rugged coastal landforms and indicates that such estimates should be correlated with other bathymetric indicators and parameters. Previous studies (e.g., Schröder-Adams et al., Reference Schröder-Adams, Boyd, Ruming and Sandstrom2008; Hayward et al., Reference Hayward, Sabaa and Triggs2019) have shown that the inclusion of gravitationally displaced foraminifera in the autochthonous foraminiferal assemblage of deeper parts of the basins alters the paleodepth reconstructions. During dynamic downslope transport, foraminiferal shells can undergo size sorting, abrasion, fragmentation, or dissolution. The distribution of foraminiferal shells in sediments is influenced by the interplay of factors such as foraminiferal production, sedimentation rate, ocean current transport, destruction (dissolution), and reworking of sediment through downslope transport by earthquakes and re-deposition (Schmuker, Reference Schmuker2000). Any index based on foraminiferal material is consequently susceptible to the processes mentioned above. Moreover, previous studies (e.g., Hayward et al., Reference Hayward, Sabaa and Triggs2019) have already attributed “anomalously” low percentages of planktonic foraminifera to downslope sediment transport. Therefore, the middle and upper parts of the Arkhangelos-1 section, which returned neritic paleodepths based on foraminiferal counts but yielded deep-sea taxonomic indicators, have been influenced by downslope transport resulting in outliers in the %P/(P+B) water-depth relationship.
Paleoenvironmental conditions in the Arkhangelos area
Upper bathyal paleoenvironmental conditions are first recorded at the base of the Arkhangelos-1 section (samples 1–5; Fig. 7). This is particularly evident in the benthic foraminifera and bryozoan assemblages, represented by relatively abundant deep-water species, and in the occurrence of deep-dwelling species of planktonic foraminifera. The mollusks, apart from Bathyarca pectunculoides and Delectopecten vitreus, are scarcer. Such an upper bathyal environment is consistent with the ~385 m paleodepth found in sample 3 (Table 1). In the middle part of the section (samples 6–10), deep-water indicators are absent or extremely rare in some levels, especially among the mollusks, but abundance of the bathyal to outer neritic species Kelliella miliaris can be common. The species of Dacrydium found in this part of the section (Fig. 5) resembles Dacrydium vitreum with some differences in the hinge morphology (see Salas and Gofas, Reference Salas and Gofas1997, figs. 2–6). In general, the species of Dacrydium live at depths below 200 m; only juveniles of D. hyalinum have been found at shallower depths on hard-bottom environments (Salas and Gofas, Reference Salas and Gofas1997). Benthic foraminifera and bryozoans are better represented, notably by species such as Cassidulina carinata, Melonis barleeanus, and Uvigerina peregrina (benthic foraminifera) or by Anguisia verrucosa, Palmicellaria elegans, Setosella vulnerata, Setosellina capriensis, and Tervia irregularis (bryozoans). Altogether, these assemblages characterize outer neritic paleoenvironmental conditions. The top of the section (samples 11–18) contains less-diverse and less-abundant deep-water indicators, although some deep-dwelling benthic foraminifera (e.g., Cibicidoides spp., Cassidulina carinata), bryozoans (Anguisia verrucosa), and mollusks (Dacrydium sp.) still occur. A middle neritic setting is therefore inferred.
The paleoenvironmental conditions of the Arkhangelos-2 section (Fig. 8) are not easily reconstructed based solely on the taxonomic depth indicators. Compared to Arkhangelos-1 (with approximately the same number of samples), and as previously noted for the bryozoans, fewer species are represented by fewer specimens in fewer samples. Planktonic foraminifera are rare in the lower part of the section (samples 1–4′), but the benthic foraminifera are more diverse and abundant, whereas the mollusks and the bryozoans are represented by relatively few species and specimens. The known depth ranges of the benthic foraminiferal and molluscan depth indicators, together with the lithological features (sandstones and pebble/gravel levels) observed at the base of the section (Figs. 3 and 8) indicate a slightly shallower water depth than above, probably in the outer neritic zone, in agreement with previous studies of the Kolymbia Formation (e.g., Moissette and Spjeldnaes, Reference Moissette and Spjeldnaes1995; Steinthorsdottir et al., Reference Steinthorsdottir, Lidgard and Hakansson2006). In the middle and upper parts of the section (samples 4″–13), all samples analyzed for their %P/(P+B) clearly indicate a depth in the upper bathyal zone (Table 1). The mollusks and bryozoans are poorly represented. The benthic foraminifera, which are characterized by fairly abundant specimens belonging to six species indicative of upper bathyal environments (Cibicidoides kullenbergi, C. ungerianus, Lenticulina spp., Melonis barleeanus, Uvigerina peregrina, Planulina ariminensis) in the middle part of the section, even exhibit scanty assemblages in its upper part. Altogether, these features indicate that deposition took place at upper bathyal depth and that it has been less influenced by the transportation of taxa from neritic settings.
Stratigraphic correlations and tectonic implications
Using calcareous nannofossil and planktonic foraminiferal biozones, it is possible to correlate the lower parts of the sections, from Zone CNPL7 to Zone CNPL8 (Fig. 9). Both sections are organized in an overall transgressive–regressive sedimentary cycle above a deformed basement, with the outer neritic deposits of the Kolymbia Formation changing upward into the upper bathyal deposits of the Lindos Bay Formation, and into the middle to inner neritic sediments of the Cape Arkhangelos Formation. A major maximum flooding surface can be recognized in both sections inside Subzone MNN19b (1.73–1.664 Ma) from the deepest faunal content found in both sections (Arkhangelos-1, sample 3; Arkhangelos-2, laminated marls between samples 5 and 6; Table 1). The age of this major maximum flooding event, which is also found elsewhere in Rhodes, was previously estimated to be ca. 1.4 Ma at Pefka (Milker et al., Reference Milker, Jorissen, Riller, Reicherter, Titschack, Weinkauf, Theodor and Schmiedl2019). It is now precisely restricted between 1.73 Ma and 1.664 Ma at Cape Arkhangelos. This result is consistent with the age obtained in the Lefkos Basin at Karpathos Island, 80 km southwest of Rhodes (Moissette et al., Reference Moissette, Cornée, Quillévéré, Zibrowius, Koskeridou and López-Otálvaro2017) where a major flooding event is also recorded during the 1.730–1.617 Ma interval, with upper bathyal coral buildups resting on a karstified basement. As a consequence, a major Early Pleistocene (early Calabrian) drowning occurred in the eastern Aegean forearc, suggesting a regional event linked to subduction process. In addition, considering a mean age of 1.7 Ma for the maximum flooding zone and the ages of Cornée et al. (Reference Cornée, Quillévéré, Moissette, Fietzke, López-Otálvaro, Melinte-Dobrinescu and Philippon2019) for the base of the Kolymbia (2 Ma) and top of the Cape Arkhangelos (0.4 Ma) formations, vertical motions can be roughly estimated to have occurred at Cape Arkhangelos during deposition of the Rhodes Synthem. From 2 Ma to 1.7 Ma, deposits were emplaced at upper bathyal depths of 200–600 m above an emergent basement. During this time interval, deposition was coeval with a minimum rate of drowning of 0.66 mm/yr and a maximum drowning rate of 2 mm/yr, with a mean of ~1.33 mm/yr. This is quite consistent with the rate of 1.7 mm/yr estimated southward at Pefka (Fig. 1) (Cornée et al., Reference Cornée, Quillévéré, Moissette, Fietzke, López-Otálvaro, Melinte-Dobrinescu and Philippon2019; Milker et al., Reference Milker, Jorissen, Riller, Reicherter, Titschack, Weinkauf, Theodor and Schmiedl2019). From 1.7 Ma to ca. 0.4 Ma, facies changed upward from upper bathyal to neritic, and to emergence. A minimum uplift rate of 0.14 mm/yr and a maximum of 0.43 mm/yr, with mean at ~0.28 mm/yr, can be estimated. This rate is much lower than those estimated in other areas of Rhodes (1.3 mm/yr; Cornée et al., Reference Cornée, Quillévéré, Moissette, Fietzke, López-Otálvaro, Melinte-Dobrinescu and Philippon2019). Consequently, the island was probably affected by differential uplift events between 2 Ma and 0.4 Ma, and by repeated, shorter-term, and possibly rapid vertical motions overprinted on the Early Pleistocene regional tectonically forced regressive trend, as already shown along its eastern coast by Milker et al. (Reference Milker, Jorissen, Riller, Reicherter, Titschack, Weinkauf, Theodor and Schmiedl2019) and Quillévéré et al. (Reference Quillévéré, Nouailhat, Joannin, Cornée, Moissette, Lécuyer, Fourel, Agiadi, Koskeridou and Escarguel2019). Noticeably, this average uplift rate is consistent with that calculated at Karpathos, estimated to be 0.27–0.49 mm/yr (Moissette et al., Reference Moissette, Cornée, Quillévéré, Zibrowius, Koskeridou and López-Otálvaro2017).
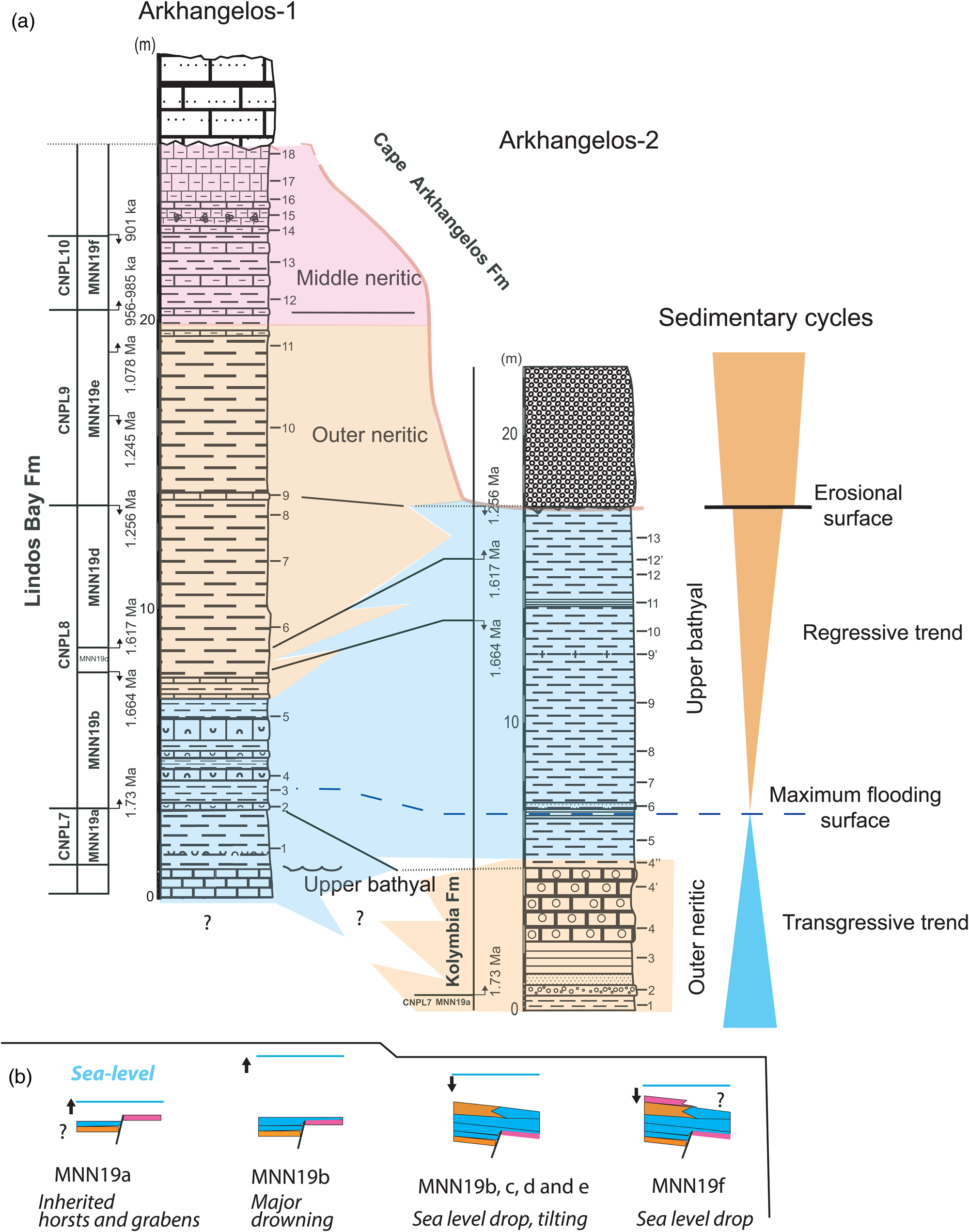
Figure 9. (a) Correlation scheme based on the litho- and biostratigraphy (with calcareous nannofossil bio-event calibrations of Raffi et al., Reference Raffi, Backman, Fornaciari, Pälike, Rio, Lourens and Hilgen2006) showing relationships between stratigraphic units of the study area and sedimentary organization. (b) Sedimentary evolution in the study area during the Early Pleistocene (Calabrian) within calcareous nannofossil Subzone intervals. MNN19a: transgression over paleo-relief, with bathyal deposition occurring within former grabens and middle neritic deposition occurring above horsts. Early MNNN19b: major flooding, the entire area was drowned in the upper bathyal zone. MNN19b to MNN19e: tectonically forced regression accompanied by a NW tilting of the area and by deposition of outer neritic deposits above bathyal deposits in the SE. MNN19f: continued regression and middle neritic deposition occurring in the southeastern part of the study area (Arkhangelos-1).
The investigated sections from the Cape Arkhangelos area are only about 300 m from each other but show different facies and thicknesses (Fig. 9). During the time interval corresponding to Zone/Subzone CNPL7/MNN19a, outer neritic sediments of the Kolymbia Formation laterally change to exhibit upper bathyal facies and components at Arkhangelos-1. This indicates that up to the LO of medium Gephyrocapsa at 1.73 Ma, the sediments of the Arkhangelos-1 section were deposited in a deep, previously existing paleovalley. Between 1.73 Ma and 1.664 Ma (i.e., during the time interval corresponding to Subzone MNN19b), deposition occurred in the upper bathyal at both locations, indicating a general drowning of the studied area. Finally, between 1.664 Ma and at least 1.256 Ma (i.e., subzones MNN19c and MNN19d), deposition occurred in different settings in the two sections. While deposition changed from upper bathyal to outer neritic at Arkhangelos-1, it remained upper bathyal at Arkhangelos-2. This indicates a northwestward tilting of the micro-basin, in accordance with tilting of the island of Rhodes during the major regression that finally led to the deposition of the Cape Arkhangelos Formation (van Hinsbergen et al., Reference van Hinsbergen, Krijgsman, Langereis, Cornée, Duermeijer and van Vugt2007; Cornée et al., Reference Cornée, Quillévéré, Moissette, Fietzke, López-Otálvaro, Melinte-Dobrinescu and Philippon2019).
Consequently, deposition of the Lindos Bay Formation has been controlled partly by tectonic processes: (1) abrupt facies changes resulted from an irregular topography of the calcareous basement during the Early Pleistocene (early Calabrian) transgression, (2) deposits then reached upper bathyal settings during the time interval corresponding to Subzone MNN19b, and (3) northwestward tilting and a regressive trend triggered differential depositional settings during the time interval corresponding to Subzone MMM19d. Later, paleovalleys were infilled by outer to middle neritic sediments, sealing the previous paleorelief irregularities in this part of the basin. Notably, the detrital materials (sands, gravels, pebbles) are consistent with this trend in both studied sections (Figs. 2 and 3) in which they are more abundant in the lower and upper parts of the sections than in their middle part.
Conclusions
During the Early Pleistocene, the paleo-landscape at Cape Arkhangelos comprised a Mesozoic basement of calcareous rocks, which was previously deformed and faulted. The area was divided into blocks responsible for abrupt facies changes during the Early Pleistocene (early Calabrian) transgression. Later, the whole area drowned to bathyal depths with concomitant deposition of hemipelagic sediments of the Rhodes Synthem (marls of the Lindos Bay Formation). These sediments exhibit atypical facies containing both autochthonous (deep-water organisms) and allochthonous (gravels and shallow-water organisms) elements. Finally, an uplift of the investigated area occurred, coeval with differential vertical movements that again triggered abrupt facies changes and then emergence. Our study shows that paleodepth estimates solely based on the number of planktonic foraminifera relative to the total number of foraminifera, %P/(P+B), can be severely biased in such hemipelagic sediments deposited at bathyal depths in the vicinity of coastal areas and steep paleorelief. Consequently, a complete inventory of the foraminifera, bivalve, gastropod, scaphopod, brachiopod, and bryozoan faunas was needed in order to precisely reconstruct the depositional settings. The differentiation between autochthonous and allochthonous fossil components was mostly based on extant species and genera, but also on a few morphological features. Despite a high abundance of fauna transported from coastal areas, deep-water index benthic taxa were found (as for example Cibicidoides kullenbergi, Melonis barleeanus, and Planulina ariminensis for the foraminifera; Dacrydium sp., Limatula sp., and Entalina tetragona for the mollusks; Anguisia verrucosa and Adeonellopsis distoma for the bryozoans), and combined with %P/(P+B) values that were estimated only in levels that were devoid of transported gravels and coralline algae. Maximum flooding within the Rhodes Synthem, which is patchily recorded along the eastern coast of Rhodes, is now precisely dated between 1.73 Ma and 1.664 Ma, which is consistent with the age obtained at the island of Karpathos, 80 km southwest of Rhodes.
Supplementary material
The supplementary material for this article can be found at https://doi.org/10.1017/qua.2024.19.
Acknowledgments
The authors thank Derek Booth and Louisa Bradtmiller, senior and associate editors of this paper. Yvonne Milker and two anonymous reviewers are also warmly thanked for their constructive comments on an early version of the manuscript. This work was funded by the National programs Tellus-INTERRVIE (FQ) and Tellus-SYSTER (JJC) of CNRS-INSU. The authors would like to acknowledge Konstantina Agiadi and Vasiliki Lianou for help during field work.