1. Introduction
Jasperoids consist of silica formed by metasomatic carbonate replacement from fluid–rock interaction (Lovering, Reference Lovering1962) in active geothermal systems. In continental settings, many geothermal systems are hosted in carbonate rock volumes that play the role of extensive reservoirs (Barbier, Reference Barbier2002; Faulds et al. Reference Faulds, Coolbaugh, Hinz, Cashman, Kratt, Dering, Edwards, Mayhew and McLachlan2011; Garland et al. Reference Garland, Neilson, Laubach, Whidden, Garland, Neilson, Laubach and Whidden2012; Brogi et al. Reference Brogi, Liotta, Ruggieri, Capezzuoli, Meccheri and Dini2016). Carbonate rocks, in fact, are prone to forming permeable volumes if interconnected fractures develop during their deformation (e.g. Agosta et al. Reference Agosta, Alessandroni, Antonellini, Tondi and Giorgioni2010). It occurs when carbonate rocks are involved in tectonic events at relatively low temperatures (i.e. in the upper crustal level), as in the case of extensional processes triggering detachment zones (Carmignani et al. Reference Carmignani, Decandia, Disperati, Fantozzi, Kligfield, Lazzarotto, Liotta, Meccheri, Vai and Martini2001; Brogi & Cerboneschi, Reference Brogi and Cerboneschi2007; Matera et al. Reference Matera, Ventruti, Zucchi, Brogi, Capezzuoli, Liotta, Yu, Shen, Huntington, Rinyu and Kele2021). In these geological contexts, the carbonate units can offer suitable secondary permeability to host significant volumes of geothermal fluids (Romagnoli et al. Reference Romagnoli, Arias, Barelli, Cei and Casini2010; Brogi et al. Reference Brogi, Liotta, Capezzuoli, Matera, Kele, Soligo, Tuccimei, Ruggieri, Yu, Shen and Huntington2020) and, depending on their chemical features, react with them, depositing neoformational minerals (i.e. banded calcite veins: Hancock et al. Reference Hancock, Chalmers, Altunel and Çakir1999; Capezzuoli et al. Reference Capezzuoli, Ruggieri, Rimondi, Brogi, Liotta, Alçiçek, Alçiçek, Bülbül, Gandin, Meccheri, Shen and Baykara2018; Brogi et al. Reference Brogi, Alcicek, Liotta, Capezzuoli, Zucchi and Matera2021) and jasperoids. Jasperoid formation is often associated with ore development mainly consisting of antimony and gold deposits (Dessau, Reference Dessau1952, Reference Dessau1977; Klemm & Neumann, Reference Klemm, Neumann, Wauschkuhn, Kluth and Zimmermann1984; Bagby & Berger, Reference Bagby, Berger, Berger and Bethke1985; Tanelli et al. Reference Tanelli, Lattanzi, Ruggieri, Corsini and Ladeira1991; Cline & Hofstra, Reference Cline and Hofstra2000; Cline et al. Reference Cline, Hofstra, Muntean, Tosdal, Hickey, Hedenquist, Thompson, Goldfarb and Richards2005; Sillitoe & Brogi, Reference Sillitoe and Brogi2021). This is the case for southern Tuscany where jasperoids consist of extensive stratabound volumes (Lattanzi, Reference Lattanzi1999; Brogi et al. Reference Brogi, Fabbrini and Liotta2011; Brogi & Fulignati, Reference Brogi and Fulignati2012; Morteani et al. Reference Morteani, Voropaev and Grinenko2017) confined between low-permeability units, developed in correspondence with cataclastic horizons formed during the Neogene–Quaternary extensional evolution of the inner Northern Apennines (Carmignani et al. Reference Carmignani, Decandia, Disperati, Fantozzi, Lazzarotto, Liotta and Meccheri1994; Brogi et al. Reference Brogi, Lazzarotto, Liotta and Ranalli2005). Nevertheless, the metasomatic process triggering the formation of jasperoids and Sb–Au deposition is the result of repeated pulses of fluid injection within the hosting rocks, a consequence of the tectonically controlled dynamic evolution of the geothermal system.
The study of chalcedony and agate generally addresses multiple objectives such as the identification of the sources of the elements, the characterization of the fluids and the reconstruction of the mobilization, transport, precipitation and depositional conditions. The study of chalcedony represents a flourishing research topic, prompting international interest. Several scholars have contributed to the knowledge of these materials, providing essential information on their composition, microstructure and banding (see, e.g. Heaney, Reference Heaney1993; Graetsch, Reference Graetsch, Heaney, Prewitt and Gibbs1994; Heaney et al. Reference Heaney, Veblen and Post1994; Heaney & Davis, Reference Heaney and Davis1995; Merino et al. Reference Merino, Wang and Deloule1995; Wang & Merino, Reference Wang and Merino1995; Götze et al. Reference Götze, Nasdala, Kleeberg and Wenzel1998; French et al. Reference French, Worden and Lee2003). Various studies have also been focused on the investigation of their genesis in volcanic (see, e.g. Götze et al. Reference Götze, Tichomirowa, Fuchs, Pilot and Sharp2001, Reference Götze, Möckel, Vennemann and Müller2016; Gilg et al. Reference Gilg, Morteani, Kostitsyn, Preinfalk, Gatter and Strieder2003; Moxon & Reed, Reference Moxon and Reed2006; Dumańska Słowik et al. 2008; Richter et al. Reference Richter, Götze, Niemeyer and Möckel2015) and sedimentary environments (see, e.g. Moxon & Reed Reference Moxon and Reed2006; Götze et al. Reference Götze, Möckel, Kempe, Kapitonov and Vennemann2009). Furthermore, the widespread distribution of chalcedony in correspondence with meso- to epithermal ore deposits has also favoured research on polymetallic (Sb, Zn, Pb, Au, Ag) vein-type ore deposits (see, e.g. Dill et al. Reference Dill, Melcher and Botz2008; Moreira & Fernandez Reference Moreira and Fernandez2015; Radosavljević et al. Reference Radosavljević, Stojanović, Radosavljević-Mihajlović and Vuković2016).
The studies performed on chalcedonies indicate that the deposition temperatures range from 20 to 230 °C (Harris, Reference Harris1989; Fallick et al. Reference Fallick, Jocelyn, Donnelly, Guy and Behan1985; Saunders, Reference Saunders1990; Heaney, Reference Heaney1993; Götze et al. Reference Götze, Möckel and Pan2020) and that, in sedimentary and low-temperature hydrothermal environments, near-surface conditions are the most favourable for their formation (see, e.g. White & Corwin, Reference White and Corwin1961; Cady et al. Reference Cady, Wenk and Sintubin1998). In the area under examination, a few studies performed on quartz fluid inclusions estimated homogenization temperatures ranging between 125 ± 3 and 232 °C (unpub. studies performed by Roedder on quartz from Poggio Fuoco, mentioned by Dessau et al. Reference Dessau, Duchi and Stea1972), between 140 and 232 °C (quartz from Pereta) or between 155 and 255 °C (quartz from Poggio Peloso in Brogi & Fulignati, Reference Brogi and Fulignati2012). These temperatures are close to those stated for stibnite precipitation, which has been constrained to between 132 and 245 °C by Lattanzi (Reference Lattanzi1999) and confirmed at 250 °C by Morteani et al. (Reference Morteani, Voropaev and Grinenko2017).
Following the work previously carried out in this area, this paper presents results of the study of the chalcedonies from the Pietratonda–Poggio Peloso Sb–Au epithermal mineralization (southern Tuscany, Italy; Fig. 1), which consists of an exhumed hot geothermal system developed during Quaternary time (Brogi & Fulignati, Reference Brogi and Fulignati2012). The main purposes of the study are: (1) to investigate the origin of antimony, gold and other elements present in the examined chalcedonies, by comparing the geochemical features and rare earth element (REE) patterns of the chalcedonies and selected lithotypes which may have reacted with the fluids that deposited the chalcedonies and, (2) to obtain information on the depositional environment of the chalcedonies. To these aims, optical and X-ray diffraction analyses were carried out and major, minor and trace elements were determined on six samples of chalcedonies and 11 samples from selected lithotypes, by laser ablation inductively coupled plasma mass spectroscopy (LA-ICP-MS) and X-ray fluorescence (XRF).
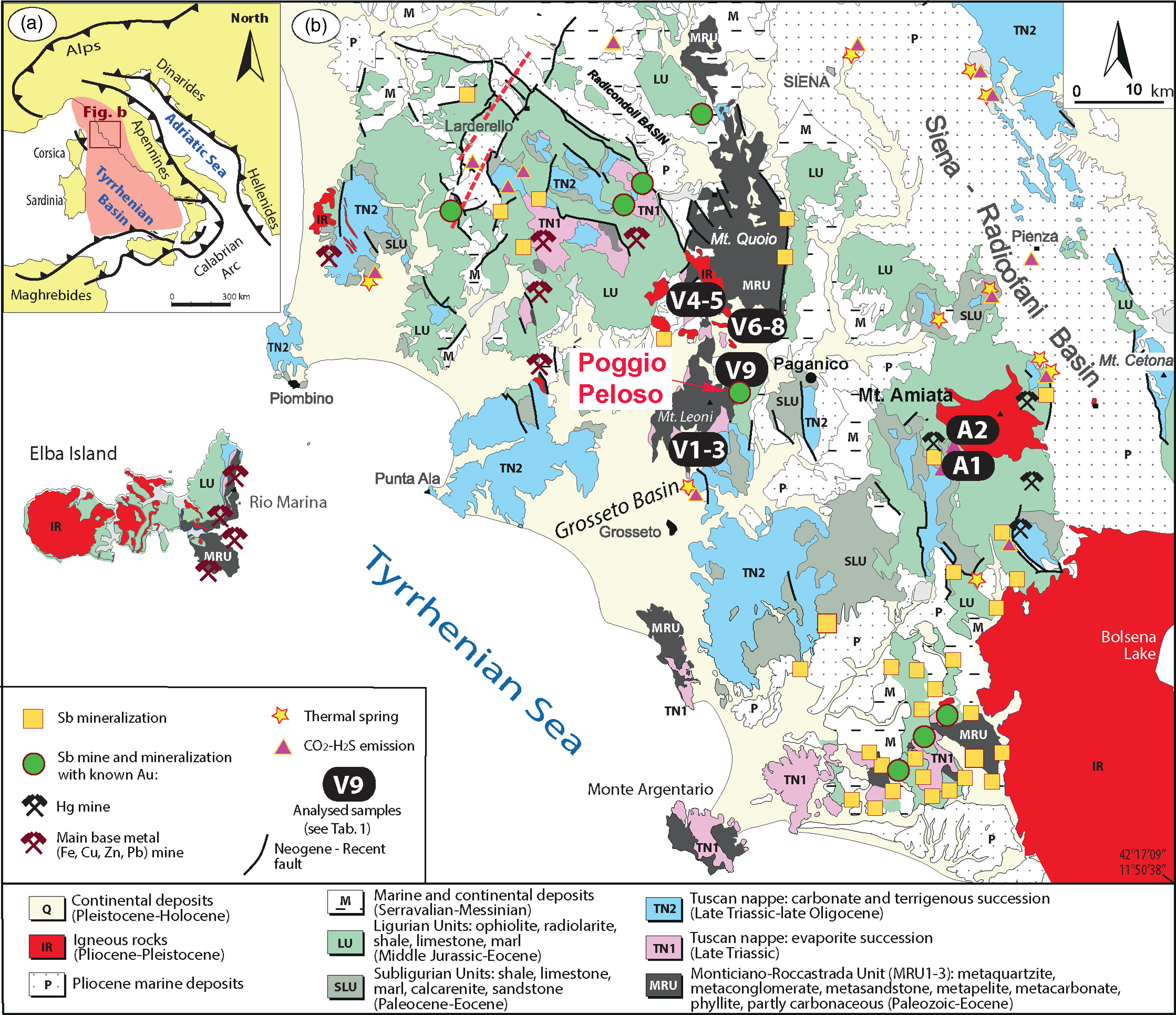
Fig. 1. The geographic distribution of Sb-mineralization in southern Tuscany and northern Lazio. The sampling sites are indicated by the name of the sample except for the chalcedonies (all taken from Poggio Peloso).
2. Geological outline
2.a. Southern Tuscany in the inner Northern Apennines
Southern Tuscany is part of the inner zone of the Northern Apennines orogen (Fig. 1), a Tertiary fold-thrust belt formed during collision between the Adria microplate (part of the African plate) and the Corsica–Sardinia massif (related to the European plate). Late Cretaceous through early Oligocene convergence of the African and European plates by subduction of the intervening western Tethys Ocean led to late Oligocene – early Miocene collision and eastward transport and emplacement of a thrust stack (see, e.g. Molli, Reference Molli, Siegesmund, Fügenschuh and Froitzheim2008). The Northern Apennines stack is formed, from the top down by (1) Jurassic–Cretaceous ophiolite and pelagic sedimentary cover of the Tethyan oceanic realm (Ligurian units, LU) and (2) Cretaceous–Oligocene turbidites representing the continent–ocean transition (Sub-Ligurian units, SLU), both of which were thrust in late Oligocene – early Miocene times over (3) the Upper Triassic to lower Miocene evaporite, carbonate, terrigenous and marine siliciclastic succession of the Adria passive margin (Tuscan Nappe, TN2). The evaporite level of the Tuscan Nappe is made up of partly de-dolomitized, anhydrite-dolostone breccia (known as the Calcare Cavernoso and Burano formations, TN1); its highly permeable and vacuolar texture is due to the interplay among different processes, such as cataclasis, anhydrite dissolution and karstification (see, e.g. Gandin et al. Reference Gandin, Giamello, Guasparri, Mugnaini and Sabatini2000). During early Miocene time, the Tuscan Nappe was thrust over (4) the polydeformed blueschist- to greenschist-facies (Giorgetti et al. Reference Giorgetti, Goff, Memmi and Nieto1998; Brogi & Giorgetti, Reference Brogi and Giorgetti2012) tectonic wedge complex (known as the Tuscan metamorphic complex; Pandeli et al. Reference Pandeli, Bertini and Castellucci1991) made up of phyllite and metaquartzite of Early to Middle Triassic age (Verrucano Group, MRU3), which overlies (5) phyllite and metacarbonate derived from Carboniferous–Permian protoliths (MRU2). MRU2 overlies (6) Precambrian to early Palaeozoic micaschist (MRU1) and (7) gneissic basement with Precambrian to early Palaeozoic protoliths. Locally, the thrusting caused interleaving of the Calcare Cavernoso and underlying metamorphic units, especially in the upper part of the tectonic wedge complex.
Beginning in early Miocene time, the previously stacked units were affected by extension (Carmignani et al. Reference Carmignani, Decandia, Disperati, Fantozzi, Kligfield, Lazzarotto, Liotta, Meccheri, Vai and Martini2001). Extension produced: (a) lateral segmentation of the previously stacked units and formation of bowl-shaped basins (Brogi & Liotta, Reference Brogi and Liotta2008; Brogi, Reference Brogi2011); and (b) development of NW-striking Pliocene–Quaternary normal faults, cross-cutting all the previous structures and inducing development of NW-trending tectonic depressions, filled by continental and marine sediments (Martini & Sagri, Reference Martini and Sagri1993; Brogi et al. Reference Brogi, Fidolini and Liotta2013; Martini et al. Reference Martini, Ambrosetti, Brogi, Aldinucci, Zwaan and Sandrelli2021). The primary evidence of extension is the opening of the Tyrrhenian Basin (Bartole, Reference Bartole1995) and the present crustal and lithospheric thicknesses of ∼17 and 40 km, respectively (Calcagnile & Panza, Reference Calcagnile and Panza1981; Locardi & Nicolich, Reference Locardi and Nicolich1982; Di Stefano et al. Reference Di Stefano, Bianchi, Ciaccio, Carrara and Kissling2011; Möller et al. Reference Möller, Grevemeyer, Ranero, Berndt, Klaeschen, Sallares, Zitellini and de Franco2013).
At least since Langhian time, extension was accompanied by eastward migrating magmatism (Serri et al. Reference Serri, Innocenti and Manetti1993). It affected the area corresponding to the Tuscan archipelago and the inland inner Northern Apennines (Serri et al. Reference Serri, Innocenti, Manetti, Vai and Martini2001; Dini et al. Reference Dini, Gianelli, Puxeddu and Ruggieri2005, Reference Dini, Westerman, Innocenti, Rocchi, Thomson and Petford2008). Magmas were emplaced at shallow crustal levels (6–8 km depth, mainly: Serri et al. Reference Serri, Innocenti, Manetti, Vai and Martini2001) mostly along NE-striking brittle shear zones playing the role of transfer zones (Dini et al. Reference Dini, Westerman, Innocenti, Rocchi, Thomson and Petford2008). Hot geothermal systems (T >200 °C) developed near the intrusive bodies and are now exposed in the whole Tuscan archipelago and southern Tuscany (e.g. Liotta et al. Reference Liotta, Ruggieri, Brogi, Fulignati, Dini and Nardini2010; Vezzoni et al. Reference Vezzoni, Dini and Rocchi2016; Zucchi et al. Reference Zucchi, Brogi, Liotta, Rimondi, Ruggieri, Montegrossi, Caggianelli and Dini2017; Zucchi, Reference Zucchi2020). Active systems are presently exploited at depth for geothermal energy production (cf. Liotta et al. Reference Liotta, Brogi, Ruggieri and Zucchi2021) in the Larderello and Monte Amiata areas (Batini et al. Reference Batini, Brogi, Lazzarotto, Liotta and Pandeli2003 and references therein).
2.b. The Pietratonda–Poggio Peloso area
The Pietratonda–Poggio Peloso area (Fig. 1) is mainly characterized by exposures of jasperoid that formed by the silica replacement of the basal Upper Triassic evaporitic horizon of the Tuscan Nappe (Bernoulli, Reference Bernoulli, Vai and Martini2001). This level tectonically overlies the Triassic continental metasiliciclastic succession of the Verrucano Group mainly consisting of quartzite and phyllite with metacarbonate and phyllite at the top (Meccheri et al. Reference Meccheri, Moretti and Volterrani1987; Moretti, Reference Moretti1991; Aldinucci et al. Reference Aldinucci, Brogi and Sandrelli2005), which recorded a polyphase tectonometamorphic evolution (Aldinucci et al. Reference Aldinucci, Brogi and Sandrelli2005; Brogi, Reference Brogi2006). At the top of the jasperoid level, discontinuous bodies made up of upper Oligocene – lower Miocene quartz–feldspar sandstone (Macigno Fm) occur. The contact juxtaposing the Macigno Fm on the jasperoid is tectonic and corresponds to a low-angle normal fault (Brogi, Reference Brogi2008). On top, Ligurian units and Miocene sediments formed the cap of the palaeo-geothermal system that was controlled by WSW–ENE strike-/oblique-slip and NNE–SSW normal faults, forming linkage zones between the main strike-slip fault segments (Brogi & Fulignati, Reference Brogi and Fulignati2012). The geothermal fluids consisted of meteoric water circulated to depth and heated owing to the geothermal anomaly. According to Brogi & Fulignati (Reference Brogi and Fulignati2012), these fluids reached temperatures of ∼200–225 °C and a maximum salinity of ∼3 wt % NaCl equiv.
2.c. Sb-mineralization and disseminated gold
Southern Tuscany has represented one of the most important mining districts of the Mediterranean basin from the Etruscan period to the twentieth century, with a limited time break during the Roman Empire. Deposits of pyrite, iron, lead, zinc and minor silver and gold are widespread and typically related to the activity of geothermal fluids associated with the Neogene–Quaternary magmatism (Tanelli, Reference Tanelli1983; Tanelli et al. Reference Tanelli, Lattanzi, Ruggieri, Corsini and Ladeira1991; Serri et al. Reference Serri, Innocenti and Manetti1993; Lattanzi, Reference Lattanzi1999; Peccerillo et al. Reference Peccerillo, Poli and Donati2001; Dini, Reference Dini2003; Peccerillo, Reference Peccerillo2003; Dini et al. Reference Dini, Gianelli, Puxeddu and Ruggieri2005; Boschi et al. Reference Boschi, Dini, Dallai, Ruggieri and Gianelli2009). The most significant Sb-ore sources are typically located along the NE–SW strip connecting Mount Amiata with Capalbio; however, further deposits are located north of the area of concentration (Fig. 1). The distribution of Sb-deposits shows common features throughout the area: (1) the distribution includes the peripheral zones of present and fossil geothermal areas, coinciding with a Sb mineralized belt; (2) they are associated with fluid flow channelled by extensional faults (Dessau et al. Reference Dessau, Duchi and Stea1972; Tanelli, Reference Tanelli1983; Tanelli & Scarsella, Reference Tanelli and Scarsella1990; Tanelli et al. Reference Tanelli, Lattanzi, Ruggieri, Corsini and Ladeira1991; Brogi et al. Reference Brogi, Fabbrini and Liotta2011; Brogi & Fulignati, Reference Brogi and Fulignati2012); (3) they are typically found at the contact between the Upper Triassic evaporite (‘Calcare Cavernoso’ Fm) and the overlying, impermeable, Ligurian and Sub-Ligurian units, which have played a fundamental role in fluid containment (Dessau et al. Reference Dessau, Duchi and Stea1972; Tanelli, Reference Tanelli1983; Tanelli & Scarsella, Reference Tanelli and Scarsella1990; Tanelli et al. Reference Tanelli, Lattanzi, Ruggieri, Corsini and Ladeira1991; Lattanzi, Reference Lattanzi1999); (4) they are characterized by silicic (jasperoid) and, subordinately, argillic alteration (Tanelli & Scarsella, Reference Tanelli and Scarsella1990; Tanelli et al. Reference Tanelli, Lattanzi, Ruggieri, Corsini and Ladeira1991).
The Sb-ore bodies were intensively exploited during the first half of the nineteenth century when at least 17 mines were active (Campiglia, Capita, Casal di Pari, Cetine, Macchia-Casella, Manciano, Montauto, Monticchio, Niccioleta, Pereta, Pietrarotonda–Salamagna, Poggio Fuoco, Rosia, San Martino sul Fiora, Selvena, Tafone, Zolfiere; list provided by Morteani et al. Reference Morteani, Voropaev and Grinenko2017). The deposition is still ongoing as testified to by the stibnite and metastibnite precipitation observed some years ago at the Pereta and Tafone mines (Dessau, Reference Dessau1952; Klemm & Neumann, Reference Klemm, Neumann, Wauschkuhn, Kluth and Zimmermann1984), as well as by the Sb- and As-rich geothermal fluids (0.2–1.3 mg/L Sb and 22.5–65.4 mg/L As) and the Sb-rich (75 %) scales in the wells (Morteani et al. Reference Morteani, Voropaev and Grinenko2017) and piping (Cappetti et al. Reference Cappetti, D’Olimpio, Sabatelli and Tarquini1995; Möller et al. Reference Möller, Morteani, Dulski and Preinfalk2009; Morteani et al. Reference Morteani, Ruggieri, Möller and Preinfalk2011) of the Piancastagnaio geothermal field and power plant, respectively.
The Sb-mineralization of Poggio Peloso hill was first documented by Rimbotti (Reference Rimbotti1884) but never exploited due to its low industrial potential (Brizzi & Sabelli, Reference Brizzi and Sabelli1985). Reported minerals are: quartz, as the main gangue mineral of Sb-bearing minerals, followed by calcite; stibnite (Sb2S3), in radial nodules of thin crystals (2–3 cm); stibiconite (Sb3O6(OH)), often pseudomorphic on stibnite; valentinite (Sb2O3), generally in spherules with a radius of a few millimetres; rare senarmontite (Sb2O3), showing small colourless octahedra; very rare onoratoite (Sb8O11Cl2); pyrolusite (MnO2), in the form of concretions of blackish powder; alunite (KAl3(SO4)2(OH)6), generally present as a white powder often associated with stibiconite; frequent barite (BaSO4), in tabular crystals typically below centimetre size; and Fe-hydroxides (Braga, Reference Braga1980; Brizzi & Sabelli, Reference Brizzi and Sabelli1985).
Brogi & Fulignati (Reference Brogi and Fulignati2012) described the Pietratonda–Poggio Peloso Sb-mineralization as ‘a fossil hydrothermal system that was probably active during the volcanic activity that produced rhyolitic lava flows’ of the neighbouring Roccastrada volcanic complex (∼2.3 Ma). What was the source of the Sb is still a matter of debate. Dessau et al. (Reference Dessau, Duchi and Stea1972) suggested that the metals had either a magmatic origin (direct) or that they were leached from the rocks crossed by the fluids (indirect). Lattanzi (Reference Lattanzi1999) suggested the existence of a distal ‘magmatic connection’ with the igneous rocks of the ‘Tuscan Magmatic Province’, even in the absence of a physical contact between the mineralization and the igneous bodies. On this basis, Brogi & Fulignati (Reference Brogi and Fulignati2012) speculated on the existence of a similar ‘magmatic connection’ between the Sb-deposits of Poggio Peloso and the Roccastrada volcanic complex.
Gold occurrences have long been associated with Sb- and Ba-deposits (Tanelli et al. Reference Tanelli, Lattanzi, Ruggieri, Corsini and Ladeira1991), and ‘disseminated’ gold has been found in this ore deposit (Pipino, Reference Pipino1988; Tanelli & Scarsella, Reference Tanelli and Scarsella1990; Tanelli et al. Reference Tanelli, Lattanzi, Ruggieri, Corsini and Ladeira1991; Lattanzi, Reference Lattanzi1999). Lattanzi (Reference Lattanzi1999) hypothesized that the igneous rocks of the Tuscan Magmatic Province may have been likely gold contributors, as well as the ophiolites of the Ligurian units as suggested by Montini et al. (Reference Montini, Lattanzi, Ruggieri, Maineri and Tanelli1995). The latter found a positive Au–Cr correlation in mineralized samples at Frassine (Monterotondo Marittimo, Grosseto) and observed that the only likely source of Cr in the area was the ophiolites occurring in the Ligurian units.
3. Materials
The list of investigated samples is provided in Table 1 and sampling sites are shown in Figure 1.
Table 1. Rock samples list

Abbreviations: Qz – quartz; wm – white mica; Chl – chlorite group; Cal – calcite; Dol – dolomite; Fsp – feldspars; Gp – gypsum; Opq – opaque minerals such as Fe- and Ti-oxides; sul – sulfates of the alunite-jarosite group. Further information on the mineralogical assemblage is provided in online Supplementary Material Note 1.
Six samples of chalcedonies were collected at Poggio Peloso (Fig. 2). All the chalcedonies showed the typical light blue colour, alternating to lighter (up to whitish) or darker (up to greyish) bands. Only sample PP5 showed light orange to light grey/blue bands. The hand specimens showed primary crustiform and colloform textures, while zonal marking was only present in sample PP5. Banding was generally parallel to the rim. Overall, these agates can be described as wall-lining and vein agates. The host rock was the Calcare Cavernoso (Triassic evaporitic Burano Fm) for all samples.

Fig. 2. The chalcedonies from Poggio Peloso investigated in this study.
Considering the various hypotheses formulated on the origin of antimony and gold, it was immediately clear that a sampling exclusively focused on chalcedonies would not have provided convincing answers. Therefore, samples of the carbonate base of the Tuscan Nappe (sample V9), the underlying Verrucano Group (samples V1–V3), the Upper Triassic evaporites (samples V4, V5) and the Palaeozoic phyllites (samples V6–V8) were also collected with the aim of determining their trace compositions. In addition, two samples of Palaeozoic metamorphic rocks drilled in the Monte Amiata geothermal area, consisting of graphitic quartz-metasandstone and metasiltite and graphitic phyllite, coming from the geothermal wells BG3bis (sample A2) and BG25 (sample A1), at depths of 3111 and 3663 m below the ground level, respectively, were also analysed for comparison. Sample A2 was collected in correspondence with a fractured volume (Ruggieri et al. Reference Ruggieri, Giolito, Gianelli, Manzella and Boiron2004) hosting the deeper (2500–3500 m below ground level) exploited geothermal reservoir (Batini et al. Reference Batini, Brogi, Lazzarotto, Liotta and Pandeli2003; Bertini et al. Reference Bertini, Cappetti and Fiordalisi2005).
4. Methods
4.a. LA-ICP-MS
LA-ICP-MS measurements were performed on flat-polished samples. The instrument combines an ablation microbeam based on a Nd:YAG laser source (Brilliant, Quantel) operating at 266 nm (for details see Tiepolo et al. Reference Tiepolo, Bottazzi, Palenzona and Vannucci2003) and a quadrupole ICP-MS (PerkinElmer Sciex-Elan DRC-e). Thirty-four masses from 7Li to 238U were acquired; the laser was operated at a 10 Hz repetition rate, the power on the sample was 1.5 mW and the spot size was set at 50 µm. The optimization of the LA-ICP-MS to minimize elemental fractionation was performed by ablating NIST 610 glass and adjusting the nebulizer Ar and the carrier laser cell He gas flows to obtain the ratio of 232Th and 238U signals close to 1 by minimizing the ThO+/Th+ ratio (<1 %) in order to reduce the formation of polyatomic oxides. Accuracy was assessed on the USGS BCR-2 reference glass (analysed as an unknown in each analytical run) and was better than 20 % at the sub parts per minute (ppm) level. Data reduction was carried out with the software package GLITTER (van Achterbergh et al. Reference van Achterbergh, Ryan, Jackson, Griffin and Sylvester2001) and using NIST SRM 610 and 29Si as external and internal standards, respectively.
4.b. X-ray fluorescence
Major and minor element compositions of the bulk rocks were determined by XRF. Samples were mechanically crushed in a planetary mill and manually ground into a powder in an agate mortar. Quantitative analyses were performed on powder discs obtained by pressing 0.5 g of sample on a support of boric acid. The XRF instrument was a Philips MagiX-Pro. Background and mass absorption intensities were calculated using calibrations based on 24 international geological reference materials. Loss on ignition was determined by heating samples to 1050 °C for 2 hours.
4.c. Optical microscopy
All chalcedonies were cut orthogonally to the banding and polished. Half of the polished sample was prepared in thin-section for optical microscopy (OM) observations, while the other opposite half was investigated by LA-ICP-MS. Rock sample nos V1–V9 were prepared in thin-section to evaluate the possible presence of microfeatures that could lead to their exclusion and to decide the points on which to conduct further analyses.
4.d. Powder X-ray diffraction
An aliquot of ∼20 g was crushed and pulverized in an agate mortar. About 1 g was used for powder X-ray diffraction (PXRD), carried out on an automated PANalytical X’Pert PRO diffractometer, using Cu–Ka radiation (Ni-filter, 2θ range = 5–70°, step size = 0.02°, time per step = 1 s, room temperature = 25 °C). Measurements were performed on powders gently pressed into a back-loading cavity mount. The phase analysis was carried out using the software X’Pert HighScore Plus that uses the Inorganic Crystal Structure Database (ICSD). The starting coordinates of the atoms, cell parameters and space groups were taken from Le Page & Donnay (Reference Le Page and Donnay1976) for quartz, Brigatti et al. (Reference Brigatti, Frigieri and Poppi1998) for phengitic muscovite, Walker & Bish (Reference Walker and Bish1992) and Guggenheim & Nelson (Reference Guggenheim and Nelson1993) for clinochlore, Kawano et al. (Reference Kawano, Shiraki and Tomita1998) for sericite, Aleksandrova et al. (Reference Aleksandrova, Drits and Sokolova1973) for sudoite, Neder et al. (Reference Neder, Burghammer, Grasl, Schulz, Bram and Fiedler1999) for kaolinite, Cole et al. (Reference Cole, Sörum and Kennard1949) for sanidine, Chao et al. (Reference Chao, Hargreaves and Taylor1940) for orthoclase and Ferguson et al. (Reference Ferguson, Traill and Taylor1958) for albite.
5. Results
5.a. The chalcedonies
5.a.1. LA-ICP-MS
The average composition of all samples is characterized by SiO2 contents ranging from 98.0 to 99.8 wt % (av. 99.1 wt % ± 0.3) and a rather low minor- and trace-element content, i.e. the typical composition of chalcedonies and agates (see, e.g. Blankenburg, Reference Blankenburg1988; Tanaka & Kamioka, Reference Tanaka and Kamioka1994; Merino et al. Reference Merino, Wang and Deloule1995; Götze et al. Reference Götze, Tichomirowa, Fuchs, Pilot and Sharp2001; Möckel & Götze, Reference Möckel and Götze2007; Götze et al. Reference Götze, Möckel, Kempe, Kapitonov and Vennemann2009, Reference Götze, Möckel, Vennemann and Müller2016). Some spots with low SiO2 and high Ca, Mg, Al, K or Fe contents were also identified as carbonates, feldspars and metal oxide inclusions. The presence of inclusions made it necessary to exclude some measurements (fully provided in the online Supplementary Material Tables S1–S6 and Figs S1–S6) from the calculation of the average composition of the chalcedonies provided in Table 2. Table 2 shows that SiO2 contents of the chalcedony range between 99.2 and 99.4 wt % and that none of the other minor and trace components is above 1000 ppm, except for Sb. The latter show contents (1543–1846 ppm; average 1697 ± 119 ppm) that greatly exceed those reported for the upper continental crust (UCC) (0.4 ppm; Rudnick & Gao, Reference Rudnick, Gao and Rudnick2003) or for surficial waters (<0.001 ppm) (Filella et al. Reference Filella, Belzile and Lett2007). To a lesser extent, B and Ge are also enriched, ranging on average between 81 and 113 ppm for the former and between 7 and 9 ppm for the latter. As for Ba contents, it is worth underlining that the amounts reported in Table 2 are underestimated. Indeed, numerous Ba-rich inclusions were found but omitted from the calculation of the average chalcedony composition.
Table 2. LA-ICP-MS data. The chemical composition of the chalcedony. Average values provided as ppm if not differently specified. Measurements of quartz and inclusions were omitted from calculation
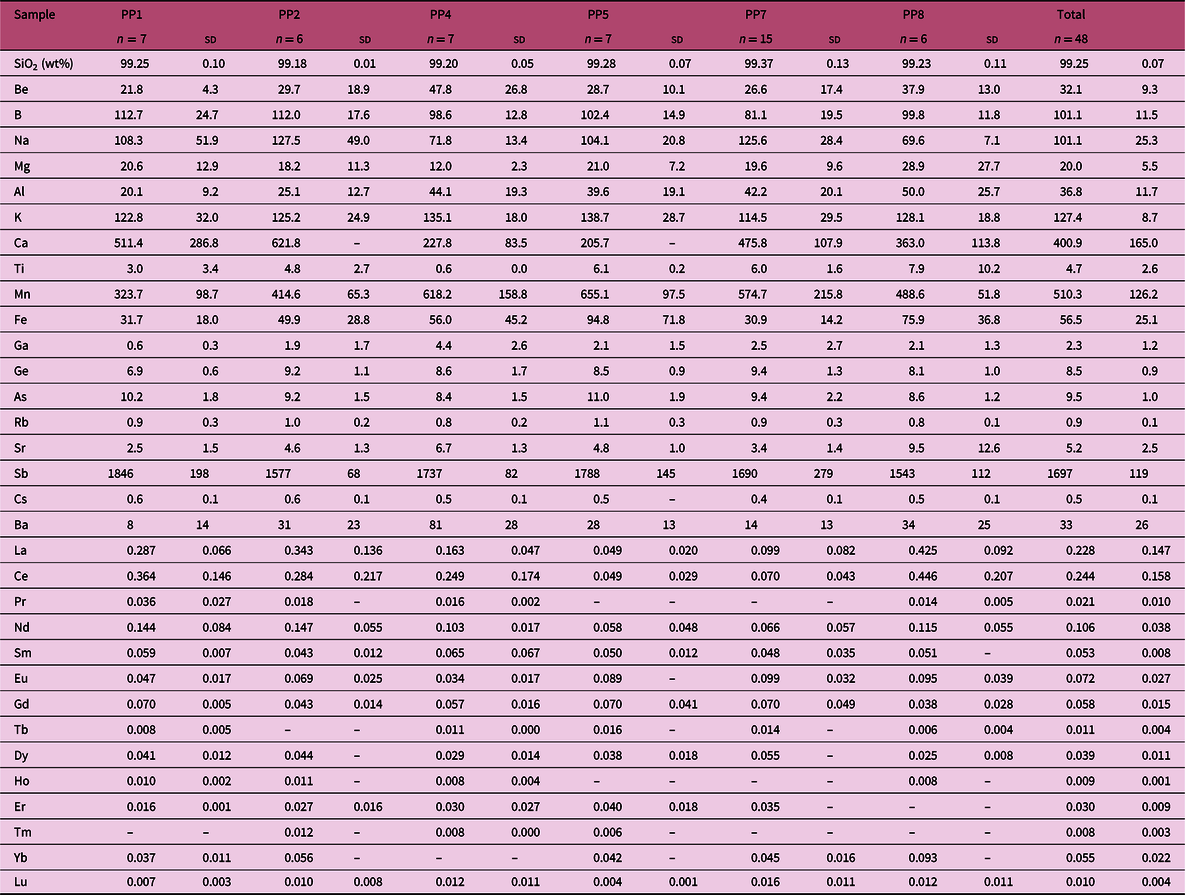
The correlations between elements and element pairs are shown in online Supplementary Material Figures S1–S6 and Tables S7–S12, respectively. Here the attention is focused on the correlation coefficients of Sb with major, minor and trace elements (Table 3) and on correlated pairs of elements (Table 4). Sb can be strongly correlated (r > 0.9) with B, Mn, As and Cs or well correlated (0.60 < r < 0.89) to poorly correlated (0.40 < r < 0.59) with all the remaining elements. The correlation with B and, to a lesser extent, Mn, As and Cs appears consistent among the different samples (Table 3; Fig. 3). On the contrary, the correlation with the other elements is only observed in a few samples. The well to strongly correlated pairs Be–Mn, Be–Ga, B–Rb, K–Rb, K–Cs, Rb–Cs and Ba–Sr, and the poorly correlated pair SiO2–Ge are worth noting (Table 4).
Table 3. Correlation coefficients (r) of Sb with major, minor and trace elements in the chalcedonies (LA-ICP-MS data)

Highest values are marked in bold.
Table 4. Correlated pairs of elements in the chalcedonies (LA-ICP-MS data)

Highest values are marked in bold.
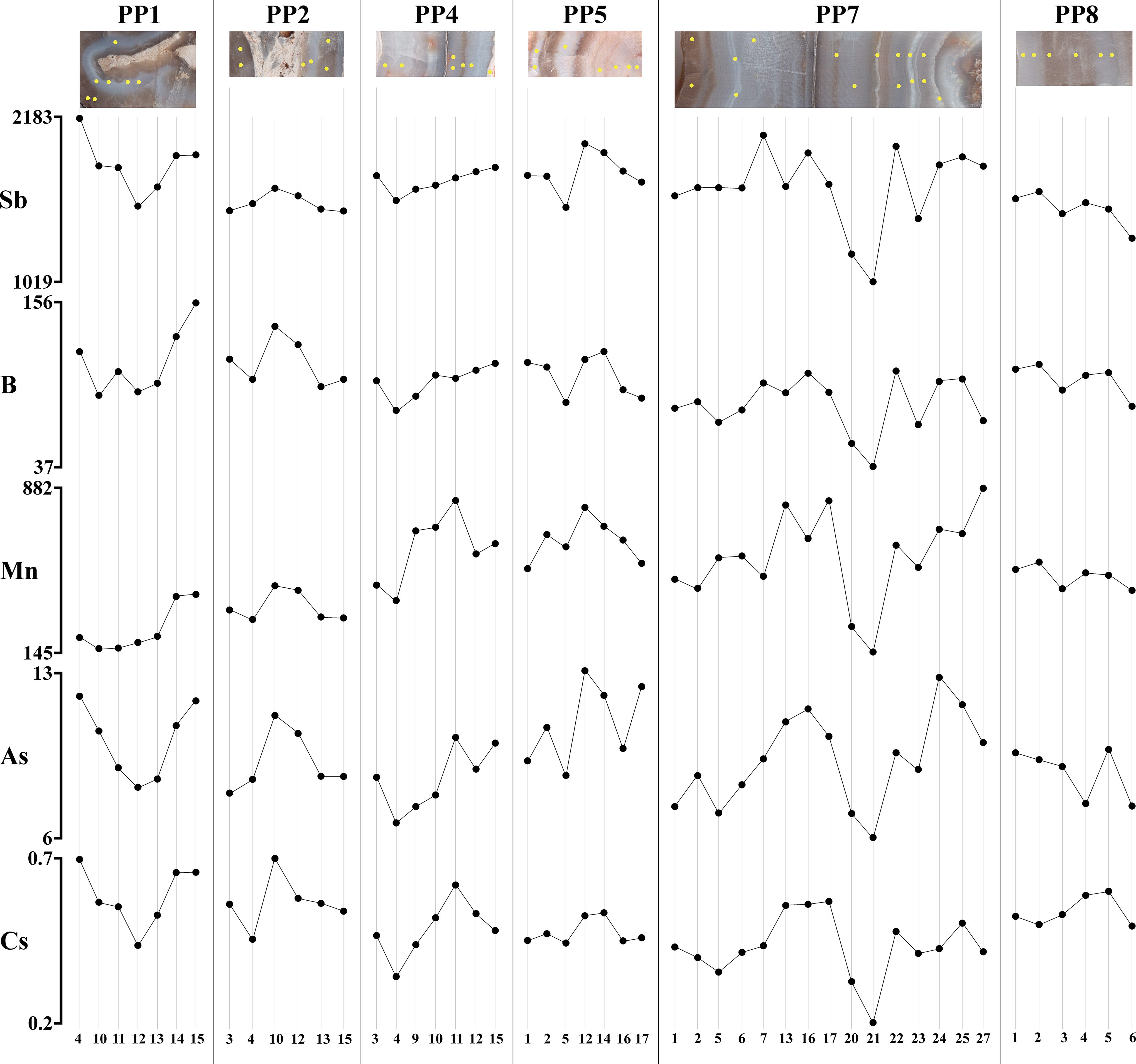
Fig. 3. LA-ICP-MS measurements. The Sb, B, Mn, As and Cs patterns of the chalcedonies from Poggio Peloso.
As for gold, despite that the values are to be considered qualitative, this element has been frequently detected, both in chalcedony and carbonate inclusions. The significance of its correlation is lowered by the small number of available measurements but appears consistent with Ag in sample PP7 (r = 0.865). As for the correlation between chromophores and agate colours, the techniques used are not suitable for proposing conclusive information. For example, (a) the variability of Fe and Mn amounts cannot be correlated to light/dark bands in the geochemical profiles of samples PP1, PP2, PP5, PP7 and PP8, and (b) the whitish and blue bands of sample PP4 show comparable contents of both Fe and Mn. Conversely, the brown band of the latter sample (online Supplementary Material Table S3, measurement no. 11) shows the highest Fe and Mn values.
As for REE contents, the chalcedonies show a depleted chondrite-normalized pattern (Fig. 4a), which is typical of these materials. Except for PP7 showing a Lan/Lun ratio below 1, light REEs (LREEs) prevail over heavy REEs (HREEs) in the other samples (Lan/Lun ratio 1.3–4.2). The LREE normalized patterns show a decreasing slope in all samples (Lan/Smn 1.3–5.2) except PP5 (Lan/Smn 0.6). A slight negative Ce anomaly is present in all samples (Ce/Ce* 0.57–0.94), except PP5 and PP7 for which it cannot be determined. Conversely, a weak to strong positive Eu anomaly characterizes all samples (Eu/Eu* 1.67–6.32). The HREE normalized patterns show an increasing slope (Gdn/Lun 0.4–0.6), except in PP1 and PP5 (Gdn/Lun 1.2 and 2.3, respectively). The UCC-normalized REE pattern (Fig. 4b) is particularly depleted and shows an enrichment of HREEs with respect to LREEs. Both Lan/Lun and Gdn/Lun ratios are constantly below 1 (range 0.06–0.76), as shown by the overall increasing slope of the patterns. The Ce anomaly is negative in all samples (Ce/Ce* 0.20–0.95) except PP4 (Ce/Ce* 2.05), while the Eu anomaly is always positive (Eu/Eu* 1.03–7.28).

Fig. 4. (a) The chondrite-normalized REE concentration pattern and (b) the upper continental crust (UCC)-normalized trace-element pattern of the chalcedonies (average values from Table 2). (c) The REE patterns of the metamorphic rocks. Black and grey lines indicate the samples investigated in this study. Red lines correspond to the Radicondoli (5.1960 m) drill core chip analysed by Möller et al. (Reference Möller, Dulski and Morteani2003) – indicated with ‘R’ – and the phyllites from the well Ciabattino 2 (305 m depth) and the Boccheggiano outcrops (sample nos 10179-B and 10179-D) analysed by Möller et al. (Reference Möller, Morteani, Dulski and Preinfalk2009). For comparison, the pattern of the chalcedonies is visible in the background. (d) REE pattern of selected Tuscanian volcanites compared to the chalcedonies. Reference data from Giraud et al. (Reference Giraud, Dupuy and Dostal1986) and Pinarelli et al. (Reference Pinarelli, Poli and Santo1989). The REE concentrations of the chondrite are from McDonough & Sun (Reference McDonough and Sun1995); those of the UCC are from Rudnick & Gao (Reference Rudnick, Gao and Rudnick2003).
5.a.2 . PXRD
Quartz was identified in all samples. Calcite has been clearly detected in sample PP2 while barite and haematite were rarely detected. The results of the XRD analyses performed on the rocks analysed for comparison are provided in the online Supplementary Material (Note 1) and confirmed the macroscopic identification of the sampled rocks.
5.a.3. Optical microscopy
The samples are mainly constituted by fibrous chalcedony (length-fast) and micro-/macro-crystalline quartz. In the following description, the correspondence with the spot-analyses (i.e. ‘S(no.)-no./nos’) refers to LA-ICP-MS measurements (online Supplementary Material Tables S1–S6). In sample PP1, long fibres of fan-shaped chalcedony with flamboyant extinction (S1- nos 14, 15) are richer in Mg, V, Mn, B, Na, K, Cs, As and Rb, and lower in Be, Al and Cu with respect to the thin parallel-bladed fibres (S1-nos 10, 11). Sb, Sr, Ba and Ga contents are similar in both types of textures. In sample PP2, the sequence includes several textures: fibrous flamboyant (S2-nos 1–4), comb, parallel-bladed (S2-nos 9, 10 and 13–15) and pseudo-granular (S2-nos 1, 11, 12, 16, 17). The last band of granular quartz (S2-nos 22, 23) contains the highest SiO2 and the lowest levels of almost all other elements, except for Mg, Ca and As. A thick and heterogeneous layer mainly constituted by calcite (S2-nos 5–8) is also present. Sample PP4 shows several generations of hatched bands (‘Runzelbänderung’), constituted by long and thin parallel fibres, alternating with thin layers of comb quartz. In sample PP5, the pseudo-granular texture (S4-nos 3, 4, 7–17) is generally poorer in most elements with respect to the flamboyant spherulites and fan-shaped fibres (S4-nos 1, 2, 5, 6). In sample PP7, the chemical composition varies without an apparent consistency among the different depositional layers. The layers show flamboyant (S5-nos 1, 2, 15–17, 22–27), parallel-bladed (S5-nos 3–8, 20–21) and feathery chalcedony (S5-nos 13, 18), along with comb (S5-nos. 14, 19) and granular quartz (S5-nos 9–12, 28, 29). Similarly, to the previous sample, PP8 also shows parallel-bladed (S6-nos 1, 2, 5, 6), flamboyant (S6-nos 3, 4, 7) fibres along with comb micro- and macro-quartz (S6-nos 8–10). The last layer is constituted by granular quartz and abundant residues of carbonaceous materials (S6-nos. 11, 12).
Regarding the distribution of the elements in the various layers, it may be interesting to note that while the antimony remains at almost comparable levels in all types of textures and generally decreases drastically in correspondence with carbonate inclusions, gold is present in chalcedony and carbonates while absent in comb quartz. A series of photos of the main textures (with a scale of detail greater than those given in the online Supplementary Material) is provided in Figure 5.

Fig. 5. The textures of chalcedony. OM images (crossed polarized light). (a) Parallel-bladed and comb textures. The arrows indicate two distinct layers of comb quartz. Along the lower one, a concentration of iron oxides approximately corresponds to the dark line indicated by the arrow. (b) Parallel-bladed texture. (c) Flamboyant texture. (d) Flamboyant and comb (indicated by the arrow). (e) Feathery quartz (jigsaw-puzzle texture). (f) Pseudo-granular. The scale bar equals 1 mm.
5.b. The rocks
The rocks were analysed only to confirm their identification and to estimate their Sb and Au contents; however, a complete analysis is provided for most of them in the online Supplementary Material (Note 1; Table S13).
The rocks are characterized by low to very low amounts of Sb: below 1 ppm in samples V1, V4, V5, V6, V7, V9 and R; between 1 and 2 ppm in samples V3, V8 and A1; and between 3 and 4 ppm in samples V2 and A2. Among the other elements, while minor and trace elements typically show higher values in the rocks than in the chalcedonies, the contents of Be and Ge appear systematically higher in the chalcedonies. The relatively high contents of B in samples V9 and A2, Mn in samples V9, A1 and A2, as well as the amounts of Au measured in samples V1, V7–V9, A2 and, especially, A1 may be informative for comparison.
Figure 4c shows the REE patterns of the examined rock samples and those of the Palaeozoic phyllites from the Larderello–Travale geothermal area analysed by Möller et al. (Reference Möller, Morteani, Dulski and Preinfalk2009). The first observation to make is that none of the rock samples show such pronounced depletion as that observed in the chalcedonies except V1. The Ciabattino and Boccheggiano samples appear particularly enriched compared to those investigated in this study, while the Radicondoli sample is comparable with both V6 and V7. The general trend of the phyllites is decreasing except in samples A1 and A2, which also show an increase in HREEs compared to LREEs. The different pattern of these two samples compared to the other rocks is probably due both to the partially different mineralogy (including the relative abundance of the accessory phases) and to the different migration speed of the HREEs compared to the LREEs in different conditions of alteration (in particular pH variability). As they fall beyond the scope of this research, we will not dwell on these aspects, while it is worth noting another important feature concerning the Eu anomalies. While the samples A1, A2 and V3 show a weak positive Eu anomaly (similar to the chalcedonies), the phyllites analysed by Möller et al. (Reference Möller, Dulski and Morteani2003, Reference Möller, Morteani, Dulski and Preinfalk2009) and the other metamorphic rocks investigated here are characterized by a negative Eu anomaly (Eu/Eu* 0.5–0.9). Furthermore, the samples A1 and A2 show a Lan/Lun ratio (0.8–1.2) closest to that of the chalcedonies.
In Figure 4d, the REE patterns of the chalcedonies are further compared with those of the Tuscan volcanites. The data obtained by Giraud et al. (Reference Giraud, Dupuy and Dostal1986) and Pinarelli et al. (Reference Pinarelli, Poli and Santo1989) on the volcanites from both Roccastrada and Monte Amiata evidence a clear difference not simply related to the absolute REE contents but also regarding the overall behaviour of the REEs.
6. Discussion
6.a. Poggio Peloso chalcedony: an outlier based on Sb contents
The samples from Poggio Peloso contain the highest Sb amounts found so far in chalcedonies. Table 5 shows a selection of reference data including chalcedony, carnelian and agate, as well as quartz, amethyst, chert/flint, jasper and quartzites from several locations. Low amounts of Sb are typically found in all silica-based minerals and rocks; however, the contents measured by the authors in agates rarely exceed 50 ppm.
Table 5. Sb amounts in silica-based minerals and rocks
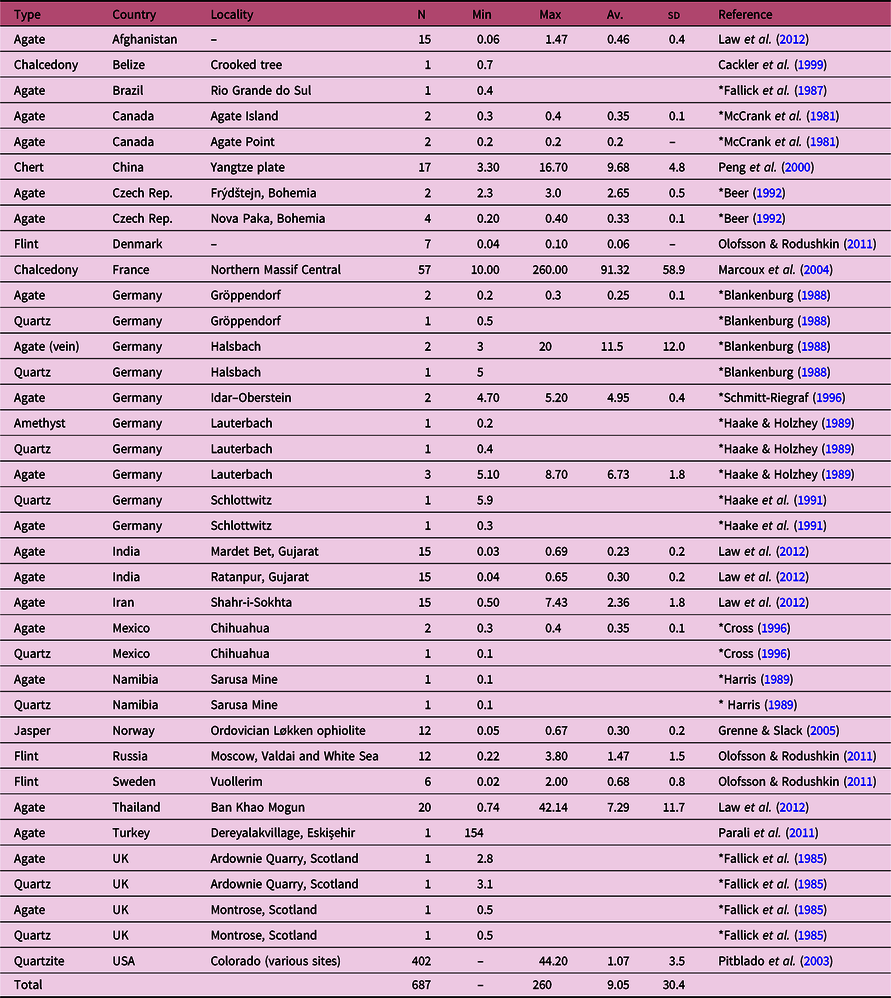
Abbreviations: N – number of analysed samples; Min. – minimum value; Max. – maximum value; Av. – average value; sd – standard deviation value
* From Götze et al. (Reference Götze, Tichomirowa, Fuchs, Pilot and Sharp2001).
Therefore, the comparison describes the chalcedonies of Poggio Peloso as ‘outliers’ with respect to Sb amounts, precisely as the high contents of Zr (between 295 and 687 ppm) found in vein agates from ‘Borówno’ quarry (Lower Silesia) distinguished the Polish samples (Powolny et al. Reference Powolny, Dumańska-Słowik, Sikorska-Jaworowska and Wójcik-Bania2019).
6.b. Positive Eu anomaly: hydrothermal environment
Comparing the REE patterns provided by Morteani et al. (Reference Morteani, Voropaev and Grinenko2017) for calcite with those obtained for the Poggio Peloso chalcedony, a striking difference emerges regarding Eu anomalies. While calcite patterns are characterized by negative Eu anomalies, the chalcedonies show positive anomalies. This result represents an anomalous feature even for chalcedonies, and it has been rarely observed (e.g. in vein and moss agates from the ‘Borówno’ quarry in Poland; Powolny et al. Reference Powolny, Dumańska-Słowik, Sikorska-Jaworowska and Wójcik-Bania2019).
As for calcite, Morteani et al. (Reference Morteani, Voropaev and Grinenko2017) explained the negative anomaly due to the poor Eu2+ uptake capacity of Ca minerals, based on the thermochemical reduction of Eu3+ to Eu2+ by high-temperature deep-seated aquifers. As regards the chalcedonies, it should be remembered that Eu2+ is present in a variety of magmatic and hydrothermal environments while it is rare in sedimentary environments because it is linked to particularly reducing conditions (Brookins, Reference Brookins, Lipin and McKay1989). In aqueous solutions, the redox potential of Eu/Eu* is affected by temperature and, to a lesser extent, by pH, pressure and REE speciation (Bau, Reference Bau1991). As a consequence, positive anomalies such as those observed in the chalcedonies are found in acidic, reducing hydrothermal fluids and sediments in an active ridge system (Kamber & Webb, Reference Kamber and Webb2001; Michard et al. Reference Michard, Albarède, Michard, Minster and Charlou1983; Michard, Reference Michard1989; German et al. Reference German, Klinkhammer, Edmond, Mitra and Elderfield1990). Furthermore, Eu enrichment may also reflect plagioclase weathering since Eu substitutes into the calcium site of plagioclases (see, e.g. Cabioch et al. Reference Cabioch, Camoin, Webb, Le Cornec, Garcia Molina, Pierre and Joachimski2006). However, Eu2+ can also substitute Ba2+ (Shannon, Reference Shannon1976; Guichard et al. Reference Guichard, Church, Truil and Jaffrezic1979; Mazumdar et al. Reference Mazumdar, Banerjee, Schidlowski and Balaram1999), thus making barite a possible carrier phase. In chalcedonies, barite inclusions are indicative of the activity of SO4 2− in the mineralizing medium, and Ba is one of the elements promoting silica precipitation in alkaline solutions (Dumańska-Słowik et al. Reference Dumańska-Słowik, Powolny, Sikorska-Jaworowska, Gaweł, Kogut and Poloński2018).
Möller et al. (Reference Möller, Morteani, Dulski and Preinfalk2009) reported the C1-chondrite-normalized patterns of REY in liquid and vapour produced by some wells of the Monte Amiata geothermal field. In particular, the liquid phase is characterized by strong Eu and moderate Y positive anomalies, which were interpreted to be the result of REY fractionation during water–rock interaction in the fluid source region. In particular, the deep fluid productive horizons of the Monte Amiata geothermal field are hosted in the Palaeozoic metamorphic rocks represented by the A1 and A2 samples. It is worth reiterating that such samples, contrary to the other Palaeozoic rocks, are characterized by a weak positive Eu anomaly.
6.c. Silica source and transport and Ge enrichment
The conditio sine qua non for the formation of chalcedonies is the availability of silica. Silica commonly derives from the weathering or hydrothermal alteration of silicate minerals (effective process) or from the dissolution of amorphous silica or of quartz (less effective process). As a consequence, chalcedony tends to form mainly in areas affected by thermal springs or in formations rich in weatherable silicates. Landmesser (Reference Landmesser1984) has shown that diffusion of monomeric silicic acid (H4SiO4) is the main mechanism in silica transport, based on numerous considerations including the large size/low velocity of colloidal silica and its clear prevalence under a wide range of pH conditions (1–9). This mechanism excludes the transport of silica via free liquid solution, which would also be difficult to explain compared to the low solubility of silica (Krauskopf, Reference Krauskopf1956).
Considering that the host rocks of the Poggio Peloso chalcedonies are carbonates, the high amounts of silica needed for the formation of chalcedony could have been derived from the volcanic and metamorphic rocks present in the territory or from residual magmatic fluids. Comparing REE contents in the surrounding rocks and chalcedonies, it is evident that the volcanic rocks have such different patterns (Fig. 4d) that it becomes difficult to invoke them as the primary silica source. Most of the metamorphic rocks are overall enriched with respect to the chalcedonies, but their patterns show different LREE and HREE trends (Fig. 4c). As shown above, an interesting analogy with chalcedonies is represented by the weak Eu anomaly shown by the A1–A2 samples from the Monte Amiata geothermal field. It is worth noting that the fluids produced from this geothermal field are characterized by significant concentrations of dissolved silica (up to 1160 ppm; Minissale et al. Reference Minissale, Magro, Vaselli, Verrucchi and Perticone1997) so that the brines resulting from flashing at the separator are highly supersaturated in silica, causing the formation of silica scales in geothermal pipelines (Vitolo & Cialdella, Reference Vitolo and Cialdella1995). Moreover, in one geothermal well, the high silica content is accompanied by significant Sb concentration (up to 50 mg/L), which caused the deposition of SiO2-rich, stibnite and/or metastibnite-bearing scales in the pipes (Morteani et al. Reference Morteani, Ruggieri, Möller and Preinfalk2011). However, the lack of a convincing direct correlation with any of the surrounding rocks suggests that the silica does not derive from the leaching of a unique Si-rich lithology.
The behaviour of Ge in chalcedonies has been frequently related to that of SiO2, given the similar geochemical behaviour of these two elements and the preferred structural incorporation of Ge in the quartz crystal structure (Götze et al. Reference Götze, Möckel, Kempe, Kapitonov and Vennemann2009). Ge contents measured in the chalcedonies from Poggio Peloso (7–9 ppm) are higher than those reported for the continental crust (1.4 ppm), lower than those shown by most volcanic agates, while slightly higher than those reported for sedimentary agates (Götze et al. Reference Götze, Möckel and Pan2020; Table 1). As noted in other case studies (e.g. Gliozzo et al. Reference Gliozzo, Cairncross and Vennemann2019), Ge contents are poorly (PP1, 2, 5, 7, 8) to well (PP4) correlated with those of SiO2; however, the absolute Ge amounts suggest the possible interaction of volatile fluids (HF) and the transport of SiF4, GeF4 and BF3 compounds (for this chemical transport reaction, see Götze et al. Reference Götze, Schrön, Möckel and Heide2012).
6.d. B, Mn, As and Cs and their correlations with Sb: the fluids
6.d.1. Boron
Contents ranging between 81 and 113 ppm are relatively high, both compared with the crustal B abundance (17 ppm) and the typical range of B content in quartz (from 0 to 25 ppm; Stavrov & Khitrov, Reference Stavrov and Khitrov1962), where B3+ may replace Si4+ in the SiO4 tetrahedra (see Müller et al. Reference Müller, Wanvik, Ihlen, Götze and Möckel2012 for details on the configuration of trace elements in the quartz lattice).
In magmatic systems, the incompatible behaviour of this lithophile element leads to its strong enrichment in magmatic fluids and vapours as well as in hydrous liquids. Hence, boron enrichment in hydrothermal fluids can derive from magmatic fluids, heating and feeding hydrothermal aquifers with volatile components. However, it can also be influenced by the geochemistry of the local rocks (water–rock interactions; see Bernard-Romero et al. Reference Bernard-Romero, Taran, Pennisi, Birkle and Torres-Alvarado2010) and therefore, being strictly conditioned by the hydrothermal circulation system itself. Among the surrounding rocks, the B-rich Triassic evaporites (Dessau et al. Reference Dessau, Duchi and Stea1972) may have represented the most abundant source of B. In this regard, however, it is worth noting that the A2 sample of the Monte Amiata geothermal field also contains remarkable B amounts (191 ppm) and that also the fluids produced by this field are characterized by significant B concentrations (up 3932 ppm; Minissale et al. Reference Minissale, Magro, Vaselli, Verrucchi and Perticone1997).
6.d.2. Manganese
The correlation of Sb with Mn was unexpected despite the testified presence of concretions of pyrolusite (blackish powder) in the area. Pyrolusite typically occurs in oxidation zones, mainly in sedimentary beds, and in Tuscany, its occurrence is typically related to supergene alteration. In the chalcedonies, manganese contents (324–655 ppm) are lower than those reported for the UCC (0.1 wt %; Rudnick & Gao, Reference Rudnick, Gao and Rudnick2003). In addition, they are often correlated with Be, which also shows high contents (22–48 ppm) with respect to both the continental crust and the chondrite composition. Be enrichment is frequent in jasperoids (e.g. Johnson, Reference Johnson1977; Lovering & Heyl, Reference Lovering and Heyl1980; McLemore, Reference McLemore, Lueth, Giles, Lucas, Kues, Myers and Ulmer-Scholle2002, Reference McLemore2010), and beryllium deposits often contain concentrations of manganese oxides together with fluorite, lithium, zinc, uranium and several trace elements (Lindsey, Reference Lindsey1977). Consequently, while the observed correlation between Mn and Be is not surprising, it appears more difficult to trace the origin of these two elements. The rocks investigated in this study bear low levels of Mn, except for the carbonates and the A1 and A2 metapelites. Therefore, it seems plausible to hypothesize that the high Mn contents of the chalcedonies mainly derive from the leaching of underlying carbonates. As regards Be enrichment, none of the surrounding rocks shows high levels of this element. However, no data are available on Be amounts contained by the Roccastrada rhyolites, which, in turn, could represent likely candidates. The conduits provided by faults should have then allowed beryllium to circulate, and a wall-rock reaction may have been essential for mineralization processes.
A not compelling but useful example to remember in this regard is the one offered by Lindsey (Reference Lindsey1977) for Spor Mountains and Honeycomb Hills (Utah). That is to say, carbonate-hosted beryllium (replacement) deposits genetically related to rhyolites (the only ones to return Mn-rich red beryl) and associated with hydrothermal silica, carbonates and other minerals. Moreover, it can be worth remembering that Be-bearing minerals such as bertrandite mainly occur in carbonate replacement deposits such as the jasperoids.
6.d.3. Arsenic
Arsenic enrichment has been frequently used to trace either epithermal gold mineralization (Henley, Reference Henley, Berger and Bethke1985; Berger & Silberman, Reference Berger, Silberman, Berger and Bethke1985; Silberman & Berger, Reference Silberman, Berger, Berger and Bethke1985) or geothermal activity (Bingqiu et. al. Reference Bingqiu, Jinmao, Lixin and Yaxin1986). Geothermal waters and sinters frequently bear high amounts of As, besides Sb and Hg (Weissberg, Reference Weissberg1969; Boyle & Jonasson, Reference Boyle and Jonasson1973). Furthermore, this element can be concentrated in hydrothermally altered metasediments (see, e.g. Codeço et al. Reference Codeço, Weis, Trumbull, Van Hinsberg, Pinto, Lecumberri-Sanchez and Schleicher2021) and in surficial conditions. In the latter environment, As may be released by Fe-hydroxides under oxidizing acid conditions which, in turn, can be obtained owing to sulfide decomposition (see e.g. Craw et al. Reference Craw, Chappell and Reay2000). In the investigated rocks, the highest concentrations of As (17–43 ppm) were measured in the metamorphic rocks (samples V2, V6 and V8). Consequently, the As in the chalcedonies may have been derived from magmatic/hydrothermal fluids and As-enriched meteoric waters.
6.d.4. Caesium
Cs, Rb and K (LFS elements) show a consistent correlation in several samples. In hydrothermal fluids, the alkali metals such as Rb and Cs remain in solution and are later incorporated into other phases such as micas (see, e.g. Codeço et al. Reference Codeço, Weis, Trumbull, Van Hinsberg, Pinto, Lecumberri-Sanchez and Schleicher2021). Accordingly, the metamorphic rocks, especially the metapelite A2, turned out to be the most Cs-rich rocks among those examined. The derivation of Cs from water–rock interaction with these rocks thus appears as plausible.
6.e. Gold and iron
As specified above, while the absolute quantities have to be considered with caution, the presence of Au in chalcedonies is evident and not surprising. Au leaching and transport require suitable redox conditions as well as the availability of suitable complexing agents (Mann, Reference Mann1984 ; Webster & Mann, Reference Webster and Mann1984; Stoffregen, Reference Stoffregen1986; Bowell et al. Reference Bowell, Foster and Gize1993; Freyssinet et al. Reference Freyssinet, Butt, Morris, Hedenquist, Thompson, Goldfarb and Richards2005). It is commonly believed that, at low temperatures (<400 °C), Au covalently bonds with bisulfide ligand (Wood et al. Reference Wood, Crerar and Borscik1987) and is then sequestered by sulfides (Cygan & Candela, Reference Cygan, Candela and Thompson1995; Jugo et al. Reference Jugo, Candela and Piccoli1999; Simon et al. Reference Simon, Kesler and Essene2000) while, at high temperatures (>350–400 °C), gold tends to form chloride complexes (Candela, Reference Candela and Rudnick2003). In the first case, the precipitation of gold can occur from the ore-fluids, for example, owing to fluid dilution or fluid–rock interactions, or from the achievement of oxidizing conditions that cause the breakdown of the sulfide complexes (Ilchik & Barton, Reference Ilchik, Barton, Vikre, Thompson, Bettles, Christensen and Parratt1997). In the second case, however, it is cooling that favours the precipitation of gold from gold-chloride solutions (Gammons & Williams-Jones, Reference Gammons and Williams-Jones1997).
In this regard, it is also worth mentioning the investigation by Williams-Jones & Normand (Reference Williams-Jones and Norman1997), according to whom Sb and Au jointly reach maximum concentrations when antimony is transported as HSb2S4 −. The same authors highlight how evident Sb–Au associations are reached when the transport takes place in an alkaline environment (such as limestones) and precipitation occurs in an environment suitable for the acidification of the waters (such as phyllosilicate-rich rocks). In this way, hydrogen ions are produced and can promote the deposition of gold and stibnite. Undoubtedly other processes are possible, but the similarity of the conditions described by Williams-Jones & Normand (Reference Williams-Jones and Norman1997) to those provided by the area under investigation make this reconstruction one of the most likely.
On the other hand, the absence of correlation of Au with iron seems to diminish (but not exclude) the possible importance of an association with Fe-oxyhydroxide, as often reported in the literature (Karasyova et al. Reference Karasyova, Ivanova, Lakshtanov, Lövgren and Sjöberg1998; Ran et al. Reference Ran, Fu, Rate and Gilkes2002; Cohen & Waite, Reference Cohen and Waite2004; Corral et al. Reference Corral, Corbella, Gómez-Gras and Griera2018). Moreover, in the territory under examination, the lack of a correlation between stibnite and pyrite occurrences is observed. As explained by Dessau (Reference Dessau1952), ‘pyrite and stibnite were deposited at the footwall and at the roof of the limestone, respectively, due to the chemical action, together with the drop in temperature and pressure, or due to a chronological shift for which pyrite should have been deposited in a “slightly earlier, higher temperature period”’. In a nutshell, the precipitation of pyrite and Sb–Au should be in succession. While the former lowers the amount of reduced sulfur in the ore fluid, the latter is favoured by a lowering of temperatures able to decrease Sb solubility and lead to Sb–Au precipitation. Finally, it may be interesting to recall the study performed by Nelson (Reference Nelson1990), which reports the range of Au concentration in jasperoids (up to 94 ppm) in US Carlin-type orebodies and traces the origin of gold to marine black-shales. Metamorphic rocks and chalcedonies are those bearing discrete Au amounts.
6.f. Textures
The various growth and textures observed in chalcedony testify to the physicochemical variability of the mineralizing solutions, chiefly in terms of SiO2 concentration and pH (Richter et al. Reference Richter, Götze, Niemeyer and Möckel2015). On the other hand, the feathery quartz (jigsaw-puzzle texture) observed in PP8 indicates recrystallization. Dong et al. (Reference Dong, Morrison and Jaireth1995) established that this texture forms at quartz stability temperatures (>180 °C) from the transformation of pre-existing silica phases such as amorphous silica or chalcedony (Lovering, Reference Lovering1972).
The presence of calcite layers in PP4 may indicate a process involving boiling, with consequent loss of CO2, generation of CO3 2− (from dissociation of HCO3 −) and precipitation of calcite (Henley & Brown, Reference Henley, Brown, Berger and Bethke1985; Reed & Spycher, Reference Reed, Spycher, Berger and Bethke1985). However, the analysis of fluid inclusions performed on other agates from Poggio Peloso by Brogi & Fulignati (Reference Brogi and Fulignati2012) did not retrieve evidence of boiling. Otherwise, calcite may have been generated by contact of cold fluids with hot rocks (retrograde solubility; see Simmons & Christenson, Reference Simmons and Christenson1994).
7. Conclusions
The chalcedonies of Poggio Peloso are the first Sb-rich chalcedonies reported in the literature. Not only is there no comparison among chalcedony and agate analysed to date, but not even the rocks present in the surrounding area have comparably high Sb levels. Antimony is strongly correlated with B, Mn, As and Cs in these chalcedonies. While the high presence of B and Mn may indicate the role of the fluids that, leaching the hosting carbonates, led to their replacement with chalcedony, As and Cs can be better associated with fluids interacting with metamorphic rocks.
The fluid transport in an alkaline environment agrees with the presence of carbonates, whereas silica precipitation in such an environment is possibly confirmed by features such as the abundance of barite inclusions. The latter may be further correlated with and explain the positive Eu anomaly observed in these chalcedonies (as well as in the Palaeozoic metamorphic rocks A1 and A2 from the Monte Amiata geothermal field).
The alkaline environment may also be indicated as responsible for the Sb–Au association, while the acidification of the waters promoted by metamorphic rocks or volatile fluids (HF) and SiF4, GeF4 and BF3 compounds (related to the abundance of Ge) should have favoured their precipitation.
Among the acidic components carried by the fluids, silicic acid must be included. For neither the latter nor any other constituent of these chalcedonies was it possible to draw a convincing correlation with the surrounding volcanic rocks. The comparison established on the basis of the REEs drew significant analogies only with the carbonate (B and Mn seen above) and metamorphic rocks. Among the latter, the Palaeozoic rocks (graphitic quartz-metasandstone and metasiltite and a graphitic phyllite) from two geothermal wells of the Monte Amiata geothermal field provided the most convincing comparison, also regarding the content of gold. In addition, some features of the geothermal fluids of this field (i.e. significant concentration of Sb, B and SiO2; positive Eu anomaly) seem to be similar to those of the fluid that deposited the chalcedonies. Thus, the latter fluid likely circulated in and reacted with metamorphic rocks analogous to those hosting the deep reservoir of the Monte Amiata geothermal field.
As for the deposition temperatures, it is possible to assume that they did not exceed the threshold of 225 °C, which was set as the upper term for the deposition of the Poggio Peloso quartz. In any case, the textures of the chalcedonies testify to the physicochemical variability of the mineralizing solutions and different precipitation. Therefore, they are representative of a diachronic process in which fluids with different characteristics have formed different generations of the silica phases.
Supplementary material
To view supplementary material for this article, please visit https://doi.org/10.1017/S0016756822001200
Acknowledgements
The authors would like to thank Enel Green Power S.p.A. for providing the well samples from the Monte Amiata geothermal field and the permission to publish the results. Author contributions: conceptualization, data curation, formal analysis, supervision: EG; investigation: EG, AB; resources: EG, AL; visualization: EG, AB; writing – original draft: EG, AB, GR. All data generated or analysed during this study are included in this published article and its supplementary information files.
Conflict of interest
None.