Introduction
Vitamin E is an important nutrient from the earliest stages of life (conception to post-uterine development)(Reference Lima, Dimenstein and Ribeiro1). Vitamin E is a group of fat-soluble compounds divided into tocopherols and tocotrienols (α, β, γ and δ). The α-tocopherol form has the highest biological activity and is widely available in foods(Reference Marquez, Yépez and Sútil-Naranjo2), in addition to being the most abundant form of vitamin E in human tissues(Reference Dror and Allen3). It is, therefore, a necessary nutrient for the prevention of symptoms of vitamin E deficiency (VED)(4).
Among the main functions of vitamin E, its important antioxidant role and protection of tissue lipids from attack by free radicals(Reference Fernández, Febles and Bernabeu5,Reference Jiang6) , which contributes to the maintenance of the integrity and stability of cellular structures, can be highlighted(7). In addition to its antioxidant activity, vitamin E presents other functions that are related to its action in vascular cells to limit the production of reactive oxygen species, inhibition of platelet aggregation(Reference Morrissey and Sheehy8), maintenance of the immune system by modulating the metabolism of prostaglandins and leukotrienes(Reference Marquez, Yépez and Sútil-Naranjo2), anti-inflammatory functions and modulation of cell signalling and genetic expression(Reference Dror and Allen3,4) . It is noteworthy that γ-tocopherol has pro-inflammatory functions in adults and mice(Reference Abdala-Valencia, Berdnikovs, Soveg and Cook-Mills9–Reference Berdnikovs, Abdala-Valencia, McCary, Somand, Cole, Garcia, Bryce and Cook-Mills15).
VED is rarely found in adults, but in children it occurs more frequently, since limited stocks of α-tocopherol are unable to maintain the rapid growth that occurs in childhood, thus allowing symptoms associated with deficiency to be identified more easily(Reference Traber16).
The clinical manifestations of VED involve neurological abnormalities. On the other hand, fat malabsorption syndrome and genetic defects in lipoprotein synthesis or affecting α-tocopherol transfer protein (α-TTP), in addition to severe malnutrition, may cause VED(Reference Traber17). VED is characterised by the presence of progressive peripheral neuropathy, ataxia, muscle weakness, a retinal injury that can lead to blindness (retinitis pigmentosa), infertility and dementia. Patients with autosomal recessive mutations in the α-TTP gene may present with cerebellar ataxia, loss of tendon reflexes, vibratory sensation disorders, dysarthria, muscle weakness and dystonia(Reference Dror and Allen3).
Vitamin E is a nutrient of recognised importance for health, but there are still gaps in our knowledge on the deficiency of this nutrient in childhood. A comprehensive, up-to-date review of vitamin E and its deficiency across child populations is therefore needed. Given the scarcity of bibliographic reviews evaluating VED with an emphasis on childhood, as well as the negative repercussions of this deficiency on the child’s health, this study aims to provide information on vitamin E, functions in the body, dietary sources, nutritional recommendations, metabolic aspects, clinical implications, and strategies for the prevention and control of VED in childhood, in addition to reviewing the existing data in the literature on the serum concentration of α-tocopherol in childhood and presenting the prevalence of VED in the world.
Vitamin E
Vitamin E refers to a group of fat-soluble compounds divided into tocopherols and tocotrienols (α, β, γ and δ). The α-tocopherol form has the highest biological activity, in addition to being the most abundant form of vitamin E in human tissues(Reference Dror and Allen3). Vitamin E consists of two main parts: a chromanol ring and a long sidechain(Reference Fernández, Febles and Bernabeu5). Tocopherols and tocotrienols differ from each other by the degree of methylation in the chromanol ring and by the configuration of the methyl group in the lateral chain, as shown in Fig. 1. Tocopherols have saturated side chains, and tocotrienols have three double bonds(Reference Jiang6).

Fig. 1. Chemical structure of tocopherols (A) and tocotrienols (B)(18).
The natural form of α-tocopherol occurs as RRR-α-tocopherol. The synthetic form of α-tocopherol, all-rac-α-tocopherol or dl-α-tocopherol, contains an equivalent mixture of eight stereoisomers. All stereoisomers have similar antioxidant activity, but only those with the 2R configuration have high biological activity (RRR-, RSR-, RSS- or RRS-)(4).
The half-life of RRR-α-tocopherol in plasma in healthy individuals is 48–60 h, while that of SRR-α-tocopherol and γ-tocopherol is approximately 15 h. Therefore, it is observed that the different forms of vitamin E, except α-tocopherol, are quickly removed from the body, while the α-tocopherol concentration is maintained(Reference Traber17).
The α-tocopherol form occurs naturally in food and has RRR stereochemistry in its side chains(18) with three asymmetric carbon atoms ligating them to the chromanol ring(Reference Azzi19). The α-tocopherol form has 100% biological activity, while the β-tocopherol form has half its activity (50%)(7). The γ-tocopherol form is present in foods in large quantities; however, its concentration in tissues is usually ten times lower than that of α-tocopherol(7). The preferential retention of α-tocopherol is based on the affinity of this vitamin for liver proteins (important in the metabolism and transport of tocopherols and tocotrienols) compared with other vitamers(Reference Jiang6).
Vitamin E functions
Vitamin E is considered an antioxidant nutrient and protects tissue lipids from attacks carried out by free radicals(Reference Fernández, Febles and Bernabeu5,Reference Jiang6) . It is abundant in biological membranes, where it protects polyunsaturated fatty acids from peroxidation within the phospholipid bilayer, and in plasma lipoproteins(18–20). Thus, vitamin E contributes to the maintenance of the integrity and stability of cellular structures(7). It is known that the hydroxyl radical reacts with the membrane to make peroxyl radicals, which can be quenched by vitamin E(Reference Hiroi, Ogihara, Hirano, Hasegawa, Morinobu, Tamai and Niki21) preventing the spread of chain reactions induced by free radicals(18,20) .
In addition to its antioxidant activity, vitamin E presents other functions related to its action in vascular cells: limitation of the production of reactive oxygen species, inhibition of platelet aggregation, protection against damage to DNA(Reference Morrissey and Sheehy8), maintenance of the immune system by modulating the metabolism of prostaglandins and leukotrienes(Reference Marquez, Yépez and Sútil-Naranjo2), anti-inflammation, and modulation of cell signalling and genetic expression(Reference Dror and Allen3,4) . Reduced levels of vitamin E lead to destabilisation of cell membranes of the immune system, decreased delayed hypersensitivity, decreased production of immunoglobulins, decreased cell-mediated immunity, and interleukin-2 production(Reference Fernández, Febles and Bernabeu5).
The α-tocopherol form can modulate the activity of various enzymes, including those affecting cell proliferation, membrane transport and xenobiotic metabolism(4). In addition, vitamin E acts in the apoptosis process, responsible for the elimination of tumour cells and the maintenance of the structural integrity of membranes and organelles, reduction of cell volume, chromatin condensation, nuclear fragmentation and cell fragmentation in the form of apoptotic bodies(Reference Fernández, Febles and Bernabeu5).
Food sources
Tocopherols and tocotrienols (α, β, γ and δ) are naturally present in food, albeit in different amounts (Table 1). Foods containing vitamin E are edible nuts and seeds, wheat germ, whole grains, dark-green vegetables, vegetable oils(Reference Marquez, Yépez and Sútil-Naranjo2,Reference Traber16) and egg yolk(24). The α-tocopherol form is predominantly found in Brazil nuts, hazelnuts, almonds, pistachios(22), sunflower oil(7), canola oil(6) and peanuts(24), while γ-tocopherol is found in pistachios; Brazil nuts(Reference Freitas and Naves22); maize, soybean and canola oils(7); and walnuts(Reference Jiang6).
Table 1. Amount of tocopherols (mg/100 g) in food

Legend: data not shown are represented by ‘–‘.
Source: Jiang(Reference Jiang6); WHO(7); Freitas; Naves(Reference Freitas and Naves22); IBGE(23).
The largest dietary source of vitamin E is edible oils(Reference Traber17). Vegetable oils contain varying amounts of tocopherols and tocotrienols. Sunflower, maize, olive and soybean oils contain high amounts of α-tocopherol. Maize and soybean oils contain a high amount of γ-tocopherol, and soybean oil also presents appreciable amounts of δ-tocopherol(4,Reference Jiang6) . Animal products contain a low amount of vitamin E (Table 1)(Reference Marquez, Yépez and Sútil-Naranjo2).
Although vitamin E is used in a wide variety of food preparations, its deficiency is still observed in the population, which is an important factor to be studied in future research.
Nutritional recommendations and food intake
Vitamin E intake in recommended amounts is essential for this nutrient to perform its functions in the body. The Adequate Intake of vitamin E, according to the Dietary Reference Intakes, for children between birth and 6 months of age is 4 mg/d (9·3 μmol) of α-tocopherol. The Adequate Intake is 5 mg/d (11·6 μmol) for children aged 6–12 months and 6 mg/d (13·9 μmol) for children aged 1–3 years(18).
Dietary Reference Intakes also establish the Estimated Average Requirement of 5 mg/d (11·6 μmol) of α-tocopherol for children aged 1–3 years. The Tolerable Upper Intake Level of vitamin E for children up to 12 months of age has not been established; for children aged 1–3 years, it is 200 mg/d of α-tocopherol(18).
A balanced diet in quantity and nutritional quality allows the recommended intake of nutrients for children up to 2 years of age to be achieved, thus avoiding the development of this nutritional deficiency, in addition to preventing other future diseases(25). These vitamin E recommendations are estimates for healthy children, but the levels needed during inflammation are not known.
Although it is a natural component of food, vitamin E intakes from food sources in pre-schoolers (2–6 years) and school children (7–10 years) are low(Reference Bueno, Fisberg and Maximino26–Reference Demmer, Cifelli and Houchins31). Bueno et al.(Reference Bueno, Fisberg and Maximino26) evaluated children aged 2–3 years from public and private schools in nine Brazilian cities and observed approximately 15% inadequate vitamin E intake. In a study conducted in philanthropic daycare centres in the Federal District (Brazil), it was observed that only 25·8% (n = 8) of children aged 4–12 months had adequate vitamin E intake(Reference Gomes, Costa and Schmitz27). Luna et al.(Reference Luna, Nascimento and Bandeira28) and Paiva-Bandeira et al.(Reference Paiva-Bandeira, Rios-Asciutti and Riviera29) demonstrated in a study conducted in João Pessoa (Brazil) that 41·7% of children between 2 and 3 years of age had vitamin E intake below the recommendation for this age group.
In a study conducted in the Republic of Korea in 2008, it was observed that 77% of children aged 2–6 years had dietary α-tocopherol intakes below the nutritional recommendations for this nutrient(Reference Giraud, Kim and Cho30). Demmer et al.(Reference Demmer, Cifelli and Houchins31) evaluated the dietary intakes of US children of different ethnicities from birth to 5 years of age. Twenty-four-hour recalls of the National Health and Nutrition Examination Survey (NHANES) 2011–2014 were used, which allowed the evaluation of the prevalence of inadequate nutrient intake through Estimated Average Requirement. The authors observed a high percentage of vitamin E intake below the recommended level in the children evaluated (66% in the 12–23-month age group). These data demonstrate that low vitamin E intake is a reality present in both Brazilian children and those from other countries. Regular intake of food sources of vitamin E becomes, therefore, essential at this stage of life.
Metabolic aspects of vitamin E
The process of vitamin E absorption depends on the same factors of digestion and absorption of lipids; for this, the presence of bile salts and pancreatic enzymes is essential for the formation of micelles and absorption by the intestine(Reference Marquez, Yépez and Sútil-Naranjo2,Reference Dror and Allen3,Reference Traber17) . Micelles, composed of hydrolysed lipids, dietary fat and bile secretion products, are formed in the intestinal lumen and contribute to the absorption of vitamin E(Reference Kayden and Traber32).
The estimated absorption of vitamin E in relation to the amount ingested varies between 33% and 70%(Reference Marquez, Yépez and Sútil-Naranjo2,Reference Dror and Allen3) . When tocopherol is consumed in larger amounts, its absorption is less efficient(Reference Marquez, Yépez and Sútil-Naranjo2).
The bioavailability of vitamin E can be influenced by several factors, such as the origin and amount of vitamin E ingested(Reference Schmolz, Birringer and Lorkowski33), the presence of quelant, proteins involved in the absorption of vitamin E, individual differences in the absorption process(Reference Schmolz, Birringer and Lorkowski33), vitamin E metabolism(Reference Schmolz, Birringer and Lorkowski33), lifestyle(Reference Schmolz, Birringer and Lorkowski33), sex(Reference Schmolz, Birringer and Lorkowski33), genetic polymorphisms(Reference Schmolz, Birringer and Lorkowski33–Reference Lodge, Hall and Jeanes35), the amount of fat from the diet and food matrix(Reference Dror and Allen3,Reference Borel, Preveraud and Desmarchelier34–Reference Jeanes, Hall and Ellard36) , the activity of digestive enzymes, which depends on the nutritional status of the individual(Reference Borel, Preveraud and Desmarchelier34), and the transport capacity of the intestinal cells(Reference Borel, Preveraud and Desmarchelier34). In addition, the bioavailability of vitamin E(Reference Hollander and Dadufalza37) and α-tocopherol concentrations in rats increase with age(Reference Leonard, Traber and Harrington38–Reference Traber, Leonard and Ebenuwa40). On the other hand, the bioavailability of vitamin E in rats is reduced by high intakes of pectin(Reference Schaus, Lumen and Chow41) and wheat bran(Reference Omaye and Chow42). Foods sources of lipids, such as vegetable oils, increase the absorption and bioavailability of vitamin E(Reference Borel, Preveraud and Desmarchelier34,Reference Bates and Heseker43) .
α-TTP is responsible for maintaining and regulating the concentrations of plasma α-tocopherol and is present in the liver(Reference Leonard, Traber and Harrington38,Reference Traber, Sokol and Burton44) . The preferential affinity of α-TTP for α-tocopherol is considered a determining factor for the plasmatic concentration of this nutrient(Reference Traber17). Mutations in α-TTP can lead to severe VED, characterised by neurological abnormalities and even death if vitamin E is not supplied in sufficient quantities to supply α-TTP(Reference Niki and Traber45).
Following ingestion, vitamin E is transported to the intestinal mucosa and, once inside the intestinal lumen, is absorbed through the enterocytes, bound in micelles and incorporated into chylomicrons, which are transported to the plasma via the lymphatic circulation(Reference Dror and Allen3,Reference Traber17) . A small portion of vitamin E is transported directly into the portal circulation(Reference Marquez, Yépez and Sútil-Naranjo2,Reference Traber17) . Tocopherols are distributed in the plasma by lipoproteins. Most vitamin E is present in human plasma in LDL and HDL(Reference Marquez, Yépez and Sútil-Naranjo2).
Lipoprotein lipase hydrolyses the triglycerides present in chylomicrons to release vitamin E to tissues such as muscles, adipocytes and the brain. As the remnants of chylomicrons are formed, surface components, including vitamin E, are preferably transferred to HDL and subsequently to other lipoproteins in the circulation(Reference Dror and Allen3).
Vitamin E is transferred to the liver via the remaining lipoproteins and chylomicrons. In the liver, α-TTP captures RRR-α-tocopherol and facilitates its incorporation into VLDLs, with the aid of other transport proteins, such as ATP-binding protein A1 (ABCA1)(Reference Dror and Allen3).
After the release of VLDL from the liver, α-tocopherol is redistributed to other lipoproteins for transport to peripheral tissues (Fig. 2). Adipocytes and skeletal muscle have the capacity for vitamin E storage(Reference Dror and Allen3).
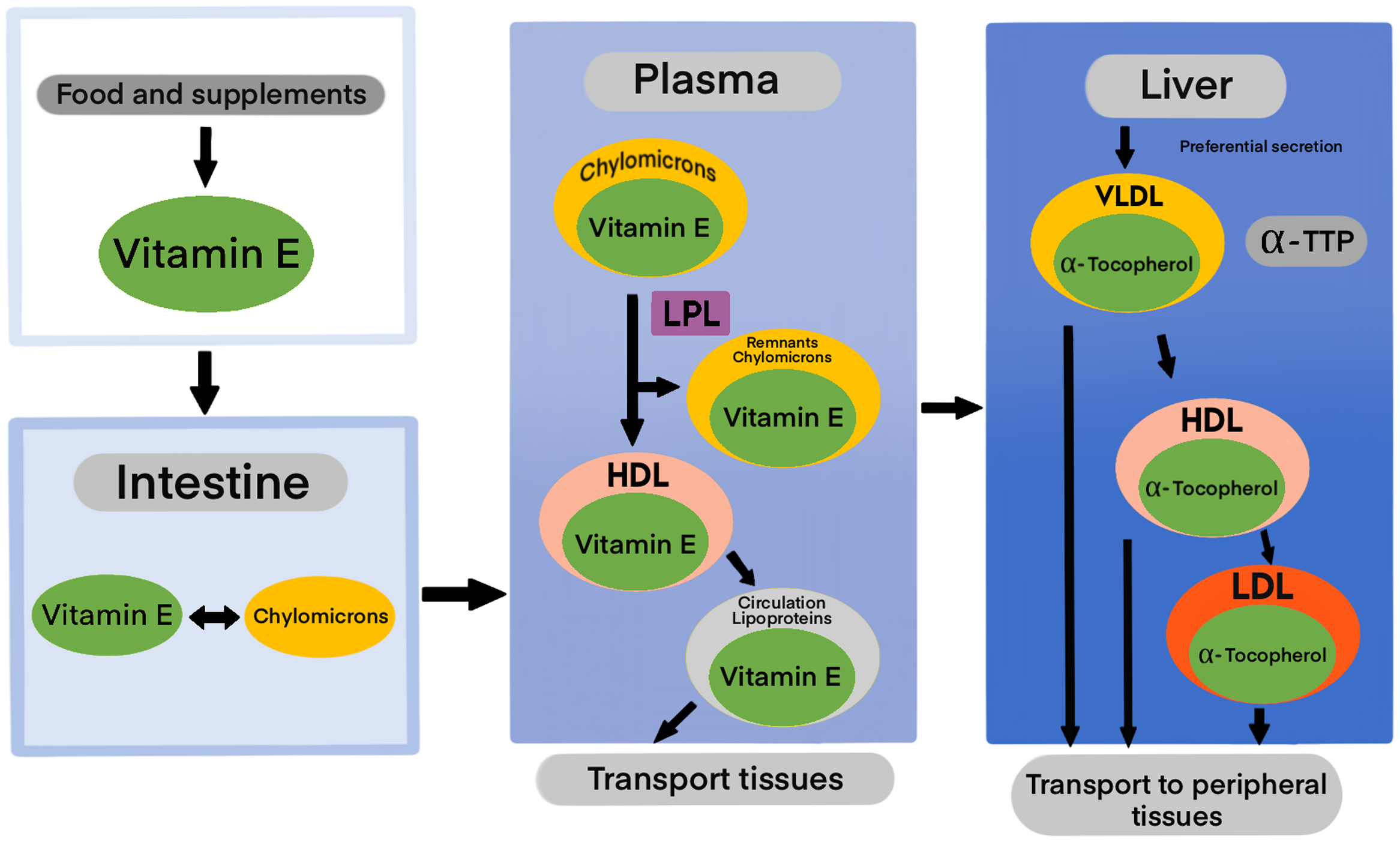
Fig. 2. Vitamin E absorption pathways (adapted from Traber, 2006)(Reference Traber, Shils, Shike, Ross, Caballero and Cousins46).
Tissues containing higher amounts of lipids have higher amounts of vitamin E. The amount of lipid in the blood is a determinant of the circulating vitamin E concentration. The liver is the organ responsible for the metabolism and excretion of vitamin E(Reference Traber17). Unlike other fat-soluble vitamins, vitamin E is highly regulated and thus does not accumulate at toxic levels in the liver(4).
The liver and intestine play important roles in the catabolism of α-tocopherol(Reference Traber, Leonard and Ebenuwa47). The main route of excretion of α-tocopherol is through faeces and in the urine(4). Since the release of vitamin E stored in tissues is a slow and little-known process, the excretion and metabolism of this nutrient becomes an important mechanism for regulating circulating concentrations in the body(Reference Dror and Allen3,Reference Traber17,Reference Traber, Leonard and Ebenuwa47) .
Traber et al.(Reference Traber, Leonard and Ebenuwa47) reported in a recent study that, after consuming a meal containing fat, part of α-tocopherol is absorbed and part is excreted in faeces. The α-tocopherol is then transported in chylomicrons in the lymph through the circulation to the liver, and preferably returns to plasma for distribution to the tissues. Excess α-tocopherol is catabolised and excreted by faeces. Urinary α-carboxyethyl hydroxychromanol (α-CEHC) excreted after consumption of fat-containing meals may be derived from sources not derived from the intestine, such as liver and kidneys. In the absence of adequate amount of fat for α-tocopherol absorption, there is an increase in the proportion of α-tocopherol catabolised in the intestine; simultaneously, there is a reduction in α-tocopherol catabolism in the liver and then excretion as urinary α-CEHC. The absence of meals and fat in adequate quantity for α-tocopherol absorption increases the proportion of α-tocopherol catabolised in the intestine but reduces the catabolised proportion in the liver and excreted as α-urinary CEHC.
Vitamin E deficiency
VED is rarely found in adults, but in children it occurs more frequently, since limited stocks of α-tocopherol are unable to maintain the rapid growth that occurs in childhood, thus allowing the symptoms associated with deficiency to be identified more easily(Reference Leonard, Traber and Harrington38).
VED has been associated with symptoms secondary to fat malabsorption and may lead to peripheral neuropathy and increased erythrocyte haemolysis(Reference Kumar, Ferrie and Balmert10,Reference Traber17,Reference Leonard, Traber and Harrington38) . Reduced concentrations of α-tocopherol may be caused by the combination of diets with low amounts of α-tocopherol and inadequate consumption of fats, proteins and calories(Reference Traber16). VED may also be caused by defects in the transport of lipoproteins or genetic defects in the hepatic α-tocopherol transfer protein(4).
Low consumption of foods containing vitamin E leads to lower absorption of this vitamin. Thus, erythrocytes become susceptible to oxidative damage and can cause anaemia. If α-TTP is deficient, α-tocopherol will be absorbed, and erythrocytes can acquire it and become protected. However, the body does not retain the vitamin in these cases, leading to its depletion in tissues. The most susceptible tissues are large-calibre sensory neurons in peripheral nerves. The signs of VED in these cases are severe owing to damage caused by free radicals(Reference Niki and Traber45).
Premature infants and very-low-birth-weight premature infants are at risk of developing VED and presenting symptoms such as haemolytic anaemia, thrombocytosis and oedema(4,Reference Baydas, Karatas and Gursu48,Reference Gomez-Pomar, Hatfield and Garlitz49) . Children who lack tendon reflexes and have low plasma levels of cholesterol and lipoproteins are suspected of presenting VED(Reference Eggermont50). On the other hand, VED may be influenced by obesity, since it may predispose individuals to this nutritional deficiency. A possible explanation for this fact is the ‘sequestration’ of tocopherol by adipose tissue(Reference Dror and Allen3) and the greater oxidative stress present in patients with obesity(Reference Traber16).
Indicators of vitamin E nutritional status
The nutritional status of vitamin E can be measured by evaluating food intake, by clinical examination and by measuring biochemical and/or physiological markers. Dietary intake may be influenced by factors such as incomplete data on food and its respective nutrients, variability in food composition or the form of preparation, inconsistency about data regarding the eating habits of individuals, and inter-individual differences in nutrient absorption and metabolism(Reference Morrissey and Sheehy8,Reference Paiva-Bandeira, Rios-Asciutti and Riviera29) .
The appearance of clinical signs of VED is rare(Reference Traber, Erdman, Macdonald and Zeisel51). Clinical methods of assessing the nutritional status of vitamin E are not very sensitive for the diagnosis of marginal deficiency status, in which there is a reduction in body stores that is severe enough to cause functional damage but does not assess visible clinical signs(Reference Morrissey and Sheehy8).
The measurement of vitamin E is technically simple, and the evaluation of plasma or serum tocopherol is a marker widely used to measure the nutritional status of vitamin E in research owing to its practicality(Reference Marquez, Yépez and Sútil-Naranjo2,Reference Dror and Allen3) . However, there are a number of confounding factors that may influence its evaluation, including age, sex, plasma lipids, use of lipid-lowering medications and smoking(Reference Dror and Allen3,Reference Morrissey and Sheehy8) . In addition, many diseases are associated with changes in the body’s vitamin E status, making it difficult to determine whether these changes constitute the cause or consequence of this process(Reference Morrissey and Sheehy8).
Biomarkers for vitamin E nutritional status
Biochemical indicators are widely used to assess the nutritional status of nutrients, and the biomarkers classically used for vitamin E evaluation are plasma/serum α-tocopherol concentration, hydrogen-peroxide-induced haemolysis, urinary excretion of α-carboxyethyl hydroxychromanol (CEHC) and α-tocopherol concentrations in adipose tissue(52).
Serum α-tocopherol is one of the main biochemical indicators for assessing vitamin E status(52). Tocopherol in plasma is carried by plasma lipoproteins. This vitamin circulates in the blood in the form of tocopherol, and the main regulator of plasmatic α-tocopherol concentration is α-TTP(Reference Traber17). The tocopherol concentration is considered a good indicator of body stores at the population level owing to its practicality of use in large studies and the possibility of laboratory analysis(Reference Dror and Allen3).
Plasma determination of α-tocopherol can be performed by HPLC methods or by ultra-performance liquid chromatography(Reference Leonard, Traber and Harrington38). A plasma concentration of α-tocopherol less than 11·6 μmol/l is considered to indicate VED(Reference Morrissey and Sheehy8,Reference Pfeiffer, Sternberg and Schleicher53,Reference Thurnham, Davies and Crump54) . Plasma concentrations lower than 8·0 μmol/l have been associated with neurological diseases in children(Reference Kalra, Grover and Ahuja55,Reference Kalra, Grover and Ahuja56) . The term VED in this study refers to low levels of α–tocopherol.
Circulating α-tocopherol is highly correlated with blood lipid levels, especially those of cholesterol. The α-tocopherol/cholesterol ratio is considered a significant indicator and the preferred measure of the status of this vitamin in healthy individuals(Reference Dror and Allen3). Some authors use the α-tocopherol/cholesterol ratio <2·2 μmol/mmol as a cut-off point for the identification of VED(Reference Morrissey and Sheehy8,Reference Schulze, Christian and Wu57) .
Tocopherol assessment can also be performed on other blood components such as erythrocytes, platelets and lymphocytes. Concentration of erythrocyte α-tocopherol has already been evaluated in studies on infant populations and patients with cystic fibrosis, premature infants, and patients with hypertension. A disadvantage of this form of evaluation is the fact that it is affected by haematocrit and plasma lipid levels(Reference Morrissey and Sheehy8).
The degree of haemolysis induced by hydrogen peroxide in vitro is a method to evaluate the state of α-tocopherol and identify the deficiency of this nutrient. This evaluation is based on the fact that erythrocytes are not able to perform de novo lipid synthesis and that the peroxidative damage resulting from oxidative stress can lead to shortening of the erythrocyte lifespan and possibly precipitate haemolysis in α-tocopherol deficiency(52). However, it is a widely criticised method and is not generally used(Reference Alfin-Slater, Hansen, Morris and Melnick58,Reference Wang, Huang and Chow59) .
α-CEHC is the final product of hepatic metabolism of different vitamin E isoforms, which is transported to the circulation for subsequent urinary excretion(Reference Leonard, Traber and Harrington38). Urinary excretion of α-CEHC may be a marker of vitamin E status(52).
The concentration of α-tocopherol in adipose tissue has been used to assess vitamin E status, although it is not an indicator with good correlation with plasma α-tocopherol concentration(Reference Traber16) and α-tocopherol intake. However, the concentration of α-tocopherol in adipose tissue seems to reflect the long-term concentration of vitamin E(52).
Clinical manifestations of VED
Neurological abnormalities
Genetic defects in α-TTP are associated with ataxia due to vitamin E deficiency (AVED)(Reference Leonard, Traber and Harrington38,Reference Di Donato, Bianchi and Federico60) , and α-TTP deficiency results in rapid plasma α-tocopherol depletion(Reference Leonard, Traber and Harrington38,Reference Morley, Cecchini and Zhang61) . Patients affected by this syndrome have neurological abnormalities, and symptoms are characterised by progressive peripheral neuropathy(Reference Traber17).
Neurological abnormalities at the onset of VED are subtle and difficult to evaluate. With the progression of deficiency, these alterations become more visible(Reference Traber16). Such neurological alterations associated with VED have already been reported in malnourished children in India and could be reversed with vitamin E supplementation(Reference Kalra, Grover and Ahuja55,Reference Kalra, Grover and Ahuja56) . On the other hand, children born to mothers with high serum vitamin E concentrations may have better cognitive function(Reference Chen, Zhang and Wei62).
Fat malabsorption syndrome
VED can be caused by any disease leading to chronic fat malabsorption, including chronic diarrhoea in children, such as hepatic cholestasis and cystic fibrosis. VED secondary to fat malabsorption occurs owing to the need for pancreatic and biliary secretions for the absorptive process(Reference Traber17).
Children diagnosed with hepatic cholestasis may experience severe malabsorption of fats caused by impaired secretion of bile into the small intestine(Reference Traber17). VED may occur in these children secondary to malabsorption, and supplementation, including PUFA supplements, is indicated to improve this nutritional aspect(Reference Sathe and Patel63,Reference Tessitore, Sorrentino, Schiano Di Cola, Colucci, Vajro and Mandato64) .
Patients with cystic fibrosis have pancreatic insufficiency, impaired secretion of pancreatic enzymes and an increased risk of malabsorption of fats and vitamin E. Such factors may favour the onset of VED in these patients(Reference Traber17,Reference Okebukola, Kansra and Barrett65) . Vitamin E supplementation associated with enzyme replacement administered orally is universally recommended for patients diagnosed with cystic fibrosis and who have pancreatic insufficiency. This treatment strategy has promising effects on the improvement of vitamin E concentrations in this group of patients(Reference Okebukola, Kansra and Barrett65). Therefore, it is advisable that vitamin E concentrations be monitored and that supplementation doses be adjusted individually in cases of deficiency(Reference Sapiejka, Krzyżanowska-Jankowskab and Wenska-Chyżyb66).
Genetic defects in lipoprotein synthesis
Lipoproteins containing apolipoprotein B are essential for the absorption and transport of plasma vitamin E. Patients with hypobetalipoproteinaemia and abetalipoproteinaemia present with steatorrhea due to impaired fat absorption, contributing to low vitamin E status. Other clinical features presented by these patients are growth retardation, acanthocytosis, retinitis pigmentosa and progressive neurological disorders with ataxia(Reference Traber17). Neurological signs resulting from abetalipoproteinaemia may start in childhood(Reference Eggermont50).
Severe malnutrition
Severely limited food intake in childhood can lead to VED due to low vitamin E intake, in addition to causing a low consumption of proteins that are necessary to synthesise α-TTP. Children with malnutrition have low plasma concentrations of α-tocopherol, and this condition can be reversed by vitamin E supplementation(Reference Traber17) and adequate consumption of vitamin E by food(Reference Traber16).
Magnitude of VED
In a systematic review of 132 studies conducted between 2000 and 2012, it was observed that the overall mean intake of vitamin E was 6·2 mg/d, and the overall prevalence of VED was 13%. In Asia, VED affects 67% of newborns and 80% of children(Reference Malik, Eggersdorfer and Kumar67). These are the groups most affected by VED, as they often have serum α-tocopherol concentrations lower than 12 μmol/l(Reference Péter, Friedel and Roos68).
Among the articles presented in this review, selected international studies that assessed nutrients in childhood found a lower prevalence of vitamin E deficiency in Asia (Saudi Arabia and Taiwan)(Reference Al-Saleh, Billedo and El-Dou69,Reference Kang, Lin and Yeh70) and a higher prevalence in North America (the United States and Mexico) (Table 2), these being the regions most affected by this nutritional deficiency(Reference Farrell, Levine and Murphy71–Reference Kim, Lora and Giraud73).
Table 2. Prevalence of vitamin E deficiency in international studies according to analysis of α-serum tocopherol

Legend: SD, standard deviation; IQR, interquartile range; VED, vitamin E deficiency; CNT, full-term children; GC, control group; GI, intervention group; HPLC, high-performance liquid chromatography; LC/MS, liquid chromatography coupled to mass spectrometry; HPLC-FR, high-performance liquid chromatography in reverse phase. Symbols: *, data not presented; †, mg/dl; ‡, µmol/l; §, health children.
The first nationwide survey in Brazil that analysed vitamin E deficiency was published in 2016 and was conducted in children aged 6–14 months from four Brazilian cities (Goiânia, Olinda, Rio Branco and Porto Alegre). A high prevalence of VED was found in the sample studied (61·5%)(Reference Cardoso, Augusto and Bortolini84). Other Brazilian studies found prevalence of VED ranging from 8·8% to 82·0%(Reference Augusto, Cobayashi and Cardoso83,Reference Morais85) .
VED can be measured by a wide range of biomarkers, and different cut-off points have been used in the literature, which makes its direct comparison difficult(Reference Dror and Allen3,Reference Monteiro, Cruz and Mussi-Pinhata82,Reference Cardoso, Augusto and Bortolini84) . Despite these factors, the results of these studies are essential to indicate the degree of disability, and the high reported prevalence indicates that it is a public health problem(Reference Dror and Allen3,Reference Fares, Sethom and Khouaja-Mokrani81,Reference Cardoso, Augusto and Bortolini84) , but prevalence data should be interpreted with caution.
Table 2 presents data from international studies on the prevalence of VED. Most studies on vitamin E are from developing countries, and studies conducted in Europe are rare. It is noteworthy that in the studies presented, the prevalence of this deficiency ranged from 1·4% to 71·3%. This wide variation is due in part to variations in the definitions of adequacy and cut-off points used, the types of studies and the difference in the age groups evaluated. Few studies address VED in children under 2 years of age.
To date, there is no widely accepted cut-off point for assessing vitamin E status, leading to divergence in the definition of vitamin deficiency, as some authors use the term ‘vitamin E insufficiency’(Reference Cardoso, Augusto and Bortolini84,Reference Oliveira, Sampaio and Muniz86,Reference Oliveira, Augusto and Muniz87) , and others ‘vitamin E deficiency’(Reference Van Biervliet, Van Biervliet and Bernard74,Reference Fares, Chahed and Feki80) for the same cut-off point (<11·6 μmol/l). In addition, some authors establish the cut-off point for vitamin E deficiency/insufficiency at values ranging from 12 μmol/l(Reference Schulze, Christian and Wu57) to 15 μmol/l(Reference Sirivichayakul, Changbumrung and Chanthavanich75).
Population-based studies evaluating the impact of vitamin E fortification and changes in serum α-tocopherol levels in childhood are rare. Table 3 presents the clinical trials that evaluated the impact of complementary feeding fortification with multiple micronutrients in childhood, including the evaluation of serum α-tocopherol concentrations. Among the five studies presented in Table 3, four studies were conducted by the International Research on Infant Supplementation (IRIS) study group that evaluated supplementation with multiple micronutrients provided in chewable tablets and serum tocopherol concentrations in children aged 6–12 months from Indonesia, Peru, South Africa and Vietnam. The authors observed that there was a significant increase in vitamin E concentrations in the group that received supplementation compared with baseline values or compared with the control group, except for Peruvian children(Reference Untoro, Karyadi and Wibowo88–Reference Hop and Berger92).
Table 3. Clinical trials on the impact of complementary feeding fortification with multiple micronutrients on serum tocopherol levels

Legend: DMM, multiple micronutrients daily; WMM, weekly multiple micronutrient (supplement on 1 d and placebo for 6 d); DI, iron supplementation daily; P, placebo; GI, intervention group; CG, control group. 1Composition: vitamins A, D, E, K, C, B1, B2, B6 and B12, niacin, folate, iron, zinc, copper and iodine.
Lobo et al.(Reference Lobo, Schincaglia and Peixoto94) evaluated the effectiveness of homemade fortification with powdered micronutrients in vitamin E levels in children aged 6–15 months and identified an 82% reduction in VED in children who received home fortification for a period of 2–3 months. In addition, the supply of homemade fortification promoted higher length/age indexes, a lower frequency of morbidity indicators (cough and wheezing) and a reduction in the presence of subclinical inflammation.
Inflammatory state and vitamin E
The plasma concentration of several nutritional biomarkers is influenced by inflammation(Reference Tomkins95), and the presence of subclinical inflammation in apparently healthy individuals may alter biomarkers of nutritional status. Therefore, it is important that inflammatory biomarkers are evaluated together with nutrients of interest(Reference Thurnham and Northrop-Clewes96).
The acute-phase response and the consequent change in acute-phase protein concentrations can lead to metabolic changes with significant nutritional implications, such as reduced nutrient intake(Reference Bhutta97,Reference Raiten, Ashour and Ross98) due to reduced appetite and fever; impaired nutrient metabolism, including reduced nutrient digestion(Reference Raiten, Ashour and Ross98,Reference Scrimshaw99) , endogenous nutrient losses and changes in nutrient transport and regulation; and increased energy needs due to metabolic changes and the host immune response(Reference Raiten, Ashour and Ross98).
In addition, other factors may contribute to nutritional deficiencies, such as low body stocks of nutrients as a result of maternal malnutrition during pregnancy, dietary deficiencies, high consumption of nutrient absorption inhibitors, and increased body losses(Reference Bhutta97). The risk of developing nutritional deficiencies may be aggravated by a short breastfeeding duration and inadequate practices during the period of introduction of complementary feeding(100).
Adequate nutritional status is an important factor for maintaining normal immune system functions and preventing or attenuating dysfunction induced by internal or external aggressor factors. Thus, nutritional deficiencies usually result in the impairment of these functions. On the other hand, the consumption of nutrients in adequate amounts can restore or further improve the immune system(Reference Lewis, Meydani and Wu101).
Vitamin E is an important nutrient for the modulation of the immune system. This is due in part to their protective effect against the oxidation of polyunsaturated fatty acids that are enriched in cell membranes belonging to the immune system, making them prone to oxidative damage(Reference Lewis, Meydani and Wu101,Reference Lee and Han102) . VED can impair the immune system(Reference Wu, Lewis and Pae103) and inflammatory profile through regulatory signals such as alterations in membrane integrity and signal transduction, modulation of inflammatory mediators, and the cell cycle. Modulation of the immune system with the use of vitamin E has clinical relevance and affects the susceptibility of the host to infections by viruses and bacteria, among others(Reference Lewis, Meydani and Wu101).
The concentrations of vitamins in plasma can be reduced in the presence of inflammation; however, the effect of the systemic inflammatory response on plasma vitamin E concentration appears to be relatively small(Reference Duncan, Talwar and Mcmillan104–Reference Ulatowski, Dreussi and Noy106). The reduction in appetite and food consumption in sick individuals with the presence of an inflammatory condition can lead to changes in the plasma concentration of micronutrients. In these cases, the altered nutrient concentration may be a consequence of reduced body nutrient status and is indirectly linked to an increased inflammatory response(Reference Duncan, Talwar and Mcmillan104). On the other hand, severe conditions also decrease serum lipids as a result of increased free-radical activity, which is why vitamin E concentration goes down(Reference Galloway, Mcmillan and Sattar105). Some authors demonstrated reduced tocopherol levels in patients with asthma(Reference Kalayci, Besler, Kilinc, Sekerel and Saraclar107–Reference Shvedova, Kisin, Kagan and Karol113) and adult and neonatal mice with allergic inflammation(Reference Traber16) Thus, it is necessary to control the inflammatory state and serum lipids levels when it is necessary to evaluate the serum concentrations of fat-soluble vitamins(Reference Silva114).
Therefore, it is suggested that an important aspect of the development of VED in children is the presence of infectious processes. In addition, VED may also be associated with the development of infectious diseases in childhood(Reference Leonard, Traber and Harrington38) by decreasing immunological resistance, generating a vicious cycle between this nutritional deficiency and infectious processes. Individual factors such as age, socio-economic status, feeding and breastfeeding may influence the acute-phase response and, therefore, the pattern of inflammation(Reference Raiten, Ashour and Ross98).
Strategies for the prevention and control of VED
The adequacy of vitamin E during the first 1000 d of life is important for health and wellbeing in adulthood(Reference Traber16,Reference Leonard, Traber and Harrington38) . Different types of interventions can become essential with the aim of improving vitamin E status in childhood. These approaches will be demonstrated below.
Dietary modifications and food and nutrition education
Food diversification is a relevant strategy for the control and prevention of hypovitaminoses(Reference Gibson, Hotz and Temple115) and refers not only to access to food but also to other important factors such as the economic conditions for acquiring it and the bioavailability and utilisation of the ingested nutrient(Reference Vellozo and Fisberg116). This process takes place through food and nutritional education actions and aims to promote healthier eating habits(117).
Regarding food, it is known that breastfeeding is the essential measure for the prevention of nutritional deficiencies up to 6 months of life. From 6 months, in addition to breast milk, the diet has the function of completing the amounts of nutrients necessary for the growth and development of the child, to avoid the deficiency of these micronutrients(118).
A variety of foods in the appropriate amounts, as part of a non-monotonous diet, is paramount for maintaining the health and growth of the child and avoiding specific nutrient deficiencies(119,120) .
Optimal vitamin E status can be achieved by modifying the diet and introducing foods rich in vitamin E. For these changes in food production, selection and processing practices become necessary. Thus, it is possible to ensure better availability of, access to and utilisation of foods with a high content and bioavailability of micronutrients(117). In addition, it is important to encourage the production and local cultivation of food sources of vitamin E and actions of Food and Nutrition Education addressing topics related to the importance of food diversification(Reference Dror and Allen3). It is known that increased intake of vitamin E food sources improves its status(Reference Batista, Costa and Pinheiro-Sant’ana121), and it is possible that interventions such as these may be directed to high-risk populations in developing countries(Reference Dror and Allen3).
Food fortification
Food fortification is an efficient public health strategy and recommended mainly for vulnerable populations living in regions where there is a high prevalence of micronutrient deficiency(Reference Osendarp, Martinez and Garrett122), aiming at improving health conditions related to malnutrition. Food fortification can be cost-effective as long as enriched foods are consumed regularly, thus maintaining constant body reserves(Reference Serdula123). This technique presents high population coverage, does not modify eating habits and presents a low risk of toxicity. The amount consumed of the fortified food and the concentration of the micronutrient contained in it should be taken into account in a food fortification programme(117).
The effectiveness of this strategy depends on a commitment between the government through supply policies and the food industries involved(Reference Bailey, West and Black124,Reference Tam, Keats and Rind125) , in addition to the need to clarify the population in order to orient itself on the preference for consumption of food fortified with micronutrients. The association and cooperation between the health sectors involving food and nutrition policies, the food industry and the scientific community is extremely important for this strategy to be an opportunity to combat nutritional deficiencies and, thus, can provide better health conditions for the population(117).
In addition to occurring naturally in food, vitamin E can be added with the aim of increasing the concentration of this vitamin, that is, fortification(Reference Batista, Costa and Pinheiro-Sant’ana121). Experimental studies have shown that fortification of foods such as milk(Reference Hayes, Pronczuk and Perlman126), margarine(Reference Van Het Hof, Tijburg and De Boer127) and breakfast cereals(Reference Leonard, Traber and Harrington38) was effective in improving α-tocopherol levels. These data reinforce the importance of food fortification, but it is important to emphasise that fortified foods and their costs should be accessible to the target population and based on local needs to provide the desired result(Reference Liberato and Pinheiro-Sant’ana128).
The fortification of foods with vitamin E is not yet carried out on a large scale, and one of the reasons for this fact is that there is still no consensus on the dose to be consumed to achieve health benefits(Reference Batista, Costa and Pinheiro-Sant’ana121). However, some studies suggest the need to intake foods fortified with vitamin E in order for the nutritional recommendation to be achieved(Reference Van Het Hof, Tijburg and De Boer127,Reference Serra-Majem129,Reference Sichert-Hellert, Kersting and Alexy130) .
Fortification of food with powdered micronutrients
The World Health Organization (WHO) recommends strengthening infant feeding with multiple micronutrient powder to reduce nutritional deficiencies in childhood(131). Food fortification with micronutrients has several advantages: it can provide the recommended daily nutrient intake(Reference Tam, Keats and Rind125); fortified food contains essential micronutrients to prevent and treat micronutrient deficiencies and improve the nutritional status of children(Reference Cardoso, Augusto and Bortolini84,Reference Hop and Berger92,131–Reference Silva, Augusto and Tietzmann134) ; it is easy to administer, micronutrients can be added to prepared and ready-to-eat foods, and it is based on food and can be easily incorporated into food practices(135). The number of countries that have adhered to this strategy in recent years has increased, and the use of multiple micronutrient powder had been implemented in forty-three countries by 2014(136).
Conclusion
Serum α-tocopherol is the most used biochemical indicator to assess the prevalence of VED, but there is insufficient evidence as to whether its levels may be reduced in the presence of inflammation. High proportions of VED provide evidence that this nutritional deficiency is a public health problem in children and still little addressed in the international scientific literature.
Nutritional interventions should be implemented with a view to recognising by population the importance of the food consumption source of vitamin E. Fortification, food diversification and fortification with multiple-micronutrient powder is highly relevant for increasing vitamin E intake.
The planning, evaluation and implementation of health policies aimed at combatting VED in the child population are extremely important. Studies evaluating VED in the infant population should be conducted, as they serve as an important source of information and surveillance for health managers.
Author contributions
Conceptualisation, investigation, data curation, writing – original draft preparation, writing – review and editing, visualisation: M.C.C.M.H. and L.M.C.L. All authors read and approved the final version.