1 Introduction
All-solid-state femtosecond lasers that deliver high peak power and extremely narrow pulse width are of great interest in many fields such as scientific research, industry, medical treatment, and high speed communication. In particular, ytterbium-based solid-state lasers have attracted increasing attention because of their excellent optical and thermal properties. Recently, a great number of Yb-doped materials including Yb:CaF2
[Reference Pirzio, Di Dio Cafiso, Kemnitzer, Kienle, Guandalini, Aus der Au and Agnesi1, Reference Machinet, Sevillano, Guichard, Dubrasquet, Camy, Doualan, Moncorgé, Georges, Druon, Descamps and Cormier2], Yb:CALGO[Reference Sévillano, Georges, Druon, Descamps and Cormier3–Reference Manjooran and Major5], Yb:CALYO[Reference Tian, Peng, Zhang, Yu, Zhu, Xu and Wei6], Yb:KYW[Reference Bain, Lagatsky, Brown and Sibbett7], Yb:KGW[Reference Zhao and Major8–Reference Akbari and Major10] and Yb:Lu2O3
[Reference Tokurakawa, Shirakawa, Ueda, Peters, Fredrich-Thornton, Petermann and Huber11] crystals as well as Yb:LuAG ceramic[Reference Kitajima, Nakao, Shirakawa, Yagi and Yanagitani12] have shown their capacity in generating sub-100-fs pulses with watt level average power via either pure Kerr-lens mode-locking (KLM) or combination of KLM and saturable absorber-based passive mode-locking. Among them, Yb-doped calcium aluminate crystals including Yb:CALGO and Yb:CALYO are the most attractive candidates for high-power sub-50-fs pulses generation, due to their flat emission spectra with more than 70 nm bandwidths as well as high thermal conductivity. Regarding sub-50-fs pulses generation, Yb:CALGO and Yb:CALYO crystals have already been proven their abilities by Zaouter et al.
[Reference Zaouter, Didierjean, Balembois, Lucas Leclin, Druon, Georges, Petit, Goldner and Viana13], Agnesi et al.
[Reference Agnesi, Greborio, Pirzio, Reali, Aus der Au and Guandalini14], Gao et al.
[Reference Gao, Zhu, Wang, Wei, Xu, Zheng, Su and Xu15] and Ma et al.
[Reference Ma, Huang, Ning, Xu, Xie, Qian, Loh and Tang16], respectively. Up to more than 3 W average power with 45-fs pulses was obtained by Manjooran et al.
[Reference Manjooran and Major5]. Still, most of the obtained average powers from above literatures were only on the order of several tens of milli-watt, due to either the small pump power of the single-mode fiber coupled laser diodes (LDs) or the critical cavity setup restrained by the low-brightness multi-mode LDs. One way to cope with the issue raised by the low-brightness LDs is to use a double confocal cavity which separates the gain medium and Kerr medium. Paradis et al. demonstrated the generations of 35-fs pulses with 1.5-W average power and 49-fs pulses with 4.5-W average power from the KLM Yb:Lu2O3 thin-disk laser, respectively, with 76-W and 96-W pump power,[Reference Paradis, Modsching, Wittwer, Deppe, Kränkel and Südmeyer17]. However, the corresponding optical-to-optical efficiency is only 2.1% and 4.7%, respectively. In terms of the KLM Yb:CALGO thin-disk laser, although down to 30-fs pulses were delivered, the average power was only 150 mW, due to the low gain and limited disk quality[Reference Modsching, Paradis, Labaye, Gaponenko, Graumann, Diebold, Emaury, Wittwer and Südmeyer18]. And for Yb:CALYO thin-disk lasers, no relevant mode-locking result has been reported so far. On the other hand, compared to the low-power single-mode fiber coupled LDs, single-mode fiber laser emitting at 976 nm emerging recently has not only higher average power up to 10 W[Reference Wu, Zhu, Wei, Wiersma, Li, Zong, Chavez-Pirson, Temyanko, LaComb, Norwood and Peyghambarian19], but also excellent beam quality with
$M^{2}<1.1$
, distinct linear polarization characteristic as well as much lower noise. As a result, it is of great interest to generate sub-50-fs pulses with high average power by pumping the Yb:CALGO or Yb:CALYO crystal with a single-mode fiber laser. In 2014, Sévillano et al. demonstrated a single-mode fiber-laser-pumped pure KLM Yb:CALGO oscillator, delivering 40-fs pulses with 1.1-W average power[Reference Sévillano, Georges, Druon, Descamps and Cormier3]. However, preliminary results of only 250-mW output power and 57-fs pulse duration were generated from a single-mode fiber-laser-pumped Yb:CALYO laser[Reference Yu, Han, Xie, Peng, Xu and Wei20], which is far away from the expectation of generating much higher output power with shorter pulse duration considering the good optical and thermal properties of the Yb:CALYO crystal.
In this letter, we demonstrated the single-mode fiber-laser-pumped high-power Yb:CALYO lasers passively mode-locked by semiconductor saturable absorption mirror (SESAM) and pure Kerr-lens mode-locking, respectively. 103-fs pulses with up to 3.1-W average power are obtained from passive mode-locking, corresponding to the optical-to-optical efficiency of 31%. In addition, as short as 36-fs pulses with up to 2-W average power are obtained from the pure KLM with extra-cavity dispersion compensation. With the repetition rate of 84 MHz, the pulse energies and peak powers for SESAM-based passive mode-locking and KLM are 37 nJ, 0.31 MW and 24 nJ, 0.58 MW, respectively.
2 Experimental setup
A schematic diagram of the experimental setup is shown in Figure 1. The pump source is a commercial single-mode fiber laser emitting at 976 nm with up to 10-W output power (ALS-IR-75, Azur Light Systems). The pump beam was focused by a lens of 60-mm focal length into a 6-mm long Yb:CALYO crystal with a waist of
$26.5~\unicode[STIX]{x03BC}\text{m}$
(
$1/e^{2}$
) and a Rayleigh length of 2.26 mm. The 5 at.% Yb3+-doped gain medium with anti-reflection coating around 980–1100 nm on both surfaces was wrapped with an indium foil and tightly mounted in a water-cooled copper heat sink to maintain at
$14\,^{\circ }\text{C}$
during the experiment. A half-wave plate was used to adjust the polarization of the pump beam since the absorption of Yb:CALYO crystal for
$\unicode[STIX]{x1D70B}$
polarization is much higher than that for
$\unicode[STIX]{x1D70E}$
polarization. C1 and C2 were two dichroic concave mirrors with 75-mm radius of curvature (ROC) and coated with high reflectivity over 1020–1100 nm (
$R>99.9\%$
) and high transmittance around 800–1000 nm (
$R<5\%$
). Several Gires–Tournois interferometer (GTI) mirrors were employed in the cavity for intra-cavity dispersion compensation. C3 was a concave mirror with ROC of 300 mm, coated with high reflectivity in the region of 1020–1100 nm (
$R>99.9\%$
). Wedged output couplers (OCs) were used to couple out the laser while avoiding mode-locking instability from the back reflection. The total cavity length was about 1.8 m, corresponding to 84 MHz repetition rate.

Figure 1. The sketch for the experimental setup of the single-mode fiber-laser-pumped mode-locked Yb:CALYO laser. HWP: half-wave plate; L: lens; C1–C3: concave mirrors; GTI1–GTI4: Gires–Tournois interferometer mirrors; OC: output coupler; SESAM: semiconductor saturable absorption mirror.
3 Results and discussion
At first, the cavity was optimized in a continuous-wave (CW) operation, which delivers 4.2 or 3.6-W output powers with 15% or 10% OCs. The absorption efficiency of the crystal for pump laser is up to 99.5% under lasing. Then, to investigate the performance of a passive mode-locking operation, a commercial SESAM (SAM-1040-0.7-1ps-x from BATOP GmbH) with 0.4% modulation depth at 1040 nm was utilized. With 10% transmittance OC, a stable mode-locking operation with up to 3.1-W average power was achieved when the total group delay dispersion (GDD) per round trip introduced by the three GTIs was
$-6600~\text{fs}^{2}$
(the GDD of GTI1, GTI2 and GTI3 per bounce is
$-1000~\text{fs}^{2}$
,
$-1000~\text{fs}^{2}$
and
$-1300~\text{fs}^{2}$
, respectively). The corresponding optical-to-optical efficiency is more than 30%. Operating in open air, the root mean square of power fluctuation over half an hour was around 1.8%. We characterized the optical spectrum as well as the temporal property via a commercial optical spectrum analyzer (AvaSpec-ULS2048, Avantes) and an intensity auto-correlator (APE PulseCheck USB), respectively. As shown in Figure 2(a), the optical spectrum of the pulses was centered at 1060.6 nm, with the full-width at half-maximum (FWHM) bandwidth of 11.7 nm. The corresponding intensity auto-correlation trace has an FWHM duration of 159 fs, as shown in Figure 2(b). If assuming a sech2 pulse shape, it results in a pulse duration of 103 fs. The corresponding time-bandwidth product was 0.327, which is very close to the transform-limited value of 0.315. Provided the 84 MHz repetition rate, the corresponding single pulse energy and peak power were 37 nJ and 0.31 MW, respectively.
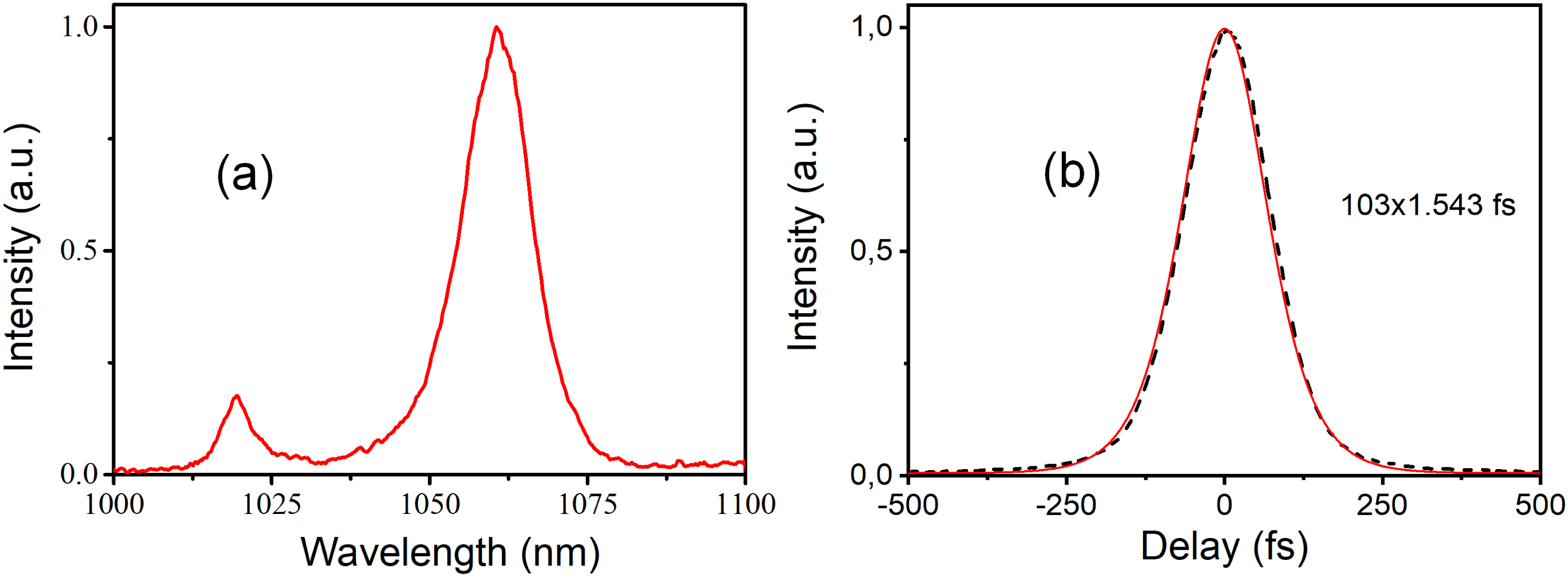
Figure 2. (a) Optical spectrum and (b) the corresponding auto-correlation trace of the passively mode-locked Yb:CALYO laser. The black dot and red solid curve in (b) stand for the experimental data and sech2-fitting, respectively.

Figure 3. (a) Spectra; (b) output powers and pulse durations of different pure KLM operations realized by detuning the cavity with
$\unicode[STIX]{x0394}L$
; output properties under different pump power for KLM operation at
$\unicode[STIX]{x0394}L=-6.126~\text{mm}$
: (c) evolution of spectra and (d) changing in average power as well as pulse duration.
For pure Kerr-lens mode-locking operation, the SESAM was replaced by a fourth GTI mirror. When the dispersion provided by GTI4 per bounce was
$-1000~\text{fs}^{2}$
, several different KLM operations were able to be realized by tuning the position of GTI4 close to the stability edge of the cavity. Because of the excellent beam quality of the pump laser and proper cavity design, soft-aperture type of KLM is easy to realize. When just slightly detuning the cavity with a distance of
$\unicode[STIX]{x0394}L=-0.660~\text{mm}$
(the position of
$\unicode[STIX]{x0394}L=0~\text{mm}$
is where the maximum CW output power is produced), initial KLM operation would be obtained by a weak perturbation such as taping the mirrors or pushing the translation stage. The corresponding average power and pulse duration were 3.64 W and 116 fs, respectively. Then by further reducing the distance between C3 and GTI4, shorter pulses with broader spectrum were produced at the cost of linear decrease of the output power. The closer the cavity is to the edge of a stable region, the deeper the modulation depth of KLM is, resulting in higher loss. As shown in Figures 3(a) and 3(b), we recorded and compared the performances of different KLM operations versus the position of GTI4 mirror. The broadest spectrum was obtained at
$\unicode[STIX]{x0394}L=-6.126~\text{mm}$
, which covers from 980 to 1090 nm. The lower intensity at shorter wavelength was due to the relatively high re-absorption of Yb:CALYO crystal. Via Fourier transform with zero chirps, the transform-limited pulse duration is 34 fs, meaning that there is residual chirp in the directly measured 66-fs pulses. At the position of
$\unicode[STIX]{x0394}L=-8.845~\text{mm}$
, the spectrum did not get broadened while the pulse duration was shortened to 61 fs, where the average power remained 1.52 W. Due to the decreasing of average power, it was not able to support the stable KLM operation when the absolute value of
$\unicode[STIX]{x0394}L$
was over 8.9 mm.
For a given position of GTI4 (
$\unicode[STIX]{x0394}L=-6.126~\text{mm}$
), we characterized the performances of KLM pulses at different pump powers, as shown in Figures 3(c) and 3(d). It is clear that the pulse duration and average power of the laser pulses were decreasing, and linearly increasing with the rising of pump power, while their spectra did not change a lot. The corresponding slope efficiency of such a KLM operation was 30.4%. At the maximum pump power of 10 W, neither obvious thermal distortion nor multi-pulse operation was observed, indicating that the maximum output power of 2.1 W was limited by the limited pump power, and higher output power is possible with higher pump power.

Figure 4. (a) The auto-correlation trace of the compressed pulses. Black and red curves are experimental result and sech2 fitting, respectively. The blue curve is the auto-correlation trace of the Fourier transformation of the corresponding optical spectrum. (b) The corresponding RF spectrum of the 36-fs pulses measured with frequency windows of 3 MHz at 1 kHz of RBW and 0.6 GHz at 100 kHz of RBW (inset), respectively.
To reduce the residual chirp of the femtosecond pulses, extra-cavity compression was implemented by using a pair of GTI mirrors with
$-950~\text{fs}^{2}$
GDD in total. As a result, down to 36-fs pulses were obtained assuming a sech
$^{2}$
shape; the corresponding auto-correlation trace is shown in Figure 4(a). Meanwhile, the average power remained 2 W, which is believed to be the highest average power from the reported sub-40-fs Yb-based solid-state oscillators. The corresponding single pulse energy and peak power were 24 nJ and 0.58 MW, respectively.
To claim the status of the KLM operation, the radio frequency (RF) spectra were measured via a photodetector (PD) with bandwidth of 1 GHz and a commercial RF spectrum analyzer (Agilent E4407B). As described in Figure 4(b) is the RF spectrum of the compressed pulses of KLM operation at
$\unicode[STIX]{x0394}L=-6.126~\text{mm}$
. The signal was recorded for frequency spans of both 3 MHz with 1 kHz resolution bandwidth (RBW) and 0.6 GHz with 100 kHz RBW. The distinct signal-to-noise ratio of the fundamental beat note at 84.3 MHz was as high as 70 dB. No obvious side peaks of the several harmonics of the fundamental frequency were observed, which indicates that the KLM operation was running stably. Due to the excellent beam quality of the single-mode fiber laser as well as the well-designed cavity, the pure KLM operation could last for several tens of hours in open air once started.
4 Conclusion
In conclusion, regarding the excellent optical property of the Yb:CALYO crystal, we have studied the KLM Yb:CALYO laser pumped by a single-mode fiber laser. Using an output coupler of 15% transmittance, different states have been realized by detuning the cavity. As the cavity was getting closer to the edge of stable region, the Kerr strength increased so that the modulation depth was rising accordingly. As a result, broader spectra as well as shorter pulses were obtained. The broadest spectrum was able to cover the wavelength from 980 to 1090 nm, which supports 34-fs pulses with zero chirp. By extra-cavity chirp compensation, near transform-limited pulses of 36 fs have been demonstrated. The average power after compression was 2 W, meaning that the single pulse energy and peak power were up to 24 nJ and 0.67 MW, respectively. An overview of the state-of-the-art performance of ultrafast Yb solid-state oscillators generating sub-40-fs pulses is given in Table 1.
Table 1. Overview of the state-of-the-art performance of ultrafast Yb solid-state oscillators generating sub-40-fs pulses. a

a
$\unicode[STIX]{x1D70F}$
, pulse duration;
$P_{\text{out}}$
, average output power; TDL, thin-disk laser.
Compared to the previous works in sub-40-fs generation from Yb solid-state lasers, we have presented, to the best of our knowledge, the highest average power as well as the largest single pulse energy within bulk geometry. Since no saturation in average power and multi-pulse operation was observed, sub-50-fs pulses with higher average power are believed to produce from such a single-mode fiber-laser-pumped Yb:CALYO laser with higher pump power or longer crystal. It is noticed that the shorter wavelength (980 nm–1040 nm) of the spectrum has relatively lower intensity due to the re-absorption. Another possibility is using a gain-matched output coupler[Reference Cihan, Beyatli, Canbaz, Chen, Sumpf, Erbert, Leitenstorfer, Kartner, Sennaroglu and Demirbas23] to realize smoother spectrum, which is possible to support pulses with only a few cycles of pulse duration. Above all, the broad spectrum as well as good power stability of the laser provides a unique ultrafast source for seeding the femtosecond solid-state amplifier.
Acknowledgements
This research has been supported by the National Key Basic Research Program of China (No. 2017YFB0405202), National Natural Science Foundation of China (NSFC) (Nos. 61705174 and 11774277), Natural Science Basic Research Plan in Shaanxi Province of China (No. 2018JQ6061) and Open Research Fund of the State Key Laboratory of Pulsed Power Laser Technology, Electronic Engineering Institute, Hefei, China (No. SKL2017KF04).