The Odyssey of the African Buffalo
The African continent hosts a unique diversified megafaunal assemblage, one which exceeds that of any other biogeographical region of the world. The African buffalo is the largest African bovid and occurs throughout most of sub-Sahara in a wide range of ecosystems, from savanna to rainforest. It exhibits a marked morphological polymorphism across its range, greater than most other African mammals, both in body size and weight, but also in pelage colour and horn shape. The African buffalo is also one of the most successful large African mammals in terms of abundance and biomass. In this context, this species represents a powerful model to enhance our understanding of African biogeography and wildlife conservation.
Buffalo, together with around 80 per cent of ungulates (hoofed mammals), belong to the bovid family, characterized by the presence of two or rarely four unforked horns (at least in the adult male). In Africa, this family, which includes the true antelopes and the African buffalo, emerged some 2.8 million years ago following the increase of open habitats and the expansion of grasslands.
Since the differentiation of African buffalo, which took place some 500,000 years ago, major climate fluctuations during the Quaternary shaped distribution range and caused population oscillations. The expansion of the equatorial rainforest towards eastern Africa during pluvial periods periodically formed a major biogeographical barrier to gene flow between savanna populations. This is why African wildlife species with a continental distribution pattern show a biogeographical pattern structured north and south of the equatorial forest belt. In the African buffalo, this resulted in the emergence of two main genetic lineages: Syncerus caffer caffer in eastern and southern Africa, and S. c. nanus in western and central Africa.
In addition to climate change, humans and their newly domesticated animals have posed a worldwide ecological threat to wildlife since the beginning of the Holocene epoch (about 12,000 years ago). The Holocene resulted in the extinction of many mammals weighing more than 40 kg. Nevertheless, such a drastic reduction did not occur in Africa, where humans and wildlife had long coevolved (with the exception of some species like the giant long-horned buffalo (Pelorovis antiquus); Chapter 2).
In Africa, anthropogenic pressure on African wildlife took on an unprecedented scale from the Neolithic revolution onwards, marked in eastern and southern Africa by the Bantu expansion. From about 3000 bc until ad 1800, human populations originating from present-day Cameroon, Central African Republic and Congo undertook a long series of migrations and settled eastern and southern Africa, which until then had been occupied by small populations of nomadic huntergatherers for the past 100,000 years. These migrants brought with them several important commodities and skills, such as domesticated animals (including cattle), cultivation of crops (millet, sorghum, etc.) and the manufacture of metal weapons. This revolution, together with climatic factors, resulted in a strong decline of Cape buffalo populations as shown by recent genetic investigations (Chapter 3).
About 130 years ago, another major crisis hit the African buffalo populations on a continental scale. The introduction of the exotic rinderpest morbillivirus in 1889 by a colonial military expedition to the Horn of Africa caused up to 95 per cent mortality among buffalo populations, wildebeest and cattle across Africa. This was followed by other episodes throughout the twentieth century, until its official eradication in 2011 (last case reported in 2001; Chapter 4). Despite an extremely high mortality rate reported at the continental scale, the Great Rinderpest pandemic apparently had very low impact on the genetic diversity of the African buffalo, as shown by recent studies (Chapter 3). Throughout the twentieth century, buffalo populations gradually recovered, but obviously not to the levels that prevailed before the Great Rinderpest pandemic of the 1880s.
The sub-Saharan human population grew exponentially throughout the twentieth century, increasing from 95 million in 1900 to 1.1 billion by 2020. According to current projections, this figure could reach 1.8 billion in 2050 (i.e. a nearly 20-fold increase in 150 years). To meet the needs of the human populations in Africa and the raw material needs of the rest of the world, many natural habitats have been transformed or severely degraded, and what remains is under increasing pressure. The original distribution range of the African buffalo and wildlife in general therefore was (and still is being) progressively reduced and relegated to protected areas, which today cover around 16 per cent of the total land area. However, in the face of human pressure, the integrity of numerous protected areas has been jeopardised. Hence, the overall number of large mammals in Africa within protected areas decreased by 60 per cent between 1970 and 2005 and by about 85 per cent in Western Africa during that same period.
In response to this major crisis, African governments together with the international community have mobilized in recent decades, and conservation efforts are beginning to bear fruit. As we will see in Chapter 4, African buffalo populations have stabilized overall over the last 20 years. However, these figures conceal major disparities between regions, as well as the concentration of wildlife populations in the besieged fortresses that protected areas have become.
Introduction
If one sees an African buffalo (Syncerus caffer) for the first time after seeing many water buffalo (Bubalus bubalis), one could easily believe they are closely related. In 1758, Carolus Linnaeus named the water buffalo scientifically, but he did not classify the African buffalo. The first formal mention is by Anders Sparrmann (Reference Sparrmann1779), a pupil of Linnaeus, who classified the species as Bos caffer, just as his mentor had classified the Asian species as Bos bubalis. A military artist named Charles Hamilton Smith coined the Latin genus name Bubalus for the Asian buffalo in 1827. A nineteenth-century taxonomist, Brian Hodgson, elevated the African buffalo to its own genus, namely, Syncerus Hodgson, Reference Hodgson1847. What justifies the separation of these two ‘buffalo’ into distinct genera? Strangely enough, two fundamental characters: namely, in Syncerus the vomer and the palate are not fused, and the nuchal hair-stream is not reversed (Groves, Reference Groves1969). Groves states: ‘Consequently the generic separation of Bubalus from Syncerus seems thoroughly justified, and some at least of the similarity between them (such as that in the shape of the horn cores) must be put down to parallelism.’ Whether these two fundamental traits have any ecological meaning is unknown, but the case for parallelism is intriguing.
A systematic classification is in principle based on diagnosable (often morphological, thus not necessarily functionally important) characters, mainly of extant species (see Zachos, Reference Zachos, Zachos and Asher2018 for a review). Systematicists decided that the African buffalo should not be classified into one genus with the Asian buffalo, but does the fossil material combined with DNA-based phylogenies provide enough clues to establish the evolutionary history of the African buffalo? Our analysis will show that there is quite some doubt as to whether the African buffalo is related to the Asian buffalo species swarm, or to the larger one comprising wild cattle, yak and bison. The question that arises, of course, is whether taxonomy and systematics have any bearing on ecology and management. We believe it does if, by having knowledge on related species, one can more safely generalize; if not, then systematics at the level of the genus or higher is irrelevant. Indeed, conservation is about species – not genera, families or tribes.
The African buffalo is a large bovid. Mammals are classified as ‘bovid’ if they have, at least in the adult male, two or rarely four unforked horns. These are composed of bone cores protruding from their skull after ‘horn buds’ which are covered by a permanent layer of keratin start growing in the skin and fuse with the skull (Davis et al., Reference Davis, Brakora, Stilson, Melletti and Burton2014). Bovids emerged some 18.5 Myr ago (Vrba and Schaller, Reference Vrba, Schaller, Vrba and Schaller2000) or even slightly more recently (Bibi, Reference Bibi2013). Some studies refer to an older emergence of the Bovidae based on material from Mongolia in the Middle Oligocene, thus 26 Myr ago (Trofimov, Reference Trofimov1958; see Thomas, Reference Thomas1984), but this is now questioned (Métais et al., Reference Métais, Antoine and Marivaux2003). The mammals classified as Bovidae are thought to be related to each other, and the common trait of unforked horns is taken to be a shared, derived character, common between ancestor and descendants. Modern molecular techniques allowed this assumption to be put to the test, resulting in updated insights about the classification of the ~140 bovid species within the approximately 40 genera (Grubb, Reference Grubb, Wilson and Reeder1993). Within this group of Bovidae, African buffalo are classified with the subfamily Bovinae, within the tribe Bovini. The other two tribes in that subfamily are the Tragelaphini and the Boselaphini. All other bovids are classified within the subfamily Antilopinae.
Modern molecular techniques show that the subfamily Antilopinae as classified by morphologists has a very different evolutionary, and thus classificatory, structure than previously thought (Ropiquet and Hassanin, Reference Ropiquet and Hassanin2005; Hassanin, Reference Hassanin, Melletti and Burton2014). Enough reasonably well-dated fossils are available to pinpoint some major bifurcations between tribes in time. These phylogenies all suggest that the tribe Bovini is nested together with the Tragelaphini and the Boselaphini in one ‘proper’ subfamily, the Bovinae (Bibi, Reference Bibi2013; Druica et al., Reference Druica, Ciorpac and Cojocaru2016). At first sight, the message about the evolution of the Bovini does not appear to have changed much since publications by Sinclair (Reference Sinclair1977) and Gentry and Gentry (Reference Gentry and Gentry1978). Yet there is now perhaps more reason to consider the Bovini as a heterogeneous (non-monophyletic) group, the African buffalo not being closely related to either the water buffalo of Asia (Bubalus) or oxen, bisons and yaks. Perhaps it deserves a special tribe, Syncerini, but the evolution of the Bovini is still shrouded in much uncertainty. Five insights play havoc. First, phylogenies based on molecular markers rely heavily on available genetic material. For bovids, to date this material has been taken from living and thus contemporary specimens; fossil material does not yet play a role, except for some very recently extinct species. This means that for extinct tribes or even subfamilies there is no genetic information that has the potential to upset phylogenies that are based on parsimonious calculus (cf. Frantz et al., Reference Frantz, Schraiber and Madsen2013; Table 2.1). Second, the phylogeny based on mitochondrial DNA shows a short period around 18–15 Myr in which the Boselaphini, Tragelaphini and Bovini separated (Hassanin, Reference Hassanin, Melletti and Burton2014; Zurano et al., Reference Zurano, Magalhãesa and Asato2019). It should be realized, however, that the phylogenetic trees based on DNA suggest such divergence to have taken place some 10 million years before the oldest finds of Tragelaphini (second half Late Miocene, c.7 Myr) or Bovini (c.8 Myr). Furthermore, the calibration of the molecular-based phylogeny is based on fossils from other families mainly (see Zurano et al., Reference Zurano, Magalhãesa and Asato2019 for details) while fossil Boselaphini may be hard to identify, because early forms had few distinctive features. Third, the fossil material itself may indicate that Bovini evolved from Boselaphini several times and not just once (Gentry, Reference Gentry, Werdelin and Sanders2010). In fact, evidence for this is very slender, but this may nevertheless still be true because there is no evidence that early African Bovini (which are rare and poorly known) are derived from Asian forms. It is quite possible that they derived directly from African Boselaphini (close to Tragoportax; see Figure 2.1). Fourth, the number of Bovid species recognized in the fossil material is strongly determined by sampling effort, and there are many more sites for some periods than for others (Patterson et al., Reference Patterson, Faith, Bobe and Wood2014). Lastly, within the Bovini tribe there is a worrying lack of clarity about not only the proper naming of species and genera in the fossil material, but also whether particular fossil species and their living descendants should be taken to belong to a particular genus or to another. Much dust has been stirred up on the systematic position of Pelorovis. Was it a distinct genus? Did it belong to the genus Bos? Did it belong to the genus Syncerus? Yet if animal populations cannot be classified into valid species and allocated precise generic status, then concepts like ‘competitive exclusion’ or ‘niche differentiation’ become very difficult to apply.

Figure 2.1 Phylogeny of the Bovini and Syncerini. During the Pleistocene members of the genus Bos ventured into Africa too (see text). The separation between Boselaphini and Bovini or Syncerini is very unclear.
The Genus Pelorovis and the Syncerus antiquus Conundrum
We start with Pelorovis and the issues surrounding its phylogenetic position to better understand the evolution of Syncerus. The most important insight that emerges is that there was a second species of Syncerus, namely S. antiquus, in much of Africa that went extinct only very recently, in the last two millennia. It overlapped in space and in time with the extant African buffalo.
Hans Reck started the excavations in Olduvai (Tanzania) and found the remains of a large mammal, which he named Pelorovis (‘frightful sheep’). Later, Gentry (Reference Gentry1967) classified Pelorovis with the Bovini, but thought it to be very distantly related to the Asian Bovini. Pelorovis may have been derived from Simatherium (Geraads, Reference Geraads1992) like the African buffalo Syncerus. The difficulty of Bovini classification is well underscored by the struggle palaeontologists have in allocating the different species of Pelorovis to their classificatory nook: does a fossil belong to Pelorovis or to Bos or Syncerus, and, alternatively, should the genus Pelorovis be seen as an independent genus, or do the species of this genus better fit in Bos or Syncerus? Indeed, an identical specimen may be classified as Pelorovis or as Simatherium (Gentry, Reference Gentry, Werdelin and Sanders2010), showing the opaqueness of the systematics and phylogeny of the Bovini (see Table 2.1).
Table 2.1 Interplay between palaeontology and genetics to deduce a reliable phylogeny. The trade-off one makes between knowledge from genetics and palaeontological knowledge is not straightforward. It may upset established phylogeny, yet it may also strengthen it. If knowledge from palaeontology and genetics (if these have been reached independently) overlap, inference about the past is very strong. If there are mismatches between the two fields of enquiry, a research strategy can be formulated once one realizes the mismatch.
Genetics | ||||
---|---|---|---|---|
Species that have been allotted an unquestionable place in a phylogeny, thus ‘knowns’ | Species of which the place in a phylogeny depends on a priori choices | Species of which the genetics has not yet been carried out, thus ‘unknowns’ | ||
Palaeontology | Knowns (i.e. species that have been found and have been classified with confidence) | If there is match, we have reached a true justified belief (the hallmark of good science). If the two do not match, both fields of knowledge have to actively work together to solve the issues. Exciting new insights can arise: e.g. on the origin of Bison bonasus as a possible hybrid species of Bison priscus and Bos primigenius | Genetics should follow palaeontology and recalculate phylogenies. Bayesian approaches should incorporate prior knowledge from palaeontology | Here future progress in palaeo-DNA will perhaps make very unexpected changes |
Uncertain (i.e. species that have been found but about which the classification is unsure) | Palaeontology should incorporate knowledge from genetics to decide on the best place of such a species in an existing phylogeny | Danger exists that there is false certainty in published phylogenies by geneticists | A general state of ignorance predominates | |
Unknowns (i.e. species that have not yet been found or identified) | Phylogenies based on absent species may give a false sense of certainty | Phylogenies based on absent species may give a false sense of certainty | ‘Unknown unknowns’, which may upset any established phylogeny |
Seven species of Pelorovis have been named. Pelorovis oldowayensis is the best-known form; it has long, regularly curved horncores, first emerging almost posteriorly but recurving forwards, with a total span that can reach 2 m. It is best represented in Olduvai, but also in other Eastern African sites and in Israel (Geraads, Reference Geraads and Tchernov1986). Pelorovis turkanensis has shorter horns; it overlaps in time with the former species, but appears earlier. The North African ‘Bos’ bubaloides, ‘Bos’ praeafricanus and Pelorovis howelli (Hadjouis and Sahnouni, Reference Hadjouis and Sahnouni2006) are almost certainly identical with one or the other East African forms. Pelorovis kaisensis from Uganda and perhaps Ethiopia differs in its virtually straight horns (Geraads and Thomas, Reference Geraads and Thomas1994; Alemseged et al., Reference Alemseged, Wynn and Geraads2020). The origin of the genus is unclear, especially because the distinction between the earlier African Ugandax and Simatherium dwindled recently with the discovery of more fossils. The last species to go extinct was Pelorovis antiquus (a.k.a. Homoiceras antiquus, H. baineii or H. nilsonii: Rossouw, Reference Rossouw2001). However, this species may be better understood as Syncerus antiquus. Neither Gentry (Reference Gentry, Werdelin and Sanders2010) nor Klein (Reference Klein1994) were convinced that this was correct, but at present the leading verdict is that one could accept this view. S. antiquus had a wide distribution, and survived in northern Africa till recent times (Figure 2.2). A very late drawing of it may have been from Egypt just prior to the first Pharaoh from the so-named Amratian Civilization (~3600 bce ; see Childe, Reference Childe1958, figure 1.9). Lovely rock art from the desert of Algeria shows scenes, including bulls fighting (e.g. Brodrick, Reference Brodrick1948, p. 37).

(a) S. antiquus from I-n-Habeter, Mesāk, Libya.

(b) Rock engraving of S. antiquus from Tilizzāyen, Mesāk, Libya.
Figure 2.2 In rocky massifs in the Sahara, petroglyphs (engravings in the rock) of animal species are quite widespread. This rock art was made when the Sahara was covered by savannas or steppes, and thus shows many species that are now only known from the Sahel or East Africa. Among these are depictions of Syncerus (or Pelorovis) antiquus, which is now extinct but was once widespread.
In the Early Pleistocene beds in Arabia, a very large bovid has been found that is classified as Pelorovis cf. oldowayensis (Thomas et al., Reference Thomas, Geraads and Janjou1998). This may be an early proof of an outward migration of members of the genus Pelorovis, together with the ‘Ubeidiya occurrence. Intriguingly, it had very large feet apparently adapted to move on soft substrates’ (Thomas et al., Reference Thomas, Geraads and Janjou1998). The case shows how nomenclature intertwines with dating: the finds described by Thomas et al. (Reference Thomas, Geraads and Janjou1998), and interpreted on the basis of morphological data as being close to P. oldowayensis, were later re-interpreted because the beds from which the fossils were extracted were dated later in time and were thus allocated to Syncerus antiquus (Stewart et al., Reference Stewart, Louys, Price, Drake, Groucutt and Petraglia2019). This latter approach to classification is, in our opinion, incorrect. Similarly, molars of a smaller species that looked like those of S. caffer were classified as S. antiquus because S. antiquus is known from south-west Asia but S. caffer is not (Stewart et al., Reference Stewart, Louys, Price, Drake, Groucutt and Petraglia2019). However, Geraads (Reference Geraads and Tchernov1986) also identified Pelorovis oldowayensis from the Early Pleistocene in a nearby area, namely Israel, and later Martínez-Navarro et al. (Reference Martínez-Navarro, Belmaker and Bar-Yosef2012) confirmed the identification, but assigned the species to Bos.
This raises the issue of the relationships of Pelorovis with Bos, a mostly Eurasian genus that includes, besides the modern cattle and aurochs, several wild, endangered southern Asian species and fossil species in the same area. In Africa, unquestionable early representatives of the genus are Bos buiaensis from Eritrea, dated to 1 Myr (Martínez-Navarro et al., Reference Martínez-Navarro, Rook, Papini and Libsekal2010), a Middle Pleistocene B. primigenius from Tunisia dated to 0.7 Myr (Martínez-Navarro et al., Reference Martínez-Navarro, Karoui-Yaakoub and Oms2014) and a species from the lower Awash Valley of Ethiopia, which is close to the southern Asian extinct species B. acutifrons (Geraads et al., Reference Geraads, Alemseged and Reed2004).
The Tunisian find is almost certainly a Eurasian immigrant (pace Martínez-Navarro et al., Reference Martínez-Navarro, Karoui-Yaakoub and Oms2014), while the fact that the Eastern African forms were found close to the Aden straits strongly suggests that they are Asian immigrants. Detailed studies of the geology of the Bab-al-Mandab (the entry to the Red Sea from the Gulf of Aden) show that the straits between the Horn of Africa and the Hadhramaut, where a shallow sill is positioned (the Hanish Sill), remained submerged during the Pleistocene (Al-Mikhlafi et al., Reference Al-Mikhlafi, Edwards and Cheng2018). Yet during glacial periods, the straits were ‘sufficiently narrow for both sides of the channel to have been visible at all times’ and only about 1–3 km wide (Lambeck et al., Reference Lambeck, Purcell and Flemming2011), thus making it feasible that Asian species of Bos crossed here into Africa. Note that the occurrence of S. c. nanus until a century ago on Bioko Island, some 35 km off the mainland in the Gulf of Guinea, cannot be taken as an example of buffalo being able to cross such a distance at sea, because Bioko Island was linked to the mainland until the beginning of the Holocene (Ceríaco et al., Reference Ceríaco, Bernstein and Sousa2020). Nevertheless, buffalo are good swimmers, and are able to cross wide rivers like the Nile and the Zambesi.
By contrast, Martínez-Navarro et al. (Reference Martínez-Navarro, Pérez-Claros and Palombo2007, Reference Martínez-Navarro, Rook, Papini and Libsekal2010) envisage an evolutionary line of the genus Bos starting as Bos (P.) turkanensis (Late Pliocene), B. (P.) oldovayensis (Early Pleistocene), B. (P.) buiaensis (Early Pleistocene) and thence Bos primigenius (the Aurochs) and also Bos planifrons (which more often is taken as the direct ancestral form of Bos primigenius namadicus – the Indian form of the aurochs which developed into Bos indicus, the zebu). The important consequence of accepting this interpretation is that the direct ancestors of cattle and zebu evolved in Africa and not in Asia. This would agree with the parsimony analysis on morphological characters performed by Geraads (Reference Geraads1992), which showed them to be close on the cladograms. However, the detailed study by Gentry (Reference Gentry1967) showed that the cranial morphology of P. oldowayensis is very different from that of Bos, and it is likely that their closeness on cladograms results from parallelisms. Furthermore, the contemporaneity of the last representatives of the former species with Bos buiaensis make an ancestral–descendant relationship extremely unlikely (Geraads, Reference Geraads, Gallotti and Mussi2018). Moreover, this reasoning sits very uncomfortably with studies that base their reasoning on genetics: B. primigenius, cattle and zebu all fit snugly within the phylogenies of the other Asian Bos species (cf. Van der Made, Reference Van der Made2013). After carefully considering the arguments and fossil material, Tong et al. (Reference Tong, Chen, Zhang and Wang2018) conclude that B. primigenius was not derived from species that have been classified as Pelorovis, and support the view that B. primigenius evolved in South Asia, as does Van der Made (Reference Van der Made2013). Likewise, Bar-Yosef and Belmaker (Reference Bar-Yosef and Belmaker2016) maintain the position that B. primigenius appeared in southwestern Asia as early as 1.2 Myr bp, and it continually occurred in this region until the Late Pleistocene. They recognize B. buiaensis in the Jordan Valley much later, namely 0.5–0.8 Myr, but as stated, this could well have been a Pelorovis. Indeed, many authors have stated that Pelorovis (Syncerus) antiquus was part of the mammal assemblage of the Pleistocene Levant.
Is there good reason to accept the view that Pelorovis antiquus should be considered as Syncerus antiquus as deduced by Peters et al. (Reference Peters, Gautier, Brink and Haenen1994) but rejected by Klein (Reference Klein1994)? The predecessor (but not necessarily ancestor) of P. antiquus was P. oldovayensis. This species was already present in the Levant (Bar-Yosef and Belmaker, Reference Bar-Yosef and Belmaker2016) and perhaps in Arabia (Thomas et al., Reference Thomas, Geraads and Janjou1998) in the Early Pleistocene. Yet, Martínez-Navarro and Rabinovich (Reference Martínez-Navarro and Rabinovich2011) argue to classify this species as S. antiquus; however, their publication does not present arguments other than opinion. The original argument put forward by Peters et al. (Reference Peters, Gautier, Brink and Haenen1994) to view P. antiquus merely as a form of S. caffer, or as a separate species S. antiquus, was mainly based on the observation that the postcranial skeleton hardly differed from S. caffer (Peters et al., Reference Peters, Gautier, Brink and Haenen1994). However, this is a weak argument, because ‘The similarity in the postcranial skeleton known from Bos, Bison and Bubalus arnee is surprising considering that, according to an analysis of mitochondrial DNA, the separation of the Bubalus–Syncerus clade from the Bos–Bison clade goes back to the Middle Miocene’ (Van der Made et al., Reference Van der Made, Torres, Ortiz, Fernández-Jalvo, King, Yepiskoposyan and Andrews2016; see also Von Koenigswald et al., Reference Koenigswald, Schwermann, Keiter and Menger2019). The main argument of Klein (Reference Klein1994) was that the two species coexisted for a long time, and if both were to be viewed as Syncerus, then that would not have been possible. This is, however, based on an old ‘rule’ of competitive exclusion formulated by Charles Darwin but for which there is no firm evidential support (Prins and Gordon, Reference Prins, Gordon, Prins and Gordon2014a, Reference Prins, Gordon, Prins and Gordon2014b). Note that species of the same genus can very well coexist, as exemplified by Lechwe and Puku or Plains Zebra and Grevy’s zebra in Africa, or for that matter by the many different Anas spp., Anser spp., Corvus spp., etc. in the Boreal zone.
Yet we also have not read convincing arguments to accept the view that Pelorovis antiquus was merely another African buffalo or even a more drought-adapted subspecies of the present-day African buffalo (cf. Peters et al., Reference Peters, Gautier, Brink and Haenen1994). Indeed, the stance one takes with respect to the systematic position of P. antiquus affects the way the evolutionary history of S. caffer is interpreted. Note that this has little to do with accepting or rejecting the narrow species concept proposed by Groves and Grubb (Reference Groves and Grubb2011, p. 1 ff.). However, Gentry (Reference Gentry, Werdelin and Sanders2010) takes P. antiquus (grudgingly) as S. antiquus, even though he does not present arguments for (or against) this view. However, this evidence is murky, because it depends so much on interpretation in the case of the fossil Bovini material. This implies that one has to consider two alternative scenarios in the evolution of Syncerus: namely, one with S. antiquus as a species coexisting with S. caffer and living in the same area as B. primigenius in northern Africa, and the other in which Syncerus never reached the areas to the north of the Sahara but that the relevant ‘buffalo’ species in that area was P. antiquus.
Miocene Origins of the African Buffalo
How far back in time can one trace the ancestry of the African buffalo? It may have appeared reasonably clear 50 years ago (Sinclair, Reference Sinclair1977, p. 22), but the crucial issue is whether the African buffalo really fits into the Bovini (together with the Asian buffalo and the wild cattle swarm). On the basis of DNA, it can be deduced that the last common ancestors of the Bovini and the Tragelaphini (species like the present-day kudu, bushbuck and eland antelope) lived some 18 Myr (Bibi et al., Reference Bibi, Bukhsianidze and Gentry2009) or 15 Myr ago (Zurano et al., Reference Zurano, Magalhãesa and Asato2019), but the first fossil material comes from Eotragus, which is classified as a Boselaphine (like the present-day nilgai). Between the oldest species, E. noyei from Pakistan (18 Myr), and the next species, E. sansaniensis from France (15.2 Myr), there is a gap of 3 million years, which is as long as the duration of the entire Pleistocene (Solounias and Moelleken, Reference Solounias and Moelleken1992). Then there is another enormous time gap of some 6 million years to a genus named Selenoportax/Pachyportax, again from Pakistan (9 Myr; Bibi et al., Reference Bibi, Bukhsianidze and Gentry2009). An ancestral relation between Pachyportax and Parabos (thought to be ancestral to Leptobos, Bos and Bison and perhaps to Proamphibos leading to Bubalus) has been surmised, but the evidence is weak. From Pachyportax onwards, the fog of the fossil record lifts a bit. But just when one seemed to be back on firm footing, Gentry (Reference Gentry, Werdelin and Sanders2010) dropped a bombshell by pointing out that there is a fair chance that the Bovini are not even monophyletic. Indeed, Geraads (Reference Geraads1992) had already shown that the relationship between Asian and African buffalo is not well supported. In other words, after decades of hard field work and thinking, the early history of the Bovini is not yet clear regardless of what phylogenies based on present-day DNA seem to suggest. Later we will show that cross-fertilization data between African and Asian buffalo also point to a very weak relationship within the group of organisms that are classified as Bovini.
The genus Eotragus was a long-lived one with a very wide distribution, ranging from Europe to China, Pakistan and Israel to Kenya (Solounias et al., Reference Solounias, Barry, Bernor, Lindsay and Raza1995). The genus Tethytragus was similar to Eotragus, but evolutionary perhaps not a Boselaphine, and even though T. langai still falls within the class of brachydont herbivores, it was more hypsodont than Eotragus and may already have been a grazer (DeMiguel et al., Reference DeMiguel, Azanza and Morales2011, Reference DeMiguel, Sánchez and Alba2012). Yet it appears that the early ‘invasion’ of Africa by Boselaphini at the beginning of the Middle Miocene did not lead to today’s Bovini in Africa. They may have arisen from a second ‘invasion’ of Boselaphini at the end of the Middle Miocene (Thomas, Reference Thomas1984; Gentry, Reference Gentry, Werdelin and Sanders2010).
The next genus to consider is Pachyportax, which lived during the end of the Miocene. The genus has also been classified within the Boselaphini, but it appears that the Boselaphini are not a homogeneous tribe (Bibi et al., Reference Bibi, Bukhsianidze and Gentry2009). Pachyportax latidens was a large Boselaphine during the Late Pliocene (7–3.5 Myr) of the Siwalik Hills of Pakistan with strongly developed molars for chewing roughage (Ikram et al., Reference Ikram, Safdar and Babar2017). At the same time, there was another Boselaphine in the Siwaliks with less hypsodont molars, which was of the genus Tragoportax. European Tragoportax at least are large forms, and have rather long legs (perhaps similar to the nilgai). There were quite a number of other putative Boselaphini species at that time in the Siwalik mammal assemblage (Batool et al., Reference Batool, Khan and Babar2016), but whether they were truly Boselaphine is uncertain (Bibi et al., Reference Bibi, Bukhsianidze and Gentry2009). Miocene Bovini show mesowear patterns that are similar to present-day browsers and mixed-feeders, and the molars were not yet very hypsodont (Bibi, Reference Bibi2007). Indeed, Solounias and Dawson-Saunders (Reference Solounias and Dawson-Saunders1988) elegantly showed how masticatory morphology features relating to intermediate feeding and grazing adaptations evolved in parallel several times and independently from primitive browsing conditions. According to these authors, this did not happen in a savanna-type landscape but in the broad-leaved forests and woodlands there (in Greece). Bibi’s (Reference Bibi2007) palaeoecological reconstruction is that these early Bovini started utilizing open C3 vegetation with C3 grasses. Indeed, C4 grasses became important only later (Barry et al. Reference Barry, Morgan and Flynn2002), and Bibi (Reference Bibi2007) speculates that because the hypsodont index only reached values indicating pure grazing around 8 Myr ago, this behaviour started with the emergence of C4 grassland at that time. However, the abrasion patterns of the molars do not support this (Bibi, Reference Bibi2007). The driving evolutionary force may have been the strengthening of the monsoonal system due to the uplift of the Tibetan Plateau (Searle, Reference Searle, Prins and Tsewang2017) leading to resource scarcity during the dry season (Bibi, Reference Bibi2007). The fire-dominated and grazer-induced grasslands came into existence only about 2 Myr ago in Africa (Spencer, Reference Spencer1997).
In Libya, Tragoportax cyrenaicus lived about 7 Myr ago; the species was perhaps derived from the West Eurasian form (Gentry, Reference Gentry, Werdelin and Sanders2010). From South Africa, T. acrae has been reported (also known as Mesembriportax acrae, but cladistically sitting more comfortably with Tragoportax: Spassov and Geraads, Reference Spassov and Geraads2004). Tragoportax had a very large range, from Spain to China, and from southern Asia to southern Africa (Batool et al., Reference Batool, Khan and Babar2016). In the Siwaliks, the lineage of Tragoportax changed from a C3 browser at 8 Myr to a C4 grazer at 7.5 Myr. By 6.5 Ma, most frugivores and/or browsers had disappeared even though areas of C3 vegetation remained until at least 4.5 Myr on the flood plain (Patnaik, Reference Patnaik, Wang, Flynn and Fortelius2013; cf. Saarinen, Reference Saarinen, Gordon and Prins2019).
Sinclair (Reference Sinclair1977, p. 22), based on Pilgrim (Reference Pilgrim1939) and Thenius (1969, cited in Sinclair, Reference Sinclair1977), suggested that Parabos was the ancestor of the African Bovini (Pelorovis, Syncerus) but also of the Eurasian Bos and Bubalus. The fact that much older Bovini have been found in Pakistan, namely some 8 Myr ago (Bibi, Reference Bibi2007), and that no Parabos has been found outside Europe and the Middle East, pleads against accepting the genus Parabos as ancestral to modern Bovini. This is reinforced by the fact that it seems to be seen better as belonging to the Boselaphini than to the Bovini (Gromolard, Reference Gromolard1980; Gromolard and Guerin, Reference Gromolard and Guérin1980; Geraads, Reference Geraads1992). Moreover, Parabos still occurred much later in time than the separation of Syncerus and Bubalus. It appears that Boselaphines disappeared from the African continent at the end of the Miocene (Gentry, Reference Gentry, Bubeník and Bubeník1990; Bibi, Reference Bibi2007 – the Miocene ends 5.3 Myr), unless there was a lineage leading to the present-day African buffalo.
The Pliocene Ancestors of Syncerus
Genetic data suggest a separation of Bubalus and Syncerus some 8.8 Myr ago (Hassanin, Reference Hassanin, Melletti and Burton2014) or even a million years earlier (Zurano et al., Reference Zurano, Magalhãesa and Asato2019), or (on the basis of cytochrome-c analyses) some 6 Myr ago (Druica et al., Reference Druica, Ciorpac and Cojocaru2016), thus in the Miocene. Among the oldest African Bovines, Ugandax cf. gautieri (see Thomas, Reference Thomas1984) has been reported from Lukeino, as early as about 6 Myr (Pickford and Senut, Reference Pickford and Senut2001); this species had much morphological similarity with Simatherium demissum from South Africa (Thomas, Reference Thomas1984; cf. Geraads, Reference Geraads1992). Ugandax may have been derived from the Selenoportax–Pachyportax lineage from the Siwaliks (Thomas, Reference Thomas1984; Gentry, Reference Gentry, Werdelin and Sanders2010), but Bibi (Reference Bibi2009, p. 332) states that it was also very similar to Proamphibos lachrymans (the putative ancestor of the Asian buffalo). Bibi (Reference Bibi2009, p. 339) poses that Proamphibos lachrymans was the last common ancestor of the African and Asian buffalo. Proamphibos was substantially larger than Pachyportax (Bibi, Reference Bibi2009, p. 339).
There was a suite of species within the genus Ugandax or closely related (U. [S.] demissum from Early Pliocene South Africa; U. coryndonae from the Middle Pliocene, Ethiopia; U. gautieri from Uganda, of about 5 Myr; Simatherium kohllarseni from the Middle Pliocene of Tanzania and Kenya; and S. shungurense from the Late Pliocene of Ethiopia; Geraads et al., Reference Geraads, Blondel and Mackaye2009a). Yet the evolutionary link between Ugandax–Simatherium and Syncerus also is not well supported by cladistic analyses (Geraads, Reference Geraads1992).
Ugandax coryndonae is perhaps the best known of the Pliocene African Bovini, represented by a large number of specimens from Ethiopia (Gentry, Reference Gentry2006; Geraads et al., Reference Geraads, Melillo and Haile-Selassie2009b, Reference Geraads, Bobe and Reed2012). This species may have lived until the Pleistocene, 2.5 Myr ago (Bibi, Reference Bibi2009, p. 335). In other words, the notion that Ugandax could have given rise to Syncerus (Gentry, Reference Gentry2006) is not well supported by cladistic analysis, and is further undermined by the earliest records of Syncerus perhaps overlapping in time with those of Ugandax (Gentry, Reference Gentry, Werdelin and Sanders2010; Bibi et al., Reference Bibi, Rowan and Reed2017).
The deduction that a Middle Pliocene emigration took place by a Syncerus-type buffalo from Africa into the Caucasus (Vislobokova, Reference Vislobokova2008), and from there to Eastern Europe (Evlogiev et al., Reference Evlogiev, Glazek, Sulimski and Czyzewska1997), by a species classified as Eosyncerus ivericus is most likely not justified because the material appears to be Caprine (Bukhsianidze and Koiava, Reference Bukhsianidze and Koiava2018).
So, back to Proamphibos. During the Pliocene, this large bovine lived in the foothills of the Himalayas and the floodplains of the Indus and Ganges (Khan et al., Reference Khan, Iqbal, Ghaffar and Akhtar2009). Two species have been distinguished, namely, the less advanced form (with regards to skull and horn morphology) P. lachrymans and the more advanced P. kashmiricus (Pilgrim, Reference Pilgrim1939; Khan and Akhtar, Reference Khan and Akhtar2011). The body mass of Proamphibos was about 200 kg (Dennell et al., Reference Dennell, Coard and Beech2005). Later (i.e. younger) finds of P. lachrymans have been reclassified as Damalops palaeindicus, not belonging within the Bovini but to the Alcelaphini (the hartebeest group); the presence of Proamphibos as late as 0.8 Myr ago is thus factually refuted. Apparently, it did not co-occur with Hemibos (neither with H. acuticornis nor with H. triquetricornis) and also not with Bubalus in Siwalik deposits (Badam, Reference Badam1977: his table 2; also, in figure 17.11 of Patnaik, Reference Patnaik, Wang, Flynn and Fortelius2013). The genus Proamphibos is thus considered to be more ancient than the genus Hemibos (cf. Bibi, Reference Bibi2009, p. 338). The genus Hemibos was considered to include the direct ancestor of Bubalus, and perhaps especially of the Anoa of Sulawesi (Groves, Reference Groves1976). Evidence of co-occurrence of Hemibos with Bubalus, however, pleads against this. There is no evidence that members of the genus Hemibos, which appears to have derived from Proamphibos, migrated to Africa or were involved in any way in the evolution of African Bovini and Syncerus in particular.
An independent lineage, not leading to Syncerus but perhaps related, was present in northern Africa in the form of Leptobos syrticus. Gentry (Reference Gentry, Bubeník and Bubeník1990), Duvernois (Reference Duvernois1992) and Geraads (Reference Geraads1992) concluded that it should not be maintained within the genus Leptobos; they prefer to not assign it to a genus, but conclude a similarity with Syncerus. ‘Leptobos’ syrticus may be related to Jamous kolleensis from Pliocene Chad, but this latter species does not show clear affinity with Syncerus (Geraads et al., Reference Geraads, Blondel and Mackaye2009a). Jamous kolleensis was a medium-sized bovine, still with rather primitive molars (Geraads et al., Reference Geraads, Blondel and Mackaye2009a). Because the Eurasian genus Leptobos, so important for understanding the evolution of Bos including Bison, apparently did not otherwise play a role in the evolution of Pelorovis or Syncerus, we do not deal with it in this chapter.
Thus, Proamphibos, or less likely Ugandax, is perhaps the link between Asian and African buffalo that geneticists identified to have lived some 8 Myr ago. Cladistic analysis of many fossil forms, modern Bubalus and modern Syncerus do not well support a strong relationship between Asian and African buffalo (Geraads, Reference Geraads1992). A putative separation some 8 Myr ago is an ancient one for mammals in contrast to birds because the former have prezygotic and postzygotic barriers and the latter prezygotic ones only. These postzygotic barriers are confirmed through embryo transfer experiments (see below), so the genetic distance is really to be reckoned in millions of years. On the basis of a careful analysis of karyotype evolution, it also appears that African and Asiatic buffalo evolved along two different and independent routes, as their centric fusions involved different homoeologous chromosomes (Iannuzzi et al., Reference Iannuzzi, King and Di Berardino2009).
African Buffalo Syncerus caffer – Pleistocene and Holocene Fossil Material
So, neither a cladistic analysis of many fossil and modern forms nor studies on nuclear DNA and embryology support a strong relationship between African and Asian buffalo. The ancestry of Asian buffalo, through its descending from Hemibos, which was derived from Proamphibos, appears reasonably well-founded. The ancestry of the African buffalo is shrouded in opacity. As sketched out, the Pliocene forms Ugandax led to Simatherium and may have led from there to Syncerus, but this link is not well supported by cladistic analyses (Geraads, Reference Geraads1992). Fossil Syncerus, such as at Shungura and Olduvai, had no large basal bosses (as the modern Cape buffalo Syncerus c. caffer) (Gentry, Reference Gentry, Bubeník and Bubeník1990). Gentry even states that these Simatherium were small and short-horned similar to the forest buffalo S. c. nanus of today. Whether they form an unbroken lineage to the present forest buffalo is not known, but this is very unlikely given the way that S. c. nanus is genetically nested within the other living African buffalo (Van Hooft et al., Reference Van Hooft, Groen and Prins2002). Recent genetic studies (reviewed in Prins and Sinclair, Reference Prins, Sinclair, Kingdon and Hoffmann2013) suggest that S. c. nanus is the older form and S. c. caffer only arose some 150,000 years ago. Whether the two forms (a nanus-like one and a caffer-like one), as suggested by Gentry (Reference Gentry, Bubeník and Bubeník1990), really have been present for a long time seems to be contradicted by genetic analyses (see e.g. Van Hooft et al., Reference Van Hooft, Groen and Prins2002). In Chapter 8, Prins, Ottenburghs and Van Hooft revise their opinion, and conclude that S. c. nanus is a derived form, while S. c. aequinoctialis may be closest to the ancestral form.
The first species that can be classified as Syncerus may have been Syncerus acoelotus. Geraads et al. (Reference Geraads, Blondel and Mackaye2009a) state that it was as large as the modern S. caffer but with less-advanced horns. However, because fossils are not plentiful and the remains are fragmentary, classification remains fraught with issues. Indeed, Gentry (Reference Gentry, Coppens and Howell1985) compared Shungura Member C (~2.7 Ma) Syncerus horn cores to those of Syncerus acoelotus, named from the much younger Olduvai Bed II (~1.5 Ma), but later, Gentry (Reference Gentry, Werdelin and Sanders2010) referred to them as Simatherium shungurense. Bibi et al. (Reference Bibi, Rowan and Reed2017) re-examined some of the Shungura material and state that they prefer Gentry’s (Reference Gentry, Coppens and Howell1985) opinion, so they choose to see these fossils again as Syncerus acoelotus. A possible very early find of S. caffer is from northern Sudan near Dongola; the authors were convinced it was not a Pelorovis (S.) antiquus but a true African buffalo (Chaix et al., Reference Chaix, Faure, Guerin and Honegger2000), but the age of the site is poorly supported. We are not aware of any palaeontological material that can be ascribed to some of the other existing forms of S. caffer, to wit S. c. mathewsi or S. c. brachyceros. Unless material is unearthed, one has to rely on genetic analyses to reconstruct the history of the morphological differentiation within the species. The scant sample sizes on morphology that Groves and Grubb (Reference Groves and Grubb2011, p. 122 ff.) rely on to distinguish S. brachyceros or S. mathewsi as separate species are certainly not convincing.
We mentioned earlier that phylogenies based on DNA do not take into consideration the DNA sequences of extinct species if genetic material is no longer available (see Table 2.1). So even where, for example, Bibi (Reference Bibi2013) took into account three Bubalus species (when there are five or six) into his phylogeny, he did not (and could not) include a whole suite of recently extinct species (some 10 from China: Dong et al., Reference Dong, Liu, Zhang and Xu2014) or the three species that went extinct 2–1 Myr ago (from southern Asia: Van den Bergh et al., Reference Van den Bergh, de Vos and Sondaar2001; Patnaik, Reference Patnaik, Wang, Flynn and Fortelius2013). This relative ‘blindness’ may cause an optimally parsimonious phylogeny to be an imperfect reconstruction of evolution in reality. This is no criticism of such work, to the contrary, but a call for even better integrating palaeontology with genetics (Table 2.1).
The whole group of (wild) cattle and bison combines well, but ancestors of the wild South-East Asian cattle, bison and West Asian cattle apparently speciated at one short period of time, which cannot be resolved hierarchically (MacEachern et al., Reference MacEachern, McEwan and Goddard2009). A major issue is extensive hybridization between the whole group of cattle, zebu, yak, gaur, banteng, wisent and bison. Indeed, closely related species (as established by genetic analyses) show hardly any or no barriers to cross-breeding. Species that diverged longer ago show infertility in the male offspring but none in the female offspring. Back-crosses are then very well possible, and this may explain the frequently observed introgression of genetic material in one species from another. Species that are only distantly related cannot cross-breed; in a number of cases, it has been found that in-vitro fertilization is then possible, but the embryo only survives briefly in vitro. These results are further supported by embryo transplantations of ‘normal’ embryos of one species implanted into a cow of another species.
As expected, this technique shows that embryos of Bos taurus indicus transferred to B. t. taurus cows result in fully normal parturitions (Summers et al., Reference Summers, Shelton and Edwards1983). Likewise, B. gaurus embryos have been transferred to B. taurus cows without any problems (Stover et al., Reference Stover, Evans and Dolensek1981). However, pregnancy of embryos of Bison bison that were transferred to B. taurus cows were terminated sometime between 60 and 100 days (Dorn, Reference Dorn1995). This does not mean that they are not frequently born, because they are, and are named ‘beefalo’. Sanders (Reference Sanders1925) already reported that male offspring of bison–cattle hybrids (at that time named catalo) frequently were either aborted, stillborn or died very young. Crosses between yak and cattle also often result in increased abortion (Zhang, Reference Zhang, Zhao and Zhang2000), yet the offspring that survives is valuable, because they are strong (personal observation).
Water buffalo and cattle are genetically much more distant. Indeed, the pregnancy of Bubalus bubalis embryos transferred to B. taurus cows terminated after 37 days (Drost et al., Reference Drost, Wright and Elsden1986). After in-vitro fertilization, embryos of crosses between cattle and water buffalo only survive to the blastocyst state (Kochhar et al., Reference Kochhar, Rao and Luciano2002), and to the morula state only in in-vitro fertilization of cattle with African buffalo sperm (Owiny et al., Reference Owiny, Barry, Agaba and Godke2009). Indeed, African buffalo are more distantly related to the other Bovini than to Asian buffalo.
In other words, prezygotic barriers are nearly absent between the different species of Bos and Bison, but postzygotic barriers become increasingly severe with increasing genetic (and evolutionary) distance. We deduce from this that postzygotic barriers become an overwhelming barrier between Bovini that are separated by more than 5 Myr or more, and that prezygotic barriers become an issue after a divergence of some 2 Myr. This appears to be about the same as in wild pigs (Sus; Frantz et al., Reference Frantz, Schraiber and Madsen2013), and very different from birds like ducks (Kraus et al., Reference Kraus, Kerstens and van Hooft2012) or geese (Ottenburghs et al., Reference Ottenburghs, Kraus and van Hooft2017), where postzygotic barriers do not play a (major) role against horizontal gene transfer (see also Syvanen, Reference Syvanen2012; Stewart et al., Reference Stewart, Louys, Price, Drake, Groucutt and Petraglia2019). Because the Bovini hold much interest in terms of livestock production, perhaps more is known about ‘evolution in progress’ with this species group than with nearly any other. The picture that emerges is not a simple evolutionary tree, but a system more akin to ‘reticulated evolution’ (Buntjer et al., Reference Buntjer, Otsen and Nijman2002).
Using microsatellite data, Ritz et al. (Reference Ritz, Glowatzki‐Mullis, MacHugh and Gaillard2000) put forward that some 2.5 million years ago, water buffalo and African buffalo had a common ancestor. Their data show that the genetic distance between African buffalo and species of the genus Bos appears to be equal. More recent research not using microsatellites but nuclear genome sequences suggests that the groups (Bubalus plus Syncerus) and (Bos plus Bison) split very much earlier, namely around five to nine million years ago (Bibi, Reference Bibi2013). The findings of Ritz et al. (Reference Ritz, Glowatzki‐Mullis, MacHugh and Gaillard2000) are even more difficult to understand if one realizes that a short genetic distance can point to hybridization. Hybridization between Syncerus and Bos, however, is very unlikely given the outcome of the fertilization and transplantation experiments alluded to above. An alternative explanation is that because these two genera split relatively recently, the genetic makeup is so similar because of incomplete lineage sorting (MacEachern et al., Reference MacEachern, McEwan and Goddard2009; Bibi, Reference Bibi2013).
Perhaps the true phylogenetic relationship must be derived through other techniques, as was done by Buntjer et al. (Reference Buntjer, Otsen and Nijman2002). They used amplified fragment length polymorphism (AFLP) to generate nuclear DNA fingerprints that display variation of loci dispersed over the nuclear genome of the different species. They did not use algorithms that necessitate solving a tree, and also think that a ‘consequence of reticulation is that a tree topology is not adequate for representing the phylogeny’. The Bovini thus form a prime case of ‘evolution in action’ in which there is a hugely successful group of morphologically very distinct species through which exchange of adaptive or non-adaptive genes can move within the ‘supra species’ Bos (sensu Kraus et al., Reference Kraus, Kerstens and van Hooft2012). However, the African buffalo is not part of the species swarm of cattle, gaur, zebu, banteng, yak, wisent and bison that form the Bovini. It is evolutionarily so far removed from that group of Palaearctic and Oriental Bovini that it may be thought as a single surviving species in a tribe ‘Syncerini’. Does that have any repercussions for understanding their ecology or management better? We seriously doubt this, because the amount of ecological knowledge garnered from wild Asian buffalo in their native environment is negligible. The wild Asian species is nearly extinct, and little progress has been made to reintroduce them into the wild. In other words, the African buffalo may be irreplaceable and for understanding it, one cannot plagiarize knowledge from other Bovini.
Introduction
Because the African buffalo (Syncerus caffer) exhibits extreme morphological variability across its range (e.g. body size and weight, coat colouration, horn size and curvature), its taxonomic status has been the subject of many debates over time (reviewed in Chapter 2). The most recent update of the IUCN Red List recognized four African buffalo subspecies: S. c. nanus, S. c. brachyceros, S. c. aequinoctialis and S. c. caffer. Two genetic clusters can be identified based on maternally inherited mitochondrial DNA (mtDNA): one cluster encompassing the three subspecies from West and Central Africa (S. c. nanus, S. c. brachyceros, S. c. aequinoctialis); the other cluster consisting of the S. c. caffer subspecies from East and Southern Africa. The amount of genetic differentiation between these two clusters is typical of that of subspecies in other African bovids (Smitz et al., Reference Smitz, Berthouly and Cornélis2013). The same picture emerges with the paternally inherited Y-chromosome: three haplotypes (genetic variants) among West and Central African populations and one unique haplotype among East and Southern African populations (Van Hooft et al., Reference Van Hooft, Groen and Prins2002). Thus, with both mtDNA and Y-DNA S. c. caffer emerges as a distinct genetic cluster. The only exception may be S. c. caffer in Angola and Namibia. There, two mtDNA haplotypes and one Y-haplotype typical of West and Central Africa were observed (Van Hooft et al., Reference Van Hooft, Groen and Prins2002). However, these latter observations should be taken with caution considering these genotypes were derived from zoo animals.
Nevertheless, the spatial genetic pattern based on microsatellites (polymorphic genetic markers residing on non-sex chromosomes) is different. Among S. c. caffer populations, genetic variation is mainly clinal (Van Hooft et al., Reference Van Hooft, Getz and Greyling2021). This clinal variation is characterized by a linear relationship between genetic distance (pairwise FST: the proportion of the total genetic variation per population pair, that is between the two populations) and geographic distance, a pattern also known as isolation-by-distance, with the latter explaining as much as 78 per cent of the variation. This clinal pattern even extends to the populations of S. c. brachyceros and S. c. aequinoctialis, which like S. c. caffer also occur on savannas (R2 = 0.83, Figure 3.1). Predicted pairwise FST gradually increases to ~0.15 at 5,300 km. Genetic distances involving the S. c. nanus population from the Central African Republic (Ngotto Forest Reserve) are also clinal (R2 = 0.85, Figure 3.1), but twice as large in comparison to those involving only savanna-dwelling populations. This is probably due to a combination of low population density and reduced gene flow in rainforests compared to savannas. The only exceptions to these clinal patterns are populations with elevated FST values (FST > 0.2 beyond 2000 km distance; not shown in Figure 3.1) due to small size, isolation or a bottleneck, as observed with the populations from HiP (Hluhluwe-iMfolozi Park, South Africa; Van Hooft et al., Reference Van Hooft, Getz and Greyling2019), Nairobi National Park (Kenya; Heller et al,. Reference Heller, Okello and Siegismund2010) and Lékédi Park (Gabon).

Figure 3.1 Increase of pairwise FST with geographic distance (isolation-by-distance): among savanna-dwelling populations (i.e. excluding S. c. nanus): R2 = 0.83 (solid line), between the S. c. nanus population from Central African Republic (C.A.R.) and the savanna-dwelling populations: R2 = 0.85 (dashed line). Regression is weighted by ‘square root of number of genotyped individuals per population pair X number of shared genotyped microsatellites per population pair’. Only population pairs are included with weight >102 in case of savanna-dwelling populations and with weight >48 in case pairs including the S. c. nanus population from C.A.R. In all cases, sample size per population ≥5 with number of microsatellites per population pair varying between 8 and 18. Data from Van Hooft et al. (Reference Van Hooft, Getz and Greyling2021) and unpublished data from Smitz et al. (Reference Smitz, Cornélis, Chardonnet, Melletti and Burton2014b). Genotype data came from different laboratories, which when also coming from the same population permitted allele alignment by matching each microsatellite’s allele frequencies while preserving size order.
Thus, at the level of neutral genetic markers in savanna-dwelling buffalo, neither the subspecies nor buffalo in the contact zones between them appear as distinct genetic clusters. As has been proposed in human genetics (Handley et al., Reference Handley, Manica and Goudet2007), one should abandon the traditional island model of population differentiation (treating populations as discrete random mating units) when explaining genetic structure in relation to historical gene flow (in the case of African buffalo before 1870). The observed linear relationship between genetic and geographic distance indicates that, historically, the savanna-dwelling buffalo populations constituted one large metapopulation with continuous gene flow over limited distance, in which ‘limited’ is defined as less than the lifetime dispersal distance.
The clinal pattern of genetic variation seems to be in conflict with studies that describe population genetic structure as discontinuous or clustered (Heller et al., Reference Heller, Okello and Siegismund2010; Smitz et al., Reference Smitz, Cornélis and Chardonnet2014a). It is possible that genetic clusters are an artefact of a discontinuous sampling scheme (Pritchard et al., Reference Pritchard, Stephens and Donnelly2000; Kopec, Reference Kopec2014). On the other hand, clinal and clustered depictions of genetic structure are not necessarily mutually exclusive (Handley et al., Reference Handley, Manica and Goudet2007). Genetic structure may also be described using a synthetic model, in which most population differentiation can be explained by gradual isolation-by-distance, with some discontinuities due to historical or recent geographic barriers (e.g. human-induced population fragmentation). However, clusters probably explain only a small fraction of the variation when there is a strong underlying pattern of isolation-by-distance; a fraction which in case of African buffalo is no more than 0.17 (1 minus R2) (Handley et al., Reference Handley, Manica and Goudet2007).
The question of how many subspecies of buffalo can be recognized depends on the subspecies concept to which one adheres. If one merely relies on the notion of heritable geographic variation in phenotype (Patten, Reference Patten2015), then almost any number of subspecies can be justified, as long the phenotypic traits used in subspecies designation are heritable and confined to specific areas. On the other hand, if one uses partial restricted gene flow and clearly delineated genetic clusters as additional criteria (Haig et al., Reference Haig, Beever and Chambers2006), then no more than three subspecies may be recognized: (1) S. c. caffer of the East and Southern African savannas (a separate cluster with mitochondrial and Y-chromosomal markers), (2) S. c. nanus of the West and Central African rainforests (restricted gene flow indicated by relatively high FST values) and (3) the northern savanna buffalo of the West and Central African savannas (currently assigned to two different subspecies: S. c. brachyceros and S. c. aequinoctialis). Prins et al. (Chapter 2) propose to name the latter Syncerus caffer umarii. Considering that S. c. nanus is not phylogenetically distinct from the northern savanna buffalo, one may even argue that all of the buffalo from West and Central Africa, irrespective of habitat, should be lumped into one subspecies as suggested in Smitz et al. (Reference Smitz, Cornélis and Chardonnet2014a). Irrespective of subspecies designation, which appears quite subjective according to the selected criteria and to the interpretation of the obtained results, the West and Central African buffalo should be recognized as a separate Conservation Unit (see next section).
Phylogeography and Evolutionary History of the African Buffalo
Phylogeography is the study of the geographic distribution of genetic lineages (Avise, Reference Avise2000). As mentioned above, the African buffalo is genetically divided in two main lineages, one encompassing the buffalo distributed in West, Central and possibly southwestern Africa (Angola and Namibia; hereafter called the WC cluster) and another one including buffalo roaming East and southern African savannas (hereafter referred to as the ES cluster). This clear genetic discontinuity has led to the recognition of two management units (Moritz, Reference Moritz1994) deserving specific conservation efforts (Van Hooft et al., Reference Van Hooft, Groen and Prins2002; Smitz et al., Reference Smitz, Berthouly and Cornélis2013). Each management unit is characterized by a unique evolutionary history, which can be investigated using molecular tools. In fact, genomes retain records of demographic changes and evolutionary processes that have shaped present-day diversity within the species. Reconstructing the species’ evolutionary history allows us to determine the effect of recent and past climatic events, as well as of human activities. Over the last decades, some congruent results were obtained when investigating the signature left in the buffalo genomes by past and recent events using various DNA markers (i.e. mtDNA fragments, Y-chromosomal loci, autosomal microsatellites, mitogenomes and whole genomes). In this section we review the present understanding of the effect of these events in a chronological way (from the past to the recent). However, note that inferring history and linking demographic changes to specific historical events can hardly be achieved with more than some thousand years of certainty.
The species is widespread in sub-Saharan Africa, physically able to disperse through a wide range of habitats, from sea level to the limits of forests on the highest mountains (Sinclair, Reference Sinclair1977; Prins, Reference Prins1996) and morphologically able to rapidly adapt in evolutionary terms to different ecological conditions (Smitz et al., Reference Smitz, Berthouly and Cornélis2013). Its distribution is limited by the availability of permanent sources of water. Drought is considered to be a major cause of ungulate mortality, with short-term rainfall fluctuations proven to significantly affect both vegetation indices and buffalo dynamics (Dublin and Ogutu, Reference Dublin and Ogutu2015; Abraham et al., Reference Abraham, Hempson and Staver2019; see Chapter 7). Additionally, while it was long believed to be strongly philopatric, forming large aggregations remaining on separate home ranges and with few interchanges (male-biased dispersal; Estes, Reference Estes1991; reviewed in Chapter 6), according to collaring studies in Botswana, 5 of 75 (7 per cent) female buffalo showed long-distance movement, with distances from 120 km to over 200 km, and 5 of 32 (16 per cent) herd-switching. The latter is supported by a high mtDNA diversity among females within herds in Kruger Nation Park (KNP, South Africa). Consequently, the African buffalo shows high gene flow over evolutionary timescales, reflected by low genetic differentiations between populations within lineages (Simonsen et al., Reference Simonsen, Siegismung and Arctander1998; Van Hooft et al., Reference Van Hooft, Groen and Prins2002; Smitz et al., Reference Smitz, Berthouly and Cornélis2013; de Jager et al., Reference de Jager, Glanzmann and Möller2021) – in fact, the lowest among African mammals studied, as reviewed in Smitz et al. (Reference Smitz, Berthouly and Cornélis2013) and Lorenzen et al. (Reference Lorenzen, Heller and Siegismund2012).
During the Pleistocene, oscillations in the precipitations governing the physiography of Africa – the major vegetation zones being savannas and tropical forests (Moreau, Reference Simonsen, Siegismung and Arctander1963; Dupont and Agwu, Reference Dupont and Agwu1992; DeMenocal, Reference DeMenocal2004; Dupont, Reference Dupont2011; Lehmann et al., Reference Lehmann, Archibald and Hoffmann2011; Staver et al., Reference Staver, Archibald and Levin2011) – are believed to be the main drivers of population expansion in savanna species during cool and dry phases (interpluvials/glacial) and contraction during wet and warm phases (pluvials/interglacials). This is in agreement with the fact that congruent phylogeographical patterns across taxonomic groups and trophic levels have been observed, suggesting similar forces shaped species’ evolutionary histories (reviewed in Lorenzen et al., Reference Lorenzen, Heller and Siegismund2012). Repeated shifts of the two major vegetation zones facilitated the emergence and evolution of many bovid taxa (Vrba, Reference Vrba, Vrba, Denton, Partridge and Burckle1995; Bobe et al., Reference Bobe, Behrensmeyer and Chapman2002; Bobe and Behrensmeyer, Reference Bobe and Behrensmeyer2004). These considerable fluctuations have promoted divergence within and between the two buffalo lineages (WC versus ES clusters); the latter north-south structuration has been identified across multiple species associated with savanna ecosystems (Lorenzen et al., Reference Lorenzen, Heller and Siegismund2012). Periodic separation by an equatorial forest belt during moist pluvials could have acted as a barrier to gene flow (populations isolated in refugia), with secondary contacts during dry interpluvials (Arctander et al. Reference Arctander, Johansen and Coutellec-Vreto1999; Van Hooft et al., Reference Van Hooft, Groen and Prins2002; Lorenzen et al., Reference Lorenzen, Heller and Siegismund2012). The overlapping or suture zone between WC and ES buffalo clusters is proposed to be located in East Africa (Smitz et al., Reference Smitz, Berthouly and Cornélis2013), a region identified as a melting pot of long-diverged lineages across many taxa – for example, the kob, Kobus kob (Lorenzen et al., Reference Lorenzen, De Neergaard and Arctander2007, Reference Lorenzen, Heller and Siegismund2012). Despite the lack of contemporary barriers to gene flow (supported by the aforementioned clinal genetic structure at autosomal microsatellites), lineages appear conserved, with female gene flow estimated to be in the order of no more than five mitochondrial genomes per generation since divergence (Smitz et al., Reference Smitz, Berthouly and Cornélis2013).
Some inferred demographic changes shaping the pattern of divergence and distribution of the species could be dated and linked to historical climatic, environmental and/or anthropogenetic events. The most ancient identified expansion pre-dated the above-mentioned divergence between the WC and ES clusters, and started approximatively one million years ago to continue until ~500 kyr (de Jager et al., Reference de Jager, Glanzmann and Möller2021). This period was marked by a shift between arid and moist conditions toward less extreme cycles leading to the development of a more stable savanna environment, allowing for the expansion of the buffalo ancestor (see Chapter 2). The genetic divergence between the WC and ES clusters was dated to around 130–300 kyr, resulting from populations isolated in allopatry in savanna refugia (Van Hooft et al., Reference Van Hooft, Groen and Prins2002; Smitz et al., Reference Smitz, Berthouly and Cornélis2013). These particular core areas were characterized by long-standing savanna habitat enabling the continued survival of savanna-adapted taxa (Lorenzen et al., Reference Lorenzen, Heller and Siegismund2012). Because Pleistocene-dated fossils resemble buffalo of the present-day WC cluster, the ES cluster (or Cape buffalo) might have derived from a stock of savanna buffalo from WC (Gentry, Reference Gentry, Maglio and Cook1978; Kingdon, Reference Kingdon1982). Likewise, the forest dwarf buffalo (S. c. nanus – WC cluster) turned out to be an advanced form derived from savanna buffalo, rather than being the ancestor of all living African buffalo (Smitz et al., Reference Smitz, Berthouly and Cornélis2013; see Chapter 2). African buffalo refugia were purportedly proposed in present-day Uganda and Central African Republic, where present-day populations display the highest genetic diversities within the species (Smitz et al., Reference Smitz, Berthouly and Cornélis2013). Yet, both sampling size and species distribution coverage in West Central Africa have been limiting factors in all conducted studies, presumably linked to the difficulty of collecting material for DNA-based investigations from these regions. Further efforts are recommended to fill knowledge gaps, based on the use of a new generation of molecular markers made available by technological advances in the field of genome sequencing.
The aforementioned refugia played an important role in the dispersal of the lineages. A first westward expansion event of the WC cluster after divergence occurred in the late to middle Pleistocene (~100 kyr) along two routes, into the forest belt and the Western Sahel region, hence adapting morphologically to colonize new habitats (Smitz et al., Reference Smitz, Berthouly and Cornélis2013). The latter can be associated with the shift from persistent rainforest in both dry and wet periods before ~220 kyr to its reduction and replacement by savanna after ~220 kyr (Dupont and Agwu, Reference Dupont and Agwu1992; Dupont et al., Reference Dupont, Jahns and Marret2000; DeMenocal, Reference DeMenocal2004). Unlike the WC cluster, the southward expansion of the ES cluster occurred after a core was retained in Eastern Africa, probably unable to colonize this part of the continent due to extremely arid conditions between 135 and 90 kyr. A demographic decline in the ES cluster was even identified around 100 kyr, proposed to be a consequence of a series of mega-droughts registered in East Africa around that time, to which the African buffalo is especially sensitive (de Jager et al., Reference de Jager, Glanzmann and Möller2021). After aridity decreased, reaching near modern conditions around 60 kyr (Cohen et al., Reference Cohen, Stone and Beuning2007; Scholz et al., Reference Scholz, Johnson and Cohen2007), the development of large savanna-type grasslands allowed for an expansion of the ES cluster around 50 kyr (Van Hooft et al., Reference Van Hooft, Groen and Prins2002; Smitz et al., Reference Smitz, Berthouly and Cornélis2013) or 80 kyr (Heller et al., Reference Heller, Brüniche-Olsen and Siegismund2012; de Jager et al., Reference de Jager, Glanzmann and Möller2021). Another, non-exclusive hypothesis is that the expansion could have followed the extinction of the giant long-horned buffalo (Peloveris antiquus), which dominated savannas until the late Pleistocene, as supported by fossil data (Kingdon, Reference Kingdon1982; Klein, Reference Klein, Martin and Klein1995; Van Hooft et al., Reference Van Hooft, Groen and Prins2002; see Chapter 2). This expansion was concurrent with the expansion of humans between 80 and 10 kyr (Heller et al., Reference Heller, Brüniche-Olsen and Siegismund2012). It therefore refutes an adverse ecological effect of Palaeolithic humans (Heller et al., Reference Heller, Brüniche-Olsen and Siegismund2012). Finally, it is worth pointing out that the finding of Syncerus-like fossil records in Southern Africa pre-dating this expansion (Porat et al., Reference Porat, Chazan and Grün2010) might indicate multiple colonization–extinction events in the region, following habitat suitability (Smitz et al., Reference Smitz, Berthouly and Cornélis2013). Local loss of populations in Southern Africa and subsequent recolonization from an East core was also suggested for the hartebeest Alcelaphus buselaphus, the topi Damaliscus lunatus and the giraffe Giraffa camelopardalis (Arctander et al., Reference Arctander, Johansen and Coutellec-Vreto1999; Pitra et al., Reference Pitra, Hansen and Lieckfeldt2002; Brown et al., Reference Brown, Brenneman and Koepfli2007).
Following this expansion phase, a strong signal of population decline was identified within the ES cluster, in the order of 75–98 per cent (Heller et al., Reference Heller, Lorenzen and Okello2008, Reference Heller, Brüniche-Olsen and Siegismund2012). This major decline was not detected in the studies of Van Hooft et al. (Reference Van Hooft, Groen and Prins2002) and Smitz et al. (Reference Smitz, Berthouly and Cornélis2013), although discrepant demographic signals can be obtained from different types of molecular markers and databases. This major bottleneck occurred around ~5000 years ago (Heller et al., Reference Heller, Lorenzen and Okello2008, Reference Heller, Brüniche-Olsen and Siegismund2012). The mid-Holocene aridification, marked by a pronounced transition from warm and wet (the Holocene Climatic Optimum – DeMenocal et al., Reference DeMenocal, Ortiz and Guilderson2000) to drier conditions around 4500 years ago (Marchant and Hooghiemstra, Reference Marchant and Hooghiemstra2004; Burroughs, Reference Burroughs2005; Kiage and Liu, Reference Kiage and Liu2006), was identified as a possible driver of the effective population size decline. In addition to the climate-mediated decline hypothesis, the explosive growth in human population size and their domestic bovines (the Neolithic revolution – Finlay et al., Reference Finlay, Gaillard and Vahidi2007; Scheinfeldt et al., Reference Scheinfeldt, Soi and Tishkoff2010) and correspondingly rapid decline in buffalo populations from 5 kyr onwards, could represent an alternative explanation (Heller et al., Reference Heller, Brüniche-Olsen and Siegismund2012). Together, they could have driven humans, domesticated cattle and large savanna mammals into closer contact around remaining water sources, leading to ecological competition and possible spill-over of exotic diseases from cattle to buffalo. This two-phased dynamic (expansion/decline) was also observed in other drought-intolerant species, such as the savanna elephant Loxondonta africana and baboon Papio cynocephalus (Storz et al., Reference Storz, Beaumont and Alberts2002; Okello et al., Reference Okello, Wittemyer and Rasmussen2008), indicating a community-wide collapse.
Various studies indicate that the African buffalo from Southern Africa have relatively high frequencies of deleterious alleles throughout their genome, which negatively affect male body condition and disease resistance (Van Hooft et al., Reference Van Hooft, Greyling and Getz2014, Reference Van Hooft, Dougherty and Getz2018, Reference Van Hooft, Getz and Greyling2019, Reference Van Hooft, Getz and Greyling2021). These high frequencies are attributed to an underlying sex-ratio meiotic gene-drive system. Meiotic drivers are selfish genetic elements that, by distorting meiosis, favour transmission of the chromosome on which they reside. In the case of sex chromosomes, this results in distorted primary sex ratios, as observed in KNP and HiP (Van Hooft et al., Reference Van Hooft, Prins and Getz2010, Reference Van Hooft, Getz and Greyling2019). High frequencies of deleterious alleles indicate that environmental stressors such as drought and diseases have been consistently acting as selective agents for long periods of time. Despite this, most populations of African buffalo seem to have been large in the recent evolutionary past and to be stable after their recovery from the rinderpest pandemic of 1889–1895. This seems to support the view, advocated by some population geneticists, that deleterious alleles and genetic diversity in general play a smaller role in ecology, at least with respect to demographics, than one might expect (Agrawal and Whitlock, Reference Agrawal and Whitlock2012; Teixeira and Huber, Reference Teixeira and Huber2021).
Note that overall, less is known for the WC cluster because available studies are limited by the sampling size and geographical coverage for this region, as well as by the type of DNA marker investigated, limiting the possible inferences (Van Hooft et al., Reference Van Hooft, Groen and Prins2002; Smitz et al., Reference Smitz, Berthouly and Cornélis2013). To our knowledge, two ongoing studies involving the investigation of genome-wide single nucleotide polymorphism (SNP) data and whole genomes (WGS) undertaken by the research teams of L. Morrison (University of Edinburgh) and of J. Michaux (University of Liège) might uncover some additional events which shaped the evolutionary history of the WC cluster.
Population Genetic Structure at Local Scale and Linked to Recent Events
The African buffalo has suffered important population losses during the last century, impacting all of the subspecies mentioned above. Of the more than 3 million buffalo that roamed the continent in the nineteenth century (Lessard et al., Reference Lessard, L’Eplattenier and Norval1990), only around one million presently survive (Chapter 4).
Habitat loss and poaching are the main challenges currently threatening the species. Habitat loss can be due to anthropogenic factors (Alroy, Reference Abraham, Hempson and Staver2001; Godfrey and Jungers, Reference Godfrey and Jungers2003; Surovel et al., Reference Surovell, Waguespack and Brantingham2005) or to climatic changes (Meijaard, Reference Meijaard2003; Barnosky et al., Reference Barnosky, Koch and Feranec2004; Lovett et al., Reference Lovett, Midgley and Barnard2005; Vanacker et al., Reference Vanacker, Linderman and Lupo2005), as for example the increasing drought observed in Africa since the 1990s (rain is the ecologically most important climate variable in most of Africa). The African buffalo, a species highly sensitive to drought (Ogutu et al., Reference Ogutu, Piepho and Dublin2008), exhibits important climate-mediated population decline as demonstrated by a decrease in the Masai Mara population from 10,000 to 2400 individuals during the severe drought of 1993–1994 (East, Reference East1999). This last factor was associated with other drivers like enhanced encroachments of pastoralists/cattle and commercial farms and changes in governance systems, which further aggravated the situation (Chapter 12).
Fragmentation of the natural habitat into small patches also endangers the populations by increasing genetic drift, resulting in loss of genetic diversity and consequently leading to a reduction in the evolutionary potential of the species (Frankham et al., Reference Frankham, Lees and Montgomery1999; Hedrick, Reference Hedrick2005). For example, around 75 per cent of all buffalo (estimated to be around 900,000 animals) are currently located in protected areas (i.e. national parks (NPs) and game reserves; East, Reference East1999), with many populations completely isolated each from another (Chapter 4). These reduced population sizes due to human-induced population fragmentation have a strong impact on local genetic diversity. In Kenya and Uganda, a significant correlation between park area and microsatellite heterozygosity (fraction of individuals with two different alleles per microsatellite) was observed, with populations in small parks (<400 km2) having a genetic diversity reduced by ~5 per cent compared to the population of the Masai Mara–Serengeti ecosystem (Heller et al., Reference Heller, Okello and Siegismund2010). This amount of reduction in genetic diversity was also observed among the buffalo from the Ngorongoro Crater, Tanzania (Ernest et al. Reference Ernest, Haanes and Bitanyi2012). In South Africa, genome-wide diversity in the populations from HiP (~4500 buffalo) and Addo NP (~800 buffalo) is 19 per cent and 31 per cent smaller, respectively, in comparison to the KNP population (~40,900 buffalo) due to historical population bottlenecks (de Jager et al., Reference de Jager, Glanzmann and Möller2021). Other small isolated populations with reduced genetic diversity are those in Arusha NP (Kenya, ~1800 buffalo in the early 1970s; Ernest et al., Reference Ernest, Haanes and Bitanyi2012) and Campo-Ma’an (Cameroon, <100 buffalo; Bekhuis et al., Reference Bekhuis, De Jong and Prins2008), which show ~15 per cent reduction in mtDNA diversity compared to nearby populations (Smitz et al., Reference Smitz, Berthouly and Cornélis2013). It is therefore safe to assume that genetic drift affects population in smaller conservancies more rapidly than in larger ones. It is also expected that this genetic erosion will become significantly more progressive in the near future (Heller et al., Reference Heller, Okello and Siegismund2010). Suppression or restriction of gene flow by confinement into small areas could also have an ethological impact, disturbing the behaviour of natural dispersion in response to seasonal variations in food availability (Sinclair, Reference Sinclair1977; Halley et al., Reference Halley, Vandewalle, Mari and Taolo2002; Ryan et al., Reference Ryan, Knechtel and Getz2006; Heller et al., Reference Heller, Okello and Siegismund2010).
The introduction of non-native species, such as domestic cattle, besides generating direct competition for natural resources, also poses severe problems due to the introduction of pathogens. Indeed, domestic cattle and African buffalo are related closely enough to cause considerable challenges in terms of disease transmission. It was notably the case of the rinderpest morbillivirus introduced in 1889 by a colonial military expedition to Ethiopia (Branagan and Hammond, Reference Branagan and Hammond1965; Sinclair, Reference Sinclair1977; Prins, Reference Prins1996). The African buffalo has probably been one of the African species that has suffered most from this disease (extreme regional reductions in population density, paired to many local extinctions; Wenink et al., Reference Wenink, Groen and Roelke-Parke1998), with the most severe collapse occurring in the 1890s when mortality rates estimated between 90 per cent and 95 per cent were registered over the continent (Mack, Reference Mack1970; Sinclair, Reference Sinclair1977; Plowright, Reference Plowright, Edwards and McDonnell1982; Prins and Van der Jeugd, Reference Prins and van der Jeugd1993; Shigesada and Kawasaki, Reference Shigesada and Kawasaki1997; O’Ryan et al., Reference O’Ryan, Harley and Bruford1998; Winterbach, Reference Winterbach1998).
Some studies investigated the impact of rinderpest epidemics on the genetic diversity of the African buffalo. Results contrasted between no reported genetic signature of a recent bottleneck (Simonsen et al., Reference Simonsen, Siegismung and Arctander1998; Van Hooft et al., Reference Van Hooft, Groen and Prins2000; Heller et al., Reference Heller, Lorenzen and Okello2008) to the observation of a population decline caused by the rinderpest epidemic (Heller et al., Reference Heller, Brüniche-Olsen and Siegismund2012; de Jager et al., Reference de Jager, Glanzmann and Möller2021). Nevertheless, all studies still reported high genetic diversities (O’Ryan et al., Reference O’Ryan, Harley and Bruford1998; Simonsen et al., Reference Simonsen, Siegismung and Arctander1998; Wenink et al., Reference Wenink, Groen and Roelke-Parke1998; Van Hooft et al., Reference Van Hooft, Groen and Prins2000, Reference Van Hooft, Groen and Prins2002; Heller et al., Reference Heller, Lorenzen and Okello2008, Reference Heller, Brüniche-Olsen and Siegismund2012; Smitz et al., Reference Smitz, Berthouly and Cornélis2013; Smitz et al., Reference Smitz, Cornélis and Chardonnet2014a; de Jager et al., Reference de Jager, Glanzmann and Möller2021). Even though the continent-wide pandemic reportedly caused important buffalo mortalities (with death rates in some localities possibly as high as 90 per cent; Lessard et al., Reference Lessard, L’Eplattenier and Norval1990; Estes, Reference Estes1991; Prins, Reference Prins1996; O’Ryan et al., Reference O’Ryan, Harley and Bruford1998), the absence of a pronounced effect on the genetic diversity might result from a possible overestimation of the severity of the pandemic in terms of population decline, but also from a rapid population regrowth combined with high interpopulation gene flow, reintroducing rare alleles and distorting the genetic signal of bottleneck (Van Hooft et al., Reference Van Hooft, Groen and Prins2000; Heller et al., Reference Heller, Lorenzen and Okello2008). This is supported by the observation that survivors recolonized their range, being so productive that by 1920 the species was again numerous (Sinclair, Reference Sinclair1977; Estes, Reference Estes1991). For example, in the KNP, area survival estimates were off by at least a factor of 10, considering the high number of mitochondrial and Y-chromosomal haplotypes observed in the present-day population.
High genome-wide nucleotide diversity in KNP is indicative of a large long-term effective population size of ~48,000 individuals (de Jager et al., Reference de Jager, Glanzmann and Möller2021). Because within-population nucleotide diversity is largely determined by the total size of a metapopulation, this effective population size is probably indicative for the subspecies as whole (Strobeck, Reference Strobeck1987). The aforementioned linear relationship between genetic and geographic distance (Figure 3.1) indicates that this effective population size varies little between the different savanna-dwelling subspecies. However, effective population size is probably considerably smaller for the small S. c. nanus subspecies, considering the relative isolation and small sizes of the forest-dwelling populations as indicated by the relatively large genetic distances observed with microsatellites.
Conclusion
The evolutionary history of the African buffalo began a long time ago, between one million and 500,000 years ago. It started with an expansion throughout sub-Saharan Africa, probably during cool and dry phases (interpluvials/glacial) as these periods favoured the development of more constant savanna environments. Later, around 130–300 kyr, population isolations in savanna refugia led to an allopatric differentiation and to the appearance of two main genetic lineages (the WC and EC clusters). These lineages spread again from Central African refugia, in sub-Saharan Africa during the late to middle Pleistocene along different routes: into the forest belt and the Western Sahel regions, for the WC cluster, and in the south of the continent for the EC one. Following this expansion phase, a strong signal of population decline was identified within the ES cluster around ~5000 years ago. This decline could be linked to the mid-Holocene aridification of Africa, but also to the explosive growth in the population sizes of humans and their domestic bovines (the Neolithic revolution), which also happened during this period. In more recent times, during the last century, the African buffalo also suffered important population losses. Habitat loss and poaching are the main challenges currently threatening the species. Habitat loss can mainly be due to anthropogenic factors or, to a lesser degree, climatic changes. Other aspects like the introduction of non-native species, such as domestic cattle, besides generating direct competition for natural resources, also had a deep impact on the Africa buffalo’s survival due to the introduction of pathogens.
Concerning the taxonomic aspect, genetic studies tend to propose either two (S. c. caffer of the East and Southern African savanna and S. c. nanus, in Western and Central Africa), or three (S. c. caffer of the East and Southern African savannas; S. c. nanus of the West and Central African rain forests; and S. c. umarii in the savanna buffalo of the West and Central African savannas) subspecies. However, irrespective of subspecies designation, which appears quite subjective, the Eastern and Southern populations, the West and Central African forest buffalo and the West and Central African savanna buffalo should be recognized as three separate Conservation Units. Indeed, the global conservation status of the West Central African forest buffalo is not as good as that for the West Central African savanna buffalo (Chapter 4). Its conservation context is also quite distinct from that of the West Central African savanna buffalo. A particular conservation status for the forest buffalo group is therefore needed.
From a genetic point of view, the main challenges for the conservation and management of the African buffalo are the development of new genetic markers, such as the study of whole-genome sequences, which will give an even more precise information concerning the evolutionary history of the African buffalo and the relationships among the different conservation units. The comparison of neutral as well as selective genetic traits will also help to better understand the impact of artificial hybridization among different African buffalo morphotypes, which are developed in some areas to obtain particular hunting trophies (in the frame of game farming activities). In a more general context, another important challenge will be to promote the integration of genetic studies in conservation practices (i.e. important to retain high genetic diversity and gene flow for long-term conservation – and better consider the impact of habitat fragmentation and land use and major drought events).
Introduction
In this chapter, we provide an update on the distribution and abundance of the African buffalo at the scale of the entire African continent. For this purpose, we conducted a literature search to uncover published information. We also carried out an extensive survey of national and international agencies and field experts in the 37 countries that are within the buffalo’s distribution range.
We collected abundance data from 163 protected areas or protected area complexes for the period 2001–2021. These data are mainly based on aerial counts using standardized methods, and occasionally on estimates provided by experts. We also obtained information on the presence of buffalo in 711 localities (inside and outside protected areas) for the period 2001–2021. These data and metadata were compiled in a database that is available upon request (Cornélis et al., Reference Cornélis, Melletti, Renaud, Fonteyn, Bonhotal, Prins, Chardonnet and Caron2023).
We present the distribution and abundance of each of the four subspecies of African buffalo. We are naturally aware that the validity of the ‘subspecies’ concept is under debate, and we refer to Chapters 2 and 14 for a discussion about the number of subspecies and their status. For the sake of consistency with earlier studies on buffalo distribution (East, Reference Dupuy1998; Cornélis et al., Reference Cornélis, Melletti, Korte, Melletti and Burton2014), our results are presented in accordance with the latest IUCN subspecies range (IUCN SSC Antelope Specialist Group, 2019). Therefore, maps of this chapter reproduce the geographical boundaries of the four subspecies published by the IUCN Red List of Threatened Species (IUCN SSC Antelope Specialist Group, 2019). Although clearly delineated on paper, the boundaries between subspecies’ distribution ranges are in fact blurry on the ground. In case of inconsistency or doubt about the assignment of a population to a ‘subspecies’ (especially in transitional areas), we explicitly acknowledge this in the text.
Historical Distribution
Endemic to the African continent, the buffalo is one of the most successful mammals in terms of geographical distribution, abundance and biomass. Its range covers almost all natural ecosystems south of the Sahara. It mainly inhabits savannas with high herbaceous biomass, but also occupies dry shrubland as well as grassy clearings in dense tropical rainforests, and can live at altitudes above 2500 m, such as in Aberdare National Park in Kenya. The African buffalo penetrates arid biomes where surface water is permanently available. Overlaying the African buffalo’s current continental range with mean annual rainfall (Figure 4.1) shows that 95 per cent of the buffalo’s range comprises areas with more than 450 mm of rainfall (min: 150 mm; max: 4000 mm).

Figure 4.1 African buffalo distribution range in relation to average rainfall for 1970–2000.
African buffalo formerly occupied the entire savanna zone stretching between Senegal, Gambia and Guinea and Ethiopia and Eritrea, and from there south to the Cape of Good Hope, with the exception of drylands. African buffalo did not colonize islands such as Zanzibar or Mafia, although they did colonize Bioko Island (Equatorial Guinea), from where they were extirpated sometime between 1860 and 1910 (Butynski et al., Reference Butynski, Schaaf and Hearn1997).
There is no palaeontological evidence of the presence of the African buffalo in North Africa or the Nile Valley to the north of Khartoum (Prins and Sinclair, Reference Prins, Sinclair, Knight, Kingdon and Hoffmann2013). In North Africa, the aurochs (Bos primigenius; wild ancestor of domestic cattle) occupied a similar niche (Gautier, Reference Funston, Henschel and Petracca1988), perhaps preventing the buffalo’s spread to the north. Buffalo could have expanded their range in eastern and southern Africa during the last ice age due to the extinction of possible competitors, such as Pelorovis antiquus and Elephas reckii (for more details on evolution see Chapter 2; Klein, Reference Klein1988, Reference Klein1994; Prins, Reference Prins1996).
Present Distribution
In areas of high human densities, people and their activities caused large discontinuities to arise in the historical distribution of African buffalo (Figure 4.2). Although according to our estimates its population remains above 500,000 individuals, and has been above that level since at least the last human generation (East, Reference Dupuy1998; Cornélis et al., Reference Cornélis, Melletti, Korte, Melletti and Burton2014), the species’ distribution range has been severely reduced since the nineteenth century. As there were no reliable estimates of its total population prior to the assessment undertaken by East (Reference Dupuy1998), we cannot determine whether the current population is smaller than that which existed prior to the Great Rinderpest epidemic of the 1880s (e.g. Prins, Reference Prins1996; Prins and Sinclair, Reference Prins, Sinclair, Knight, Kingdon and Hoffmann2013). The shrinkage of the species’ range was the result of the combined effects of anthropogenic impacts such as rangeland conversion, poaching, disease outbreaks and political unrest, and climatic events such as droughts. At present, most savanna populations (i.e. the three subspecies except S. c. nanus) are confined to protected areas (including trophy hunting areas).
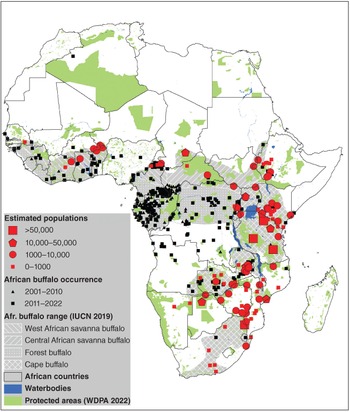
Figure 4.2 Continental distribution and abundance of African buffalo. The two classes of occurrence (2001–2010 and 2011–2022) refer only to the date of the source and do not signify a change in status between classes. Note that in certain other chapters of this book, the West African savanna buffalo and the Central African savanna buffalo are considered together and are referred to as the ‘Northern savanna buffalo’.
Since the nineteenth century, the expansion of livestock has gradually generated direct competition for space and resources and has led to large and destructive epidemics in African buffalo populations. Exotic rinderpest was historically the most devastating disease for buffalo populations throughout Africa, leading to extreme reductions in population densities and local extinctions. The most severe population collapse occurred in the 1890s, with mortality rates estimated at 90–95 per cent across the continent (Sinclair, Reference Sinclair1977; Prins and van der Jeugd, Reference Prins and Jeugd1993; Winterbach, Reference Winterbach1998). This was followed by other episodes throughout the twentieth century. The World Organization for Animal Health (WOAH, formerly OIE) declared rinderpest eradicated in Africa in 2011 (last case reported in 2001). Rinderpest was the first animal disease to have been globally eliminated, and the second disease after human smallpox to have been globally eradicated. Both diseases are caused by viruses. During the twentieth century, efforts to limit the transmission to cattle of several pathogens, such as foot and mouth disease (FMD) and trypanosomiasis (Taylor and Martin, Reference Taylor and Martin1987), also actively reduced the geographic distribution of buffalo in several countries due to large-scale culling operations and the erection of veterinary fences.
Outright competition for range use and overexploitation by all sorts of poachers including local pastoralists also were important drivers behind the degradation of the buffalo’s status (e.g. Prins, Reference Prins1992; Prins and De Jong, Reference Prins, De Jong, Kiffner, Bond and Lee2022; Scholte et al., Reference Scholte, Pays and Adam2022).
Recent climate fluctuations, such as the droughts that affected Sahelian and Sudanese regions at the end of the 1960s and southern Africa in 1992 (Dunham, Reference Douglas-Hamilton, Froment, Doungoube and Root1994; Mills et al., Reference Mills, Biggs and Whyte1995; Chapter 8), have also strongly affected buffalo populations over the past few decades. Finally, yet importantly, armed conflicts, the feeding of armies and labourers during peacetime, the traffic of weapons and the bushmeat trade have strongly contributed to the reduction of buffalo populations in some areas (e.g. Prins et al., in review).
West African Savanna Buffalo (S. c. brachyceros)
In the 1970s and 1980s, this subspecies still locally occurred in Sahelo-Sudanian savannas and gallery forests, including those found in south-eastern Senegal, northern Côte d’Ivoire, southern Burkina Faso, Ghana, northern Benin, the extreme south of Niger, Nigeria (very locally), the northern part of Cameroon and Central African Republic (west of Chari River) (East, Reference Dupuy1998). It is worth noting that the West African savanna buffalo (Figure 4.3) was (and still is) therefore also found in Central Africa, which underlines the inconsistency of this appellation (Figures 4.4 and 4.6).

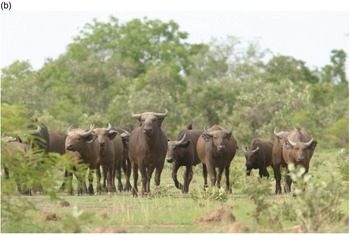
Figure 4.3 West African savanna buffalo in W National Park, Niger.
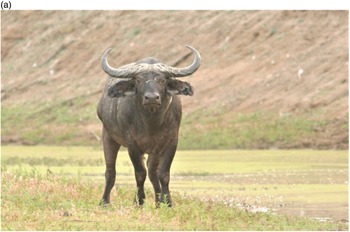

Figure 4.5 Central African savanna buffalo in Zakouma National Park, Chad.
Presently, most known populations remain in five main strongholds. Two of these are complexes hosting national parks (NP) and neighbouring trophy hunting areas: (1) W–Arly–Pendjari NPs (WAP) in Burkina Faso–Benin–Niger; and (2) Bouba N’djidda–Bénoué–Faro NPs in Cameroon. The remaining three strongholds are single NPs: (3) Niokolo-Koba NP in Senegal; (4) Comoé NP in Côte d’Ivoire; and (5) Mole NP in Ghana. In the other protected areas of the above-mentioned countries, and in Nigeria, Togo and Sierra Leone, the presence of buffalo is limited to a few scattered residual populations. At present, the populations in the remaining strongholds are isolated from each other and the distribution of the West African savanna buffalo has shrunk overall. A positive finding emerging from our investigation is that the buffalo populations inside four of these five strongholds are, when compared with 2013 figures, either constant (Niokolo-Koba NP) or increasing (Comoé NP, WAP complex, Mole NP). On the downside, the populations in Northern Cameroon appear to be decreasing.
Central African Savanna Buffalo (S. c. aequinoctialis)
This subspecies still locally populates Central African countries within the Sahelo-Sudanian savannas and gallery forests: southeast Chad, northern Central African Republic (east of Chari River), northern Democratic Republic of Congo (DRC), south-east Sudan and western Ethiopia (Figures 4.5 and 4.6). The subspecies is now extinct in Eritrea. Most presently known populations remain in two main strongholds: Zakouma NP in Chad and Garamba NP in DRC. In Ethiopia, the decline of several populations has been offset by the recent discovery of several populations outside the known range (see Ethiopia section below).
Forest Buffalo (S. c. nanus)
The distribution range of the forest buffalo comprises two separate regions in West and Central Africa (Figures 4.4, 4.6 and 4.7). In West Africa, fragmented and isolated populations persist in the relict rainforest belt, while the population’s stronghold is located in the Central African countries of the Congo Basin (Cornélis et al., Reference Cornélis, Melletti, Korte, Melletti and Burton2014; IUCN SSC Antelope Specialist Group, 2019). In the Congo Basin, forest buffalo occur in the south of the Central African Republic, western Uganda, Democratic Republic of Congo, Republic of Congo, southern Cameroon, Equatorial Guinea and Gabon.


Figure 4.7 Forest buffalo in Odzala National Park, The Republic of Congo.
In West Africa, the subspecies persists in Benin, Ghana, Guinea, Guinea-Bissau, Côte d’Ivoire, Liberia, Nigeria and Sierra Leone (see below). Forest buffalo are highly associated with forest clearings and riverine forests (Prins and Reitsma, Reference Prins and Reitsma1989; Blake, Reference Blake2002; Melletti et al., Reference Melletti, Penteriani and Boitani2007, Reference Melletti, Penteriani, Mirabile and Boitani2008; Bekhuis et al., Reference Bekhuis, De Jong and Prins2008; Korte, Reference Bekhuis, de Jong and Prins2008a). In several poorly explored areas, gaps remain in the scientific knowledge of the distribution and status of forest buffalo.
Contrary to the savanna buffalo, recent estimates of the population size of forest buffalo are available only for a few areas in the Congo Basin and their accuracy is low. Indeed, unlike aerial surveys carried out in savanna areas, surveys methods in forest environment are currently unable to provide reliable population estimates. Such estimates may become available for a larger number of sites once more appropriate techniques are implemented, such as distance sampling via camera traps, capture–mark–recapture methods using genetic fingerprinting, and methods to formally capture information from local experts (e.g. indigenous people and local communities living in the rainforests).
Cape Buffalo (S. c. caffer)
The Cape buffalo’s range encompasses East and Southern Africa and covers 17 countries (Figures 4.6, 4.8 and 4.9). In East Africa, Cape buffalo populations occur in southwestern Ethiopia, southern Somalia, Uganda, Kenya, Tanzania, Rwanda and Burundi. In southern Africa, this subspecies is distributed in Mozambique, Malawi, Zambia, south-west Angola, north-east Namibia, northern Botswana, Zimbabwe and South Africa. The current population in Eswatini (formerly known as Swaziland) was reintroduced after extirpation.

(a) Cape buffalo in Ngorongoro Conservation Area, Tanzania.
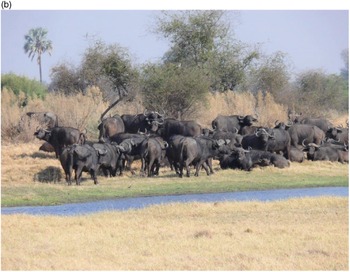
(b) Cape buffalo in Okavango Delta (Botswana).
Abundance Per Country
West Africa
Burkina Faso
West African savanna buffalo formerly occurred widely in the open woodlands of the Niger basin and southern districts (Sidney, Reference Sidney1965; East, Reference Dupuy1998). Starting from the 1980s, the population has been restricted to the southernmost areas of the country. At the national level, the buffalo population comprises around 6000 individuals. Their presence is recorded in six different localities, all conservation areas (national park, protected forest, game ranch or trophy hunting area). The largest populations are located in the eastern conservation areas: Arly complex with about 4950 individuals (0.5 ind./km²) and W Burkina Faso complex with about 300 animals (0.8 ind./km²) (Ouindeyama, Reference Ouindeyama, Chevillot and Akpona2021). The presence of buffalo is reported in central and western conservation areas (Nazinga Game Ranch, Bougouriba, Comoé-Lareba and Tuy Mouhoun areas) but in very low densities and isolated populations (Dahourou and Belemsobgo, Reference Cumming2020). In Nazinga Game Ranch, the total population is around 150 individuals (PAPSA, 2018).
After a period of growth between 2003 and 2015 (from ~4800 to ~8900 individuals; Bouché et al., 2003, Reference Bouché, Nzapa Mbeti Mange and Tankalet2015), buffalo populations of eastern conservation areas recently faced a strong population decline (~5300 individuals; Ouindeyama et al., Reference Ouindeyama, Chevillot and Akpona2021). Due to the severe worsening of the security situation, the protected areas are facing an increase in threats of banditry, adding new forms of violent interactions with protected area management teams.
Côte d’Ivoire
West African savanna buffalo formally occurred throughout the northern region (Sidney, Reference Sidney1965; East, Reference Dupuy1998), but their populations have collapsed and are now isolated in a few protected areas. The main population is located in Comoé NP with an estimated 1450 individuals (0.1 ind./km²; OIPR, 2019), which has increased slightly since 2010 (then about 900 individuals; N’Goran et al., Reference N’Goran, Maho and Kouakou2010) and 2016 (1200 individuals; Bouché, Reference Bouché, Frederick and Kohi2016). The presence of buffalo has been recorded in Lamto and Marahoué NPs, but their numbers were not reported and are thought to be very low. Interestingly, a population of about 300 individuals was reported in N’Zi River Lodge Voluntary Nature Reserve near Bouaké (2.1 ind./km²) and is known to be growing (Louis and Karl Diakité, personal communication, 2021).
The few observations of the forest buffalo subspecies are reported from the residual blocks of forest in the south of the country. This holds especially for Tai NP, where transect counts gave an estimated population size of ~500 individuals (0.09 ind./km²) in 1999–2004, with lower estimates based on transect dung counts of ~200 individuals (Hoppe-Dominik et al., Reference Holubová2011). In striking contrast, recent detailed surveys only reported indirect signs, suggesting a collapse of the buffalo population (Tiedoue et al., Reference Tiedoue Manouhin, Kone Sanga, Diarrassouba and Tondossama2019, Reference Tiedoue Manouhin, Diarrassouba and Tondossama2020).
Between 2019 and 2021, a wildlife survey on foot was conducted throughout Côte d’Ivoire (ONFI, 2021). The data from this survey for African buffalo came mainly from indirect observations (tracks and dung) for which the risk of confusion with livestock was considered high (Gilles Moynot, ONFI, personal communication), and therefore are not included here.
Benin
West African savanna buffalo ranged in the past throughout the northern region (East, Reference Dupuy1998), but populations are now restricted to Pendjari and W-Benin complexes (both complexes include the eponymous NPs and surrounding trophy hunting areas). The Pendjari complex has an estimated population of about 7200 individuals (1.2 ind./km²), while in the W-complex some 1500 buffalo were counted (0.2 ind./km²; Ouindeyama et al., Reference Ouindeyama, Chevillot and Akpona2021). The three main aerial surveys conducted over the last two decades in northern Benin show that buffalo populations have doubled since the early 2000s (2003: ~4600 individuals; 2015: ~8200 individuals; 2021: ~8650 individuals; Bouché et al., 2003, Reference Bouché, Nzapa Mbeti Mange and Tankalet2015; Ouindeyama et al., Reference Ouindeyama, Chevillot and Akpona2021).
Records of forest buffalo mainly refer to old observations located in the centre and southern sectors of Benin (PAPFCA, 2007; Sinsin et al., Reference Sinsin, Kampmann, Thiombiano and Konaté2010). The last observations of forest buffalo were reported during a ground survey carried out in the Forest of Agoua (Central Benin) in 2013 in which about 100 individuals scattered over 12 herds were seen (Natta et al., Reference Natta, Nago and Keke2014). Further investigation is needed because buffalo are regularly observed in several spots in the southern and central parts of the country (Félicien Amakpe, personal communication).
Gambia
No recent information was received from this country. To our knowledge, the West Africa savanna buffalo subspecies is now extinct in Gambia (Jallow et al., Reference Jallow, Touray, Jallow, Chardonnet and Chardonnet2004).
Ghana
Buffalo formerly occurred throughout Ghana, with the West African savanna buffalo in the northern and eastern savannas, and the forest buffalo in the southwestern forests (Sidney, Reference Sidney1965; East, Reference Dupuy1998). The species is now restricted to a few protected areas.
The major surviving population of the savanna buffalo occurs in Mole NP with an estimated 1400 animals (0.3 ind./km²; Hauptfleisch and Brown, Reference Grossmann, Lopes Pereira and Chambal2019), and an increasing trend during the last decade (from about 700 individuals: Bouché, Reference Bouché2006). Small populations persist in the following savanna protected areas: Bui National NP (~60; 0.03 ind./km²), Yerada–Kenikeni Forest Reserve (~30; 0.03 ind./km²), Kyabobo NP (~50; 0.1 ind./km²), Digya NP (~120; 0.03 ind./km²) and Bomfobiri Wildlife Sanctuary (~30; 0.6 ind./km²) (David Kpelle, Ghana Forestry Commission, personal communication). Some individuals also have been spotted in the Kogyae Strict Nature Reserve (Danquah et al., Reference Dandena and Dinkisa2015) and Kalakpa Reserve (Afriyie et al., Reference Afriyie, Asare, Danquah and Pavla2021).
The status of the forest buffalo is unclear. Its presence was reported in Subri River Forest Reserve in 2011 (Buzzard and Parker, Reference Buzzard and Parker2012), but more recent information does not exist.
Guinea
African buffalo once occurred widely, with the West African savanna buffalo in the north intergrading to the forest buffalo in the south-west and south-east. It has been eliminated in most of its former range by overhunting and habitat destruction (East, Reference Dupuy1998) and remains in pockets of relict populations spread throughout the country. The savanna buffalo is known to be present in Haut Niger NP (Nefzi, Reference Nefzi2020) and Moyen-Bafing NP (Wild Chimpanzee Foundation, 2021); however, without numerical information. Forest buffalo is present in Ziama Biosphere Reserve, the largest primary rain forest of the country, next to Liberia (Nefzi, Reference Nefzi2020). It has also been observed recently in Tokounou, Tiro and Nialia subprefectures (Catherine André, personal communication), but no numerical estimates are available. However, we suspect that both savanna and forest subspecies may be present elsewhere in the country.
Guinea-Bissau
Intermediate forms between the West African savanna buffalo and the forest buffalo formerly occurred widely in the forest–savanna mosaic of Guinea-Bissau (East, Reference Dupuy1998). The species still occurs widely in the south and is reasonably common in some areas, for example Cufada Lagoons Natural Park, Cantanhez Forest (da Silva et al., Reference Danquah and Owusu2021) as well as Boe Region (Coppens, Reference Cockar2015), but no numerical estimates are available. No information was found from the northern part of the country.
Liberia
Only forest buffalo are known to occur in Liberia. In this country, the African buffalo was reported to occur sparsely in the 1960s (Sidney, Reference Sidney1965). A national survey carried out in 1989/1990 recorded the presence of the species in poorly accessible and high-altitude forests of the south-east and north-west (Anstey, Reference Anstey1991 cited by East, Reference Dupuy1998). The contraction of its distribution range in recent decades appears to be due to civil war, unrest and widespread poaching. We have no recent information on its status, except observations by camera traps in the Grebo-Krahn NP forest in 2020 (Wild Chimpanzee Foundation; www.youtube.com/watch?v=QtYUSk53p2Q).
Niger
In the early twentieth century, West African savanna buffalo occurred in the south-western tip of Niger (Niger River basin and along parts of the Nigeria border; East, Reference Dupuy1998). It has since disappeared from most of its former range and survives only in the W-Niger complex (W-NP and Tamou Total Reserve), where the last population estimate was about 350 individuals (0.1 ind./km²; Ouindeyama et al., Reference Ouindeyama, Chevillot and Akpona2021). A comparison with aerial counts conducted over the past two decades suggests a strong reduction in numbers since 2015 (2003: ~1200 individuals; 2015: ~1100 individuals; 2021: ~350 individuals; Bouché et al., 2003, Reference Bouché, Nzapa Mbeti Mange and Tankalet2015; Ouindeyama et al., Reference Ouindeyama, Chevillot and Akpona2021).
Nigeria
In the early twentieth century, the African buffalo was reported to be very common throughout Nigeria, from coastal evergreen forests (forest buffalo subspecies) to shrubby savannas in the north of the country (savanna buffalo subspecies). During the 1960s, the same author reports its occurrence in all suitable habitats, except for the southern coastal districts (Sidney, Reference Sidney1965). In the late twentieth century, East (Reference Dupuy1998) reported populations reduced to small, generally declining populations in a few protected areas.
For the West African savanna buffalo, the findings reported by East in 1999 still apply in 2022. The subspecies maintains an extremely limited distribution in northern Nigeria with a presence recorded only in three sites that are far from each other: Yankari Game Reserve, Kainji Lake NP and Gashaka Gumti NP (Andy Dunn, Naomi Matthews and Stuart Nixon, personal communication). The prospects for restoring the populations of Kainji Lake NP are poor due to their isolation from other populations and to the insecurity prevailing when this book went to press. Gashaka Gumti NP borders Faro NP in Cameroon, where about 600 individuals were estimated to occur (Elkan et al., Reference Elizalde, Elizalde and Lutondo2015). A transfrontier conservation strategy could pave the way for the restoration of a viable buffalo population in Gashaka Gumti NP when the political and security contexts on both sides of the border so allow.
The forest buffalo was once widespread in most southern areas of Nigeria (Sidney, Reference Sidney1965), but has been eliminated from most of its former range and reduced to small, generally declining populations in a few protected areas (East, Reference Dupuy1998). To date, this subspecies seems mainly localized in Cross River NP (4000 km2), where presence was reported from 2001 to 2019 (Eniang et al., unpublished data; Eniang et al., Reference Elkan, Vanleeuwe and Eldar2017; Bassey, Reference Bassey2019). In this NP bordering Cameroon, only 131 records of forest buffalo were reported for all years combined during line transect surveys (namely, in 2001, 2005, 2009, 2013; Eniang et al., Reference Elkan, Vanleeuwe and Eldar2017). Only one forest buffalo observation was reported in the Mbe Mountains corridor (linking the Afi Mountain Wildlife Sanctuary and the Okwangwo Division of Cross River NP) during a 2019 year-round anti-poaching patrol (Eban, Reference East2020). Buffalo presence was recently reported in Okomu NP in south-central Nigeria (Akinsorotan et al., Reference Akinsorotan, Odelola, Olaniyi and Oguntuase2021). In other words, the forest buffalo is near to extinction in Nigeria.
Senegal
West African savanna buffalo were formerly widespread in the southern savannas of this country (East, Reference Dupuy1998). The Senegalese buffalo population seems to have been isolated from other populations for some time (Sidney, Reference Sidney1965). Nowadays, populations have drastically declined and most buffalo are now only located in Niokolo–Koba NP. This protected area, where buffalo populations reached about 1000 individuals in the late 1960s (Dupuy, Reference Dunham and Westhuizen1971) now hosts about 500 buffalo (0.06 ind./km²; Rabeil et al., Reference Rabeil, Hejcmanová and Gueye2018). These figures are quite similar to those observed 12 years earlier (~500 individuals (0.05 ind./km²), Renaud et al., Reference Renaud, Gueye and Hejcmanová2006). Some buffalo are present in the private fenced reserves of Bandia (ranging from 80 to 134 individuals; 3 ind./km²; Raymond Snaps, personal communication; Holubová, Reference Hickey, Granjon and Vigilant2019) and Fathala (40; 1.7 ind./km²; Holubová, Reference Hickey, Granjon and Vigilant2019). The Bandia buffalo originated from 10 individuals translocated from Niokolo–Koba NP in 2000. It is worth noting that a relict population of savanna buffalo can still be found in the Faleme trophy hunting area (Philippe Chardonnet, personal communication).
Sierra Leone
Forest buffalo may still have occurred until a decade ago, mainly in the north of the country in 2009 and 2010 (Brncic et al., Reference Brncic, Amarasekaran and McKenna2015). No recent information is available from this country.
Togo
Until the mid-1950s, the African buffalo was found in most parts of the country (Baudenon, Reference Baudenon1952). Although classified as West African savanna buffalo, this author observed an important morphological gradient across the country, with black-coated buffalo in the north and red-coated buffalo in the south. According to East (Reference Dupuy1998), African buffalo survived in small to moderate numbers in the country’s protected areas until the early 1990s, but were expected to be close to extinction in the late 1990s.
From our investigations, it appears that African buffalo are still present in small numbers in several protected areas. In the two northern regions (Savanne and Kara), small populations were reported in Oti–Kéran NP (MERF, 2013) and Djamdè Faunal Reserve (MERF, 2014). In the Central Region, observations were made in Fazao–Malfakassa NP (Atsri et al., Reference Atsri, Adjossou and Tagbi2013) and Abdoulaye Game Faunal Reserve (MERF, 2017). Further south in the Plateaux Region, Amou Mono classified forest (MERF, 2016) and Togodo complex of protected areas (GIZ, Reference Gedow, Leeuw and Koech2017) also host small numbers.
Central Africa
Cameroon
Buffalo formerly occurred more or less throughout the country, except for the more arid parts of the far north (Sidney, Reference Sidney1965), with the West African savanna buffalo in northern and central Cameroon and the forest buffalo in the southern forests, which cover about half the country’s area (East, Reference Dupuy1998). In Cameroon, the savanna buffalo is now restricted to conservation areas in the North Region. In contrast, the forest buffalo is still present in forest areas sparsely populated by humans, especially in the South and East provinces, and to a lesser extent in the south-west province.
At the beginning of the twentieth century, West African savanna buffalo used to be common in the Logone floodplain, Far North Cameroon. However, in 1935, the African buffalo was already rare in the area, and no longer occurred when Waza NP was created in 1968 (Scholte, Reference Scholte2005). Remaining buffalo are located in the Bouba Ndjidda–Bénoué–Faro complex (North Province) with an overall estimated number of 2500 individuals (0.11 ind./km²; Elkan et al., Reference Elizalde, Elizalde and Lutondo2015 for Bénoué and Faro; Grossmann et al., Reference Gouteux, Blanc and Pounekrozou2018 for Bouba Ndjidda). It is hard to evaluate the proportion of animals within national parks or trophy hunting areas, but the last surveys (2015, 2018) tend to show that Bouba Ndjidda and Faro NPs still host buffalo, while for Bénoué, all individuals were spotted in the trophy hunting areas and none in the national park. The general trend seems to show a decrease in the population, estimated at 4000 individuals in 2008 (0.18 ind./km²; Omondi et al., Reference Omondi, Bitok, Tchamba and Lambert2008).
Over the past 15 years, the presence of the forest buffalo was reported in most of the protected areas and numerous logging concessions.
In south-west and north-west provinces (border with Nigeria), buffalo are present in Korup NP (Astaras, Reference Astaras2009) and were also sighted in a logging concession located north of the park. Further north, buffalo presence was reported in the Black Bush Area of Waindow in 2014 (Chuo and Angwafo, Reference Christy, Lahm, Pauwels and Weghe2017). These buffalo populations appear to be more scattered and isolated as they are surrounded by areas of high human population density. In this respect, it should be noted that no observations of buffalo have been reported recently in the West, Littoral and Central provinces, all of which are heavily populated. However, it is plausible that buffalo populations remain in the northern part of Central Province in the triangle formed by the Mpem-Djim, Mbam-Djerem, and Deng-Deng NPs. The presence of buffalo was observed there in a logging concession in 2004 (Cornélis et al., Reference Cornélis, Melletti, Renaud, Fonteyn, Bonhotal, Prins, Chardonnet and Caron2023).
In the South province, buffalo are present in Campo–Ma’an NP (650 km2), where population sizes were estimated by Bekhuis et al. (Reference Bekhuis, de Jong and Prins2008) with 20 individuals only (densities 0.01–0.04 ind./km2) and by Van der Hoeven, de Boer and Prins (Reference Hoeven, Boer and Prins2004) at 0.07–1.27 ind./km2. The presence of buffalo was also reported in several logging concessions located on the periphery of the park (Cornélis et al., Reference Cornélis, Melletti, Renaud, Fonteyn, Bonhotal, Prins, Chardonnet and Caron2023). Further east, forest buffalo also were reported in a logging concession located north of Mangame Wildlife sanctuary.
In the south-eastern end of the country (East Province), forest buffalo are present in Dja Biosphere Reserve (Bruce et al., Reference Bruce, Wacher and Ndinga2017, Reference Bruce, Amin and Wacher2018) and have been sighted in several logging concessions located south of the reserve over the past 15 years (Cornélis et al., Reference Cornélis, Melletti, Renaud, Fonteyn, Bonhotal, Prins, Chardonnet and Caron2023). Forest buffalo were reported in Nki and Boumba-Bek NPs in 2015 (Imbey et al., Reference Imbey, Mbezele and Ahanda2019; Ngaba and Tchamba, Reference Ngaba and Tchamba2019; Hongo et al., Reference Hoeven, Boer and Prins2020) and Lobeke NP (Gessner et al., Reference Gautier2013). The area surrounding these three protected areas is almost entirely allocated to logging. The presence of buffalo also has been reported in many logging concessions over the past 15 years (Cornélis et al., Reference Cornélis, Melletti, Renaud, Fonteyn, Bonhotal, Prins, Chardonnet and Caron2023).
Central African Republic
The Central African Republic (CAR) is the only country where three subspecies of buffalo occur.
The West African savanna buffalo subspecies used to be widespread in the west of the country next to the border with Cameroon, although its West African name looks odd in a Central African country. Nowadays, information is lacking about this subspecies in CAR, but it is certainly and by far the least represented of the three subspecies present in CAR. It may be reasonable to think that buffalo are present next to the Cameroon border because there are Trophy Hunting Areas (Zones d’Intérêt Cynégétique) on the Cameroon side with buffalo quota and offtake.
Central African savanna buffalo were historically widespread in all Central African savannas (East, Reference Dupuy1998). Presently, residual buffalo populations are apparently restricted to the far Northern complex (Bamingui–Bangoran and Manovo–Gounda St Floris NPs and surrounding trophy hunting areas) and in the Southeast complex (Chinko Basin).
In the Northern complex, the population numbers declined from ~19,000 individuals (0.3 ind./km²) in 1985 (Douglas-Hamilton et al., 1985) to ~13,000 (0.2 ind./km²) in 2005 (Renaud et al., Reference Renaud, Fay and Abdoulaye2005), after which it collapsed to 13 individuals only in 2017 (Elkan et al., Reference Elkan, Mwinyihali and Mendiguetti2017). Given the level of insecurity in the region, it may have now gone extinct.
In the Southeast complex, buffalo populations strongly declined between 2012 and 2017 due to the invasion of the area by transhumant herders from South Darfur, Sudan (Aebischer et al., Reference Aebischer, Ibrahim and Hickisch2020). Conservation efforts undertaken by African Parks since 2014 have reversed the trend in the Chinko conservation area (6000 km2), where the buffalo population was estimated at over 4000 buffalo in 2022 (Thierry Aebischer, personal communication).
The huge uninhabited wilderness areas in between those residual complexes are composed of trophy hunting areas where buffalo were present and hunted before the 2012 war started. However, no recent information on buffalo presence or abundance is available.
Although a large part of potential suitable areas has not been surveyed recently, the conservation status of the Central African savanna buffalo should be considered as under major threat in the Central African Republic (see also Scholte et al., Reference Scholte, Pays and Adam2022).
Forest buffalo are mainly localized in the south-west tip of the country, covered by rainforests. Over the past 15 years, the presence of the forest buffalo was reported in all protected areas and most of the logging concessions of this region (Cornélis et al., Reference Cornélis, Melletti, Renaud, Fonteyn, Bonhotal, Prins, Chardonnet and Caron2023). Buffalo are encountered in the Dzanga-Sangha Protected Area complex, including recent records in the Special Reserve of Dzanga-Sangha (Melletti et al. Reference Melletti, Penteriani and Boitani2007 Beudels‐Jamar et al., Reference Beudels‐Jamar, Lafontaine and Robert2016). Forest buffalo were also reported from the south-east part of the country, where forest and savanna intermingle: Bangassou forest (Roulet, Reference Roulet2006) and the thick riverine forests of the upper Mbomou River (Philippe Chardonnet, personal communication).
Chad
Central African savanna buffalo were formerly widespread in the south of the country from Lake Chad to Salamat (Sidney, Reference Sidney1965). However, buffalo were extirpated from most of their original range by agricultural and livestock expansions as well as drought (East, Reference Dupuy1998). The largest population, estimated at ~15,500 individuals (5 ind./km²), is located in Zakouma NP (Fraticelli et al., Reference Fonteyn, Vermeulen and Deflandre2021). In this protected area, the buffalo population tripled in 15 years, showing an average annual growth rate of 7 per cent. In January 2022, 905 buffalo were translocated from Zakouma NP to restock the nearby Siniaka-Minia wildlife reserve (Naftali Honig, personal communication).
The last survey in Sena Oura NP did not encounter any buffalo (Elkan et al., Reference Elizalde, Elizalde and Lutondo2015). Some buffalo were reported in the far south province of Logone Oriental near Monts de Lam and Baïbokoum in 2021 (Matuštíková, Reference Matuštíková2021), suggesting that buffalo populations of Bamingui-Bangoran/Manovo-Gounda St Floris in the Central African Republic and of Bouba N’djidda complex in Cameroon could have some connection through southern Chad.
Democratic Republic of Congo
Forest buffalo seem to be widespread in the Democratic Republic of Congo (DRC; informally known as ‘Congo-Kinshasa’), but with a patchy distribution because DRC is one of the most densely populated countries in the Congo Basin. This may be due to a combination of both human pressure (e.g. poaching, meat harvesting for logging camps and for other extractive industries) and a lack of knowledge.
In the south-western section of the DRC forest block (Maï-Ndombe and Equateur Provinces), the presence of buffalo was reported in Tumba-Ledima Reserve (ICCN and WWF, 2016a) and Ngiri Triangle Reserve (T. Breuer, personal observation). The presence of the forest buffalo was recorded during the forest management surveys of many logging concessions over the past 15 years, particularly in the western and south-eastern parts of Mai-Ndombe Province (Cornélis et al., Reference Cornélis, Melletti, Renaud, Fonteyn, Bonhotal, Prins, Chardonnet and Caron2023).
In the south-central section of the DRC forest block (north of Kasai and Sankuru provinces, south of Tshuapa Province), forest buffalo were recently reported in Salonga NP (Bessone et al., Reference Bessone, Kühl and Hohmann2020) and the Tshuapa–Lomami–Lualaba landscape (John Hart, personal communication). Although highly possible, the presence of buffalo was not reported (to our knowledge) from Sankuru Reserve.
In the south-east section of the DRC forest block (Maniema and South Kivu Provinces), forest buffalo were reported in Kahuzi–Biega NP (Spira et al., Reference Spira, Mitamba and Kirkby2018), Kasongo and Pangi priority areas (ICCN and WWF, 2017a), the Itombwe NR (ICCN and WWF, 2016b) and in the Luama–Kivu region (ICCN and WWF, 2017b).
In the north-central section of the DRC forest block, the presence of buffalo has been confirmed in a dozen places of Tshopo Province over the last 15 years by several studies (van Vliet et al., Reference Van Vliet, Nebesse and Gambalemoke2012; Nebesse, Reference Nebesse2016) and forest management surveys (Cornélis et al., Reference Cornélis, Melletti, Renaud, Fonteyn, Bonhotal, Prins, Chardonnet and Caron2023). Further west, its presence was recorded in Abumonbazi Reserve (province of Nord-Ubangi; ICCN and WWF, 2015a) and in a logging concession (‘09/11-Baulu’) located south of Lomako–Yokokala Reserve (north of Tshuapa Province; Cornélis et al., Reference Cornélis, Melletti, Renaud, Fonteyn, Bonhotal, Prins, Chardonnet and Caron2023).
In the eastern section of the DRC forest block, forest buffalo were reported north of Maiko NP (Naomi Matthews and Stuart Nixon, personal communication) and in the southern section of Virunga NP (Mikeno Sector; Hickey et al., Reference Hauptfleisch and Brown2019). Further north (Ituri province), buffalo were reported in Okapi Wildlife Reserve (Madidi et al., Reference Madidi, Maisels and Kahindo2019).
Interestingly, the presence of buffalo also has been reported south of the current ‘official’ (IUCN SSC Antelope Specialist Group, 2019) range of the forest buffalo, in trophy hunting areas: Bombo Lumene (ICCN and WWF, 2017c), Bushimaie (ICCN and WWF, 2016c), and Swa-Kibula (ICCN and WWF, Reference Houngbégnon2015b) as well as in Mangai Nature Reserve (ICCN and WWF, Reference Hoppe‐Dominik, Kühl, Radl and Fischer2015c) and Kaniama Elephant Refuge (ICCN and WWF, 2016d). The taxonomic status of these populations is unclear.
Central African savanna buffalo formerly occurred on the edge of the dense forest along the northern and eastern borders of the country (East, Reference Dupuy1998). Much hunted and regularly infected with rinderpest, buffalo populations became isolated in Garamba NP (Northern border), where Sudanese meat hunters reduced the population from 53,000 in 1976–83 to 26,000 in 1995 (East, Reference Dupuy1998). Nowadays, Garamba NP and adjacent trophy hunting areas (~14,800 km2) host about 9400 buffalo (0.6 ind./km²; Ngoma et al., Reference Ngoma, Diodio and Dieudonné2021). The population in Garamba NP may have increased slightly from some 6000 individuals in 2012 (Bolaños, Reference Bolaños2012) with improved protection of the park. Population estimates in Bili–Uere NP are unknown, but most probably low with a few small groups left (Elkan et al., Reference Elkan, Hamley and Mendiguetti2013b; Jef Dupain, personal communication, 2018).
Cape buffalo – in the east of the DRC, along the border with Uganda, the central plains of Virunga NP are host to a population of savanna buffalo located in a zone of introgression between several subspecies and which we have assigned to the ‘caffer’ subspecies. The population of Virunga NP has decreased from about 2100 individuals in 2010 (Plumptre et al., Reference Plumptre, Kujirakwinja and Moyer2010) to about 600 (Wanyama et al., Reference Wanyama, Balole and Elkan2014).
In the southern savannas, the population of Upemba NP is less well monitored, but we think it is very small as only 15 individuals were spotted in 2009 (Vanleeuwe et al., Reference Vanleeuwe, Henschel and Pélissier2009). East (Reference Dupuy1998) stated that buffalo were eliminated from the Kundelungu NP population and we have not received any contradicting information.
Equatorial Guinea
There is evidence of the former widespread occurrence of forest buffalo on Bioko Island, Equatorial Guinea, but no indication of a surviving population was found during 4.5 months of field surveys there between 1986 and 1992 (Butynski et al., Reference Butynski, Schaaf and Hearn1997). Due to overhunting, buffalo were probably already extirpated from the island between 1860 and 1910.
In the mainland region of the country, the forest buffalo formerly occurred throughout Mbini (Rio Muni). It has been eradicated from parts of its range but seems to have survived locally within the remaining forested areas, including Monte Alen NP until the end of the 1990s (East, Reference Dupuy1998).
Gabon
Gabon is a sparsely populated country, 88 per cent of which is covered by equatorial forests. The country is home to a largely preserved biodiversity. Thirteen national parks were created in 2002 and protected areas cover 15 per cent of the country (41,000 km2). About half of the country (~142,000 km2) is dedicated to logging (WRI, 2013). Forest buffalo populations are widely distributed in Gabon both inside and outside protected areas, including logging and oil concessions (Prins and Reitsma, Reference Prins and Reitsma1989). Except for Akanda NP, located 30 km north of Libreville, the presence of the forest buffalo has been documented in all of the national parks over the past 15 years (Christy et al., Reference Chase, Schlossberg, Sutcliffe and Seonyatseng2008; Vanthomme et al., Reference Vanthomme, Kolowski, Korte and Alonso2013; Nakashima, Reference Nakashima2015; Hedwig et al., Reference Haurez, Petre and Vermeulen2018). For this country, we reviewed 42 reports of biodiversity inventories conducted on foot by international forestry consultancy companies in logging concessions between 2000 and 2017 (unpublished and confidential reports). Almost all of these inventories recorded evidence of buffalo presence. These observations are supported by recent surveys conducted in several logging concessions using camera traps (Houngbégnon, Reference Hongo, Dzefack and Vernyuy2015; Nunez et al., Reference Nunez, Froese and Meier2019; Fonteyn et al., Reference Fonteyn, Vermeulen and Deflandre2021; Naomi Matthews and Stuart Nixon, personal communication).
Estimates of buffalo populations were carried out in a forest–savanna mosaic area of Lopé NP (North sector 70 km2) where Korte (Reference Korte2008b) estimated about 300 individuals in 18 herds with a density of 5 ind./km2. In forest areas at Lopé NP, White (Reference White1994) estimated a density of 0.42 ind./km2. In the Réserve de Faune de Petit Loango, Morgan (Reference Morgan2007) found a density of 1.7 ind./km2. Prins and Reitsma (Reference Prins and Reitsma1989) reported a forest buffalo density of 0.51 ind./km2, but absolute numbers could not be established reliably.
Republic of Congo
Republic of Congo (informally known as ‘Congo-Brazzaville’) is a sparsely populated country, 70 per cent of which is covered by equatorial forests. The central part of the country is made up of the so-called Bateke plateaus, which are covered with savanna grassland and riverine forests.
In the northern part of the country, which is very sparsely populated, the forests of the Congolese basin are home to widely distributed buffalo populations. Forest buffalo are present in all of the protected areas: Odzala-Kokoua NP (Chamberlan et al., Reference Chamberlan, Maurois and Marechal1995), Nouabalé-Ndoki NP (Blake, Reference Blake2002), Ntokou-Pikounda NP (Malonga and Nganga, Reference Malonga and Nganga2008), and Lac Tele Reserve (Devers and Van de Weghe, Reference Devers and Weghe2006). Between these protected areas, upland forests are allocated to logging. For this area, we reviewed 10 biodiversity survey reports conducted between 2005 and 2019 by international forestry consultancy companies in 10 logging concessions (unpublished and confidential reports). All of them recorded evidence of buffalo presence. In northern Congo, Blake (Reference Blake2002) recorded densities between 0.01 and 0.04 ind./km2 at Nouabalé-Ndoki NP, while Chamberlan et al. (Reference Chamberlan, Maurois and Marechal1995) estimated the buffalo population of Odzala-Kokoua NP at around 500 individuals (0.4 ind. km2).
From the central part of the country, little information is available on the presence of forest buffalo in the savannas and gallery forests of the Bateke Plateau. However, Mathot et al. (Reference Mathot, Ikoli and Missilou2006) report buffalo presence in the Lessio-Luna Wildlife Sanctuary bordering the Lefini Reserve.
In the southern part of the country, forest buffalo are present in Conkouati-Douli NP (Devers and Van de Weghe, Reference Da Silva, Minhos and Sa2006) and Kouilou Department (Orban et al., Reference Orban, Kabafouako and Morley2018). In Niari and Lekoumou Departments, the biodiversity survey reports conducted in the logging concessions between 2005 and 2019 on foot also reported evidence of buffalo presence.
East Africa
Burundi
A resident population of cape buffalo has been living for a long time and continues to do so today in the narrow strip of Ruvubu NP, Eastern Burundi (Nzigidahera et al., Reference Nzigidahera, Mbarushimana, Habonimana and Habiyaremye2020).
Ethiopia
African buffalo populations have long been restricted to the south-western and western parts of the country, along the borders of Kenya, South Sudan and Sudan (Sidney, Reference Sidney1965). East (Reference East1998) reported that the main populations can be found in Omo and Mago NPs. Buffalo do also occur in montane forests and swampy wetlands, such as in the Chebera Churchura (Megaze et al., Reference Megaze, Balakrishnan and Belay2018) and Gambella NPs (TFCI, 2010; Rolkier et al., Reference Rolkier, Yehestial and Prasse2015). Currently their distribution is largely confined to protected areas with a total estimated population of about 15,000 (around 5000 S. c. aequinoctialis and 10,000 S. c. caffer – Table 4.1).
Ethiopia is a contact zone between the Cape buffalo and the Central African savanna buffalo where the two subspecies intergrade. The presence of intermediate phenotypes and the absence of geographical barriers make classification difficult. For the sake of consistency with earlier studies on buffalo distribution (East, Reference Dupuy1998; Cornélis et al., Reference Cornélis, Melletti, Korte, Melletti and Burton2014), our results are presented in accordance with the current IUCN subspecies range (IUCN SSC Antelope Specialist Group, 2019), but this is one of the areas where the ‘subspecies concept’ loses meaning for African buffalo.
The largest population of Cape buffalo is found in Chebera Churchura NP (~5200 animals; Megaze et al., Reference Macandza, Bento and Roberto2017). Significant populations are also found in other formally protected areas, such as Omo NP (~800 animals; Tola, Reference Tola2020), Mago NP (~850 animals; Tsegaye, Reference Tsegaye2020). In addition, about 2000 buffalo are estimated to be in the Tama wildlife reserve that connects Omo and Mago NPs (Girma Timer, personal communication). Finally, the Weleshet-Sala controlled trophy hunting area holds about 1100 individuals (Kebede et al., Reference Kebede, Timer and Gebre-Michael2011).
Significant populations of Central African savanna buffalo are found in Gambella NP (~1400 animals; TFCI, 2010). Reports from two newly established national parks indicate the presence of about 1700 buffalo in Maokomo Nature Reserve (Wendim, Reference Wendim2015). The presence of several hundred buffalo was also confirmed by a count in Dati Wolel NP (Gonfa et al., 2015), but the population estimate is not reliable. Buffalo observations were also recently reported from Alatash NP along the border with Sudan (Bauer et al., Reference Bauer, Mohammed and El Faki2018).
During the last decade, several Central African savanna buffalo populations have been reported north-east of their earlier established (IUCN SSC Antelope Specialist Group, 2019) distribution range. Two Controlled Hunting Areas (CHAs) hold a reasonable number of buffalo: Haro Aba Diko CHA (~900 individuals; Kebede and Tsegaye, Reference Kebede and Tsegaye2012) and Beroye CHA (~600 individuals; Kebede et al., Reference Kebede, Wendim and Abdulwahid2013). A population of at least 60 buffalo was reported in Didessa NP (Wendim, Reference Wendim2018) and a herd of seven animals was repeatedly observed in Jorgo-Wato National Forest Priority Area (Jebessa, Reference Jebessa2015; Erena et al., Reference Eniang, Ebin and Nchor2019). Finally, Lafto Forests area hosts about 340 buffalo (Dandena and Dinkisa, Reference Dalimier, Achard, Delhez, Eba’a Atyi, Hiol Hiol and Lescuyer2014). Yet we repeat that ‘subspecies’ designation in this country is shaky due to intergradation.
Kenya
The Cape buffalo was formerly widespread throughout southern and central Kenya, and on isolated, forested hills and mountains in the north. In the 1990s, the population became largely confined to protected areas, except in Laikipia and Lamu districts (East, Reference Dupuy1998). The status of buffalo in Kenya has recently been updated during a national wildlife census undertaken between April and July 2021. Several aerial total counts covered nearly 60 per cent of Kenya’s land mass (Waweru et al., Reference Waweru, Omondi and Ngene2021). Results show that the Cape buffalo is distributed in almost all of the wildlife ecosystems surveyed, except in the northern counties of Mandera, Wajir, Turkana as well as the Nasolot-Kerio Valley ecosystem. About 41,700 buffalo were counted.
In Kenya, seven conservation areas host populations of over 1000 buffalo, respectively, Maasai Mara ecosystem (~11,600), Tsavo ecosystem (~8000), Lake Nakuru NP (~6500), Laikipia–Samburu–Marsabit ecosystem (~6300), Lamu–Lower Tana and Garissa ecosystem (~3000), Meru ecosystem (~2600) and Naivasha–Nakuru ranches (~1500). These seven ecosystems account for about 95 per cent of Kenya’s total buffalo population. Three conservation areas contain a few hundred buffalo, namely Nairobi NP (~1000), Amboseli–Magadi ecosystem (~500) and Ruma NP (~400). Small populations occur in other protected areas, such as Athi–Kapiti ecosystem, Mwea National Reserve, Shimba Hills National Reserve and Oldonyo Sabuk NP. Other populations have not been estimated over the years because the technique of aerial surveys is not well suited for dense forests and nature of the terrain of Aberdares, Mount Kenya, and Mount Elgon forested areas or Forest Reserves such as Mukogodo, Ngare Ndare Arabuko Sokoke and Boni Dodori. For this reason, but also because some buffalo strongholds were not surveyed under optimal visibility conditions (e.g. Lamu–Lower Garissa and Tana River Ecosystem with about 13,800 buffalo in 2015), the above-mentioned figure for the Kenyan population of buffalo is likely underestimated.
According to the latest national census (Waweru et al., Reference Waweru, Omondi and Ngene2021), buffalo in Kenya are now largely confined to protected areas. In the Mara ecosystem, 70 per cent of all buffalo were found in the Maasai Mara National Reserve, while the remaining 30 per cent were recorded from the Maasai Mara community conservancies. In the Tsavo ecosystem, 80 per cent of the buffalo population was found inside the protected areas. In the Laikipia–Samburu–Marsabit–Meru ecosystem, 69 per cent of the population was counted in ranches, 27 per cent in the protected areas and 3 per cent in community/settlement areas.
In Kenya, buffalo populations suffered a sharp contraction in the 1990s because of severe drought and the very last rinderpest events. For example, the Mara population was reduced from 12,200 to 3100 by the 1993–94 drought (East, Reference Dupuy1998) and has since shown good recovery (~11,600 animals in 2021). The buffalo population in Nakuru NP has recovered and has consistently increased from about 2200 buffalo in the year 2000 to the current population of about 6400 individuals at a density of 51.3 buffalo/km2 (a continental record for the present; Lake Manyara National Park in Tanzania reached nearly twice as much: Prins and Douglas-Hamilton, Reference Prins and Douglas-Hamilton1990). In contrast, the Tsavo population decreased from an estimated 34,600 in 1991 to 5500 in 1997 (East, Reference Dupuy1998) with no strong evidence of recovery so far (~8000 in 2021). Although the Kenyan population shows a cumulative increase of about 40 per cent between 2008 and 2021, recovering the numbers from the early 1990s (approximately 95,000 buffalo) is challenging in a context of increasing competition with humans and cattle for resources (water, space and forage; Waweru et al., Reference Waweru, Omondi and Ngene2021). The effects of the 2022 drought with some heavy buffalo mortality in, for example, Lewa Downs (Susan Brown, personal communication), were not yet known when we finalized this chapter.
Rwanda
The Cape buffalo formerly occurred at high densities in Akagera and Volcanoes NPs. The population of Akagera NP, estimated to number 10,000 in 1990 (East, Reference Dupuy1998), subsequently declined due to the 1994 genocide and political unrest. The park also faced a two-thirds reduction in size to about 1120 km2. Since 2002, buffalo numbers have been increasing again, reaching ~3400 individuals in 2019 (Macpherson, Reference Macpherson2019).
In Volcanoes NP, a dung count undertaken in 2004 suggested a population of ~900 (2.0 ind./km2; Owiunji et al., Reference Owiunji, Nkuutu and Kujirakwinja2005). We are not aware of more recent surveys, but given the excellent protection of Volcanoes NP, as testified by the increasing number of mountain gorillas, we assume that the number of buffalo remained constant. In contrast, the buffalo was reported extinct at Lake Kivu shore and nearby forests (including Gishwati–Mukura) as well as Nyungwe NP (Cockar, Reference Cockar2022).
Somalia
The Cape buffalo formerly occurred in the south of the country in areas with permanent water along the lower Shebelle and lower Juba Rivers (Fagotto, 1980). At the end of the twentieth century, agricultural settlement and hunting pressure eliminated the buffalo from most of its former range, except in the Bushbush NP area (now Lag Badana NP), where it occurred in good numbers (East, Reference Dupuy1998). Buffalo presence was recently reported in Lag Badana National Park and surrounding areas in Jubalan (Gedow et al., Reference Fusari, Lopes Pereira and Dias2017), but the total number was not reported.
South Sudan
Sidney (Reference Sidney1965) reported that large herds of Central African savanna buffalo were commonly found in grassy plains. Although variations in numbers could be found, the subspecies population was very healthy in South Sudan, with probably several tens of thousands of individuals. Small migrations between the rainy and dry seasons were also observed. East (Reference Dupuy1998) also reported large populations of several thousand individuals in the main South Sudan protected areas (Boma NP and Shambe Nature Reserve), but warned that meat hunting pressure was very high. This declining trend appears to remain in process. Fay et al. (Reference Fargeot, Drouet-Hoguet and Le Bel2007) recorded ~10,200 buffalo in the protected areas of Southern Sudan (mainly in Zeraf and Sambe game reserves), while aerial reconnaissance surveys spotted only 285 individuals in 2013 and none in 2016 (Elkan et al., Reference Elkan, Fotso and Hamley2013a, Reference Elkan, Hamley and Mendiguetti2016). From this we infer that the conservation status of the Central African savanna buffalo should be considered under major threat in South Sudan.
Sudan
The Central African savanna buffalo is historically present in the south-eastern tip of Sudan along the border with Ethiopia. Bauer et al. (Reference Bauer, Mohammed and El Faki2018) reported observations of African buffalo in the Dinder–Alatash transboundary protected area (13,000 km2; Sudan and Ethiopia) during five field trips undertaken between 2015 and 2018.
Tanzania
Although once common throughout the country, the range of the Cape buffalo covered less than half its area of distribution at the end of the last century, with an estimated population of 342,000 individuals (East, Reference Dupuy1998). Tanzania today is still the country with by far the largest number of buffalo, with an estimated population of at least 240,000 individuals. The country has established a dense network of protected areas covering slightly more than 30 per cent of the land surface area (MNRT, 2021) and implementing a range of nature conservation models with both (i) consumptive use of wildlife in Game Reserves, Game Controlled Areas and Wildlife Management Areas (WMAs) and (ii) non-consumptive use of wildlife in National Parks and Ngorongoro Conservation Area.
Tanzania is the only country with single populations of buffalo exceeding 50,000 individuals (Figure 4.6). There are three of these strongholds: (i) the Serengeti Ecosystem (~23,700 km2) in the northern part of the country hosts a population of about 69,000 individuals (TAWIRI, 2021a), (ii) the Selous–Mikumi Ecosystem (~74,000 km2) in the south-east of the country has a population of about 66,800 individuals (TAWIRI, 2019a) and (iii) the adjacent Katavi–Rukwa and Ruaha–Rungwa Ecosystems (~83,000 km2) located in the west-centre of the country hold a population of about 53,000 individuals (TAWIRI, 2022).
Nearby the Serengeti Ecosystem, the Tarangire–Manyara Ecosystem (~15,500 km2) also hosts an important buffalo population, estimated at about 19,000 individuals (TAWIRI, 2020). Mkomazi NP (~2800 km2) in the north-east supports about 600 individuals (TAWIRI, 2019b). Saadani NP and Wami Mbiki WMA on the coast host about 1000 individuals (Edward Kohi, personal communication). By contrast, the last census undertaken in West Kilimanjaro–Lake Natron Ecosystem (~10,000 km2) reported a population of 46 buffalo only (TAWIRI, 2021b), a very low number largely due to cattle encroachment (Prins and De Jong, Reference Prins, De Jong, Kiffner, Bond and Lee2022).
In the north-western part of the country, the Malagarasi–Muyovozi Ecosystem hosts an important buffalo population, estimated at about 28,300 individuals (Edward Kohi, personal communication). The recently created Burigi–Chato NP (2200 km2) west of Lake Victoria along the border with Rwanda has a small number of buffalo, as well as Rubondo Island NP in Lake Victoria (Edward Kohi, personal communication). At the northwestern tip of the country, Ibanda Kyerwa NP (200 km2) supports about 215 buffalo (Edward Kohi, personal communication).
Finally, several mountainous and/or densely forested areas host buffalo populations of which the recent numbers are unknown: Arusha NP and Mount Meru Forest Reserve, Kilimanjaro NP and Udzungwa Mountains NP. Therefore, given these knowledge gaps, the estimates provided for Tanzania should be considered as minimum values.
In 2022, the conservation status of buffalo in Tanzania is uneven. On the positive side, (i) not only is Tanzania the only country holding single populations of over 50,000 buffalo, but there are three of these populations in the country; and (ii) several ecosystems show positive trends with growing buffalo populations, for example Serengeti Ecosystem. On a more worrying side, the overall national trend of buffalo is on the decrease due to (i) severe encroachment by livestock tending to replace buffalo in several ecosystems (Prins, Reference Prins1992; Musika et al., Reference Musika, Wakibara, Ndakidemi and Treydte2021, Reference Musika, Wakibara, Ndakidemi and Treydte2022; Prins and De Jong, Reference Prins, De Jong, Kiffner, Bond and Lee2022) and (ii) steady agricultural expansion and associated settlements.
Uganda
The Cape buffalo was formerly widespread in large numbers in savannas, with putative intermediates with the forest buffalo in the southwest (Greater Virunga Landscape) (East, Reference East1998). However, genetic samples so far have not yet recognized these putative hybrids in Uganda (see Chapter 3). It is noteworthy that western Uganda is a zone of introgression between several subspecies where the taxonomy is subject to controversy.
Cape buffalo are now confined to three conservation areas. In Queen Elizabeth NP (2110 km2), the most recent survey reported ~15,800 buffalo (Wanyama et al., Reference Wanyama, Balole and Elkan2014) and the population seemed to be increasing from the ~10,300 reported in 2010 (Plumptre et al., Reference Plumptre, Kujirakwinja and Moyer2010). In Murchison Falls NP and surrounding wildlife reserves (5030 km2), an aerial survey undertaken in 2016 resulted in an estimate of ~15,200 buffalo (Lamprey et al., Reference Lamprey, Ochanda and Brett2020), and the population also seemed to be increasing (from ~9200 in 2010 (Rwetsiba and Nuwamanya, Reference Rwetsiba and Nuwamanya2010). Finally, in Kidepo NP and Karenga Community Wildlife area (2400 km2), the last survey reported ~7500 individuals, mainly located inside the park (~6600; Wanyama et al., Reference Wanyama, Kisame and Owor2019). The trend is also up in Kidepo NP (from ~3800 in 2008 to ~6600 in 2019; WCS Flight Programme, 2008). These three conservation areas (together ~9400 km2) host a population of about 38,500 buffalo (4 buffalo/km2). Smaller populations were also recently reported in Lake Mburo Conservation Area (1290 km2 with ~1500 buffalo; Kisame et al., Reference Kisame, Wanyama, Buhanga and Rwetsiba2018a) and Pian Upe Wildlife Reserve (no estimate; Kisame et al., Reference Kisame, Wanyama, Buhanga and Rwetsiba2018b). Hence, the total number of Cape buffalo in Uganda is now about 40,000 head, which compares very favourably to the estimate of about 22,000 a few decades ago (East, Reference Dupuy1998).
The presence of forest buffalo was recently confirmed in Semuliki NP in 2020 (Naomi Matthews and Stuart Nixon, personal communication). No forest buffalo presence was reported from Mgahinga Gorilla NP since 2003 (Hickey et al., Reference Hauptfleisch and Brown2019) or from Kibale NP from 2013 to 2021 (Rafael Reyna-Hurtado and Jean-Pierre d’Huart, personal communication). In the latter park, records of buffalo inside the forest are related to savanna buffalo coming from Queen Elizabeth NP through the Dura corridor.
Southern Africa
Angola
Apart from the arid coastal strip in the southwest, African buffalo formerly occurred very widely, with the Cape buffalo in the south and intermediate forms with the forest buffalo in the north (East, Reference Dupuy1998). During the civil war (1975–2002), thousands of buffalo were slaughtered by the Angolan army for food. Since the 2000s, buffalo populations have remained low due to widespread poaching, habitat degradation, human encroachment and the presence of land mines. However, very little information is available on the status of buffalo in this country, especially in the central plateau and the northern and eastern regions.
Cape buffalo are still relatively common in the south-eastern parts of Angola, especially in the Mucusso region and in Mavinga and Luengue-Luiana NPs (Funston et al., Reference Fraticelli, Ourde and Arnulphy2017; Beja et al., Reference Beja, Pinto, Veríssimo, Huntley, Russo, Lages and Ferrand2019; Petracca et al., Reference Petracca, Funston and Henschel2020), but their actual numbers have not been assessed. Naidoo et al. (Reference Naidoo, Preez, Stuart-Hill and Beytell2014) report frequent movements of Cape buffalo between Angola and Namibia, particularly along the northern banks of the Okavango River, and west of the Cuando River. Large herds (over 1000 animals) were also reported to aggregate in the southeast of Luiengi–Luiana NP along the Kwando River just before the rainy season (Roland Goetz, personal communication).
In the northern Quiçama region, there were an estimated 8000 so-called ‘forest buffalo’ prior to the civil war of 1975–2002 (Braga-Pereira et al., Reference Braga-Pereira, Peres and Campos-Silva2020). During the war, uncontrolled poaching severely reduced the populations, which are now confined to a few small herds in Quiçama NP (Groom et al., Reference Gonfa, Gadisa and Habitamu2018), Luando Natural Integral Reserve (Elizalde et al., Reference Eban2019) and Cangandala NP (David Elizalde, personal communication). Although surprising at this latitude, recent photographs of buffalo taken by camera traps in Quiçama NP and Luando Natural Integral Reserve confirm the presence of buffalo that phenotypically correspond to the forest buffalo (David Elizalde, personal communication). Outside protected areas, recent sightings of buffalo were reported in the north-western section of the country, in the region of Mussera (Zaire Province), Quissafo-Ndalatando and Cassoxi (Cuanza Norte Province), and in the Pingano Mountains (Uige Province) (David Elizalde, personal communication).
Botswana
Cape buffalo are found only north of 20° S in the Okavango–Chobe region and wildlife movements are constrained by veterinary fences erected to control the spread of livestock diseases. In a 2018 aerial total count covering northern Botswana (~103,700 km2, including Moremi Game Reserve, Chobe NP, Makgadikgadi Nxai Pan NP and surrounding WMAs), the buffalo population was estimated to be some 28,500 individuals (Chase et al., Reference Chase2018). For the record, a similar survey undertaken in 2010 reported an estimate of 39,600 individuals (Chase, Reference Chardonnet, Fusari and Dias2011), while East (Reference Dupuy1998) reported about 27,000 head. It thus appears that the population is fairly constant.
Eswatini (Swaziland)
Cape buffalo were reintroduced in Swaziland, where the indigenous population was extirpated (Tambling et al., Reference Tambling, Venter, Toit, Child, Child, Roxburgh, Do Linh San, Raimondo and Davies-Mostert2016). They now occur in the Mkhaya Private Game Reserve (~20 animals, 0.2 ind./km2; Tal Fineberg, personal communication, 2021).
Lesotho
Buffalo was extirpated from this country (Tambling et al., Reference Tambling, Venter, Toit, Child, Child, Roxburgh, Do Linh San, Raimondo and Davies-Mostert2016), but historically it had occurred here even though it was no longer present a few decades ago (East, Reference Dupuy1998).
Malawi
In the late 1990s, the Cape buffalo was confined to protected areas such as Lengwe, Kasungu and Nyika NPs as well as Nkhotakota and Vwaza Marsh Game Reserves. Their population was estimated at about 1850 individuals (East, Reference Dupuy1998).
To our knowledge, buffalo occur today in Majete and Nkhotakota Wildlife Reserves as well as Liwonde and Kasungu NPs. In Majete Wildlife Reserve, where 306 buffalo were reintroduced between 2006 and 2010, the buffalo population was estimated at ~1800 individuals in 2020 (Sievert and Adenorff, Reference Sievert and Adenorff2020). Between 2016 and 2017, over 100 buffalo were moved from Majete Wildlife Reserve and Liwonde NP to Nkhotakota Wildlife Reserve as part of a rehabilitation programme undertaken by African Parks. Similarly, 80 buffalo were translocated from Liwonde to Kasungu NP in 2022 as part of a restoration programme (African Parks, personal communication).
Mozambique
Cape buffalo populations occurred throughout the country until the 1970s, but suffered greatly from 25 years of war (independence war 1964–1974 then civil war 1977–1992) (East, Reference Dupuy1998). Buffalo are well present in the northern part of the country (Niassa and Cabo Delgado Provinces). In Niassa Special Reserve, they were successively estimated at 6800 (2009), 6200 (2011) and 7100 (2014) individuals (Craig, Reference Craig2011a; Grossmann et al., Reference Grossmann, Lopes Pereira and Chambal2014a), with a density surprisingly more than five times lower than in the neighbouring Selous complex in Tanzania. In Quirimbas NP and adjacent areas, aerial sample counts undertaken in 2011 and 2014, respectively, reported 0 and 88 buffalo observations with no population estimate (Craig, Reference Craig2011b; Grossmann et al., Reference Grossmann, Lopes Pereira and Chambal2014b). We did not obtain figures for the buffalo occurring in the Chipanje Chetu community-based natural resource management initiative (6500 km²) north-west of Niassa Special Reserve, and for the numerous hunting blocks outside the reserve in the two northern provinces.
Further south, in Zambezia Province, Gilé National Reserve embarked on a restoration programme by reintroducing extinct large mammal species such as buffalo: 67 buffalo were reintroduced in 2012 and 2013–2020 from the Marromeu complex (the National Reserve and numerous trophy hunting areas) and Gorongosa NP, then 47 buffalo from the trophy hunting areas within the Niassa Special Reserve (Chardonnet et al., Reference Chardonnet, Fusari and Dias2017; Fusari et al., Reference Fusari, Lopes Pereira and Dias2017). The population in the now Gilé NP was estimated at about 139 individuals in 2017 (Macandza et al., Reference Macandza, Bento and Roberto2017). Mahimba Game Reserve, north bank of Zambezi River, would also host around 850 individuals (Grant Taylor, personal communication). In Tete Province, an aerial survey conducted in 2014 south and north of Lake Cahora Bassa and Magoe NP including the Tchuma Tchato community programme reported 4300 buffalo (Grossmann et al., Reference Grossmann, Lopes Pereira and Chambal2014c).
The largest African buffalo population of Mozambique is located south (right bank) of Zambezi River (Manica and Sofala Provinces). At the mouth of the Zambezi River into the Indian ocean (the famous Zambezi delta), the open floodplains of the Marromeu Game Reserve and surrounding trophy hunting areas (‘Coutadas’) host about 21,300 individuals according to the latest aerial total count (Macandza et al., Reference Macandza, Ntumi and Mamugy2020). Gorongosa NP was restocked between 2006 and 2011 with 186 buffalo from Kruger and Limpopo NPs (Carlos L. Pereira, personal communication). An aerial total count conducted in 2020 reported 1200 buffalo (Stalmans and Peel, Reference Stalmans and Peel2020). Finally, the trophy hunting areas located northwest of Gorongosa NP likely hold about 1000 buffalo (Willie Prinsloo, Joao Simoes Almeida and Grant Taylor, personal communication).
The Great Limpopo Transfrontier Conservation Area lies in South-Central and Southern Mozambique. In its northern section (Inhambane Province), a restoration programme has been underway since 2017 in Zinave NP, where the buffalo was extinct, with the reintroduction of 250 buffalo from Marromeu Reserve and surrounding trophy hunting areas (Mike La Grange, personal communication). A 2021 Zinave census reported 479 buffalo in the core sanctuary area (Antony Alexander, personal communication). Further south (Gaza Provinces), Banhine NP is estimated to host about 200 buffalo (Joao Simoes Almeida, personal communication). The Chicualacuala trophy hunting areas, located along Gonarezhou NP (Zimbabwe) also contain around 800 buffalo, but this figure is variable because the population undertakes seasonal migrations through Gonarezhou NP (Anthony Marx and Joao Simoes Almeida, personal communication). Finally, two areas adjacent to Kruger NP (South Africa) also host significant buffalo populations. The first is the Limpopo NP, with a population estimated around 5000 based on the 2018 census (Antony Alexander, personal communication). The second is the Great Lebombo Conservancy (including Sabie Game Park) with around 2000 buffalo (Joao Simoes Almeida, personal communication).
In the south-eastern tip of the country (Maputo Province), around 250 buffalo have been reintroduced in the Maputo Special Reserve since 2016 and their population is estimated at 300 (Antony Alexander, personal communication). Finally, around 50 individuals are present in Namaacha Catuane Community Area (close to the borders with Eswatini and South Africa; Joao Simoes Almeida, personal communication).
Buffalo are also present in numerous fazendas do bravio (private game ranches) and Coutadas (State-owned protected areas leased and managed by the private sector for hunting tourism). Most of these areas are unfenced, so nearly all buffalo in Mozambique are wild and free-ranging.
Overall, the buffalo has been experiencing a spectacular post-civil-war recovery in Mozambique since 1992, mainly by reintroductions where the species had become extinct, and by reinforcements of rump populations. In recent years, buffalo translocations have been conducted frequently in Mozambique. Some of the buffalo originate from South Africa, but most are indigenous, coming from trophy hunting areas within the Marromeu complex and the Niassa Special Reserve.
Namibia
Because the availability of perennial water is a key requirement for African buffalo, much of Namibia is not suitable for naturally occurring populations of Cape buffalo, except for the Caprivi Strip in the south and the area along the border with Angola in the north. As with probably all African buffalo populations, those in Namibia were drastically reduced during the 1890s rinderpest epidemic. Small herds survived along the perennial rivers of the far north-eastern Kavango East and Zambezi regions (Martin, Reference Martin2002). By 1934, their distribution had spread somewhat west and southwards to include what is now known as Kavango West, and small seasonal populations in Ohangwena, Omusati and Oshikoto regions (Shortridge, Reference Shortridge1934). Any further natural expansion was halted by the erection of a veterinary control fence in the 1960s to protect commercial cattle ranching from the central north southwards. The only exception to the present day has been the reintroduction of two isolated herds in the Waterberg Plateau Park and the Nyae-Nyae communal conservancy. In Waterberg, the founder population of 48 individuals were sourced gradually between 1981 and 1991 from the disease-free Addo Elephant NP population in South Africa at a rate of approximately four a year, while four animals were added to Waterberg from a zoo in then Czechoslovakia in 1986, and 11 from Willem Prinsloo Game Reserve in South Africa, also in 1986 (Martin, Reference Martin2008). The location of the herd on the plateau bordered by sandstone cliffs does not allow the buffalo to move from the plateau. In Nyae-Nyae, 30 individuals from a natural population in the area were fenced off in 1996. Only one individual tested positive for FMD, and was destroyed (Martin, Reference Martin2008). The Waterberg population has grown to at least 800 individuals, and the Nyae-Nyae population to about 250 head, both considered disease-free (Kenneth Uiseb, personal communication). The Zambezi and Kavango populations move freely into and from Angola and Botswana within the Kavango–Zambezi (KAZA) transboundary conservation area (Naidoo et al., Reference Naidoo, Preez, Stuart-Hill and Beytell2014). The current estimate in Namibia’s portion of KAZA is 7500 individuals based on a 2019 aerial census (Craig and Gibson, Reference Craig and Gibson2019). This represents a steady increase from 4500 in 2014 and 5500 in 2015 (Craig and Gibson, Reference Craig and Gibson2014, Reference Craig and Gibson2015).
South Africa
Cape buffalo were historically present throughout the country except for the arid western section. Free-ranging Cape buffalo were extirpated from their former range and are now totally confined within fenced areas (except Kruger NP along the Zimbabwe and Mozambique borders). At the end of the 1980s and beginning of the 1990s, the total number of buffalo in the country was about 50,000 head (East, Reference Dupuy1998). Based on the data collected, the present buffalo population stands at an estimated 121,000 heads, distributed between national parks (~40,000; 28 per cent), game parks (~26,000; 10 per cent) and privately owned game farms (~75,000; 62 per cent) (Chapter 14; Cornélis et al., Reference Cornélis, Melletti, Renaud, Fonteyn, Bonhotal, Prins, Chardonnet and Caron2023).
About 96 per cent of the national parks’ population is located in Kruger NP (~32,800; Ferreira et al., Reference Fay, Elkan, Marjan and Grossmann2021). The rest are located in the following national parks (Ferreira et al., Reference Fay, Elkan, Marjan and Grossmann2021): Addo (~450), Mokala (~500), Marakele (~250), Mountain Zebra (~90) and Camdeboo (~30). Populations in the parks are fairly constant despite population controlling factors such as bovine tuberculosis and the effects of droughts (see Chapter 8).
Several private game reserves (Sabi Sand, Klaserie, Thornybush, etc.) set alongside the unfenced western boundary of Kruger NP (the so-called ‘APNR’ – Association of Private Nature Reserves, 1800 km2 together with the NP named ‘Greater Kruger’) also host about 6000 buffalo (Mike Peel, personal communication). This complex as a whole (~21,000 km2) therefore hosts a population of approximately 58,000 buffalo. Further south in KwaZulu–Natal, Hluhluwe–Imfolozi Park (960 km2) carries about 6400 buffalo (Dave Druce, personal communication).
Buffalo in private care (privately owned game ranches) number about 75,000 individuals in 2022 (Peter Oberem, personal communication; Chapter 14). This population is distributed among 3144 game properties (2980 of which contain disease-free buffalo). About half (53 per cent) of the game ranches that hold buffalo are located in Limpopo Province, followed by North West Province (12 per cent) and Free State (11 per cent) (Figure 4.10). Many of these enclosed subpopulations are intensively managed (i.e., with intensive and selective breeding; Chapter 14); they are from an IUCN Red List assessment point of view supernumerary (cf. Tambling et al., Reference Tambling, Venter, Toit, Child, Child, Roxburgh, Do Linh San, Raimondo and Davies-Mostert2016), but some of the relatively untrammelled populations can still be of importance for restocking wilderness areas.
Zambia
Nowadays, the Cape buffalo in Zambia is largely confined to national parks and trophy hunting areas that in this country are named Game Management Areas (GMAs), with populations estimated around 40,000 in the late 1990s (East, Reference Dupuy1998). According to the latest available estimates, the buffalo population in this country has remained constant since then. The bulk of the population (~30,000) is located in the Luangwa Valley ecosystem (32,800 km2), mainly North and South Luangwa NPs and surrounding GMAs (DNPW, 2016). Important populations (~6400 over 45,000 km2) also occur in the Kafue ecosystem, mainly (97 per cent) in Kafue NP (Busanga Plains, Chunga and Ngoma Headquarters, and areas adjacent to Mufunta and Kasonso Busanga GMAs; DNPW, Reference Devers and Weghe2019). The Zambian section of the Lower Zambezi ecosystem hosts about 4800 buffalo over 2500 km2, with the bulk of the population (80 per cent) located in Chiawa GMA (DNPW, 2016). Several other areas in the country host populations of a few hundred animals. East of the Kafue ecosystem, Kafue Flats wetlands (5600 km2: Blue Lagoon and Lochinvar NPs Kafue Flats GMA) host about 900 head (Shanungu et al., Reference Shanungu, Kaumba and Beilfuss2015). West of the Luangwa Valley ecosystem, Bangweulu swamps host about 400 head (APN and DNPW, 2019). Finally, about 200 buffalo range in the Liuwa Plain NP and surrounding Upper West Zambezi GMA (APN and DNPW, 2020). To our knowledge, no recent information is available on the populations located between lakes Mweru and Tanganyika in the north of the country, but the total population appears to be constant.
Zimbabwe
Cape buffalo are now exclusively located in large protected area complexes in the northern (Northwest Matabeleland, Sebungwe region and Lower Zambezi Valley) and southern (South East Lowveld) sections of the country. During the period that covers the estimates of East (Reference Dupuy1998), the total number of buffalo was ~50,000. According to the most recent estimates, Zimbabwe currently hosts about 30,000–35,000 buffalo (Dunham et al., Reference Dunham, Mackie, Nyaguse and Zhuwau2015a, Reference Dunham, Mackie, Nyaguse and Zhuwau2015b, Reference Dunham, Mackie, Nyaguse and Zhuwau2015c; Cumming, Reference Cuisance2016; Dunham and van der Westhuizen, Reference Dunham and Westhuizen2018). The populations in the northern part of the country (about 15,000) are free-ranging in State-protected areas and communal land under the CAMPFIRE programme, while most southern populations (about 18,000) are fenced-in within State and private land (commercial conservancies). The northern region faced a severe decline over the last 20 years (from about 42,000 in 2001 (Dunham et al., Reference Dunham, Mackie, Nyaguse and Zhuwau2015a, Reference Dunham, Mackie, Nyaguse and Zhuwau2015b, Reference Dunham, Mackie, Nyaguse and Zhuwau2015c) to the present 15,000 head). In contrast, the numbers have been increasing in the southern section of the country. Three protected areas – Gonarezhou NP, Bubye Valley Conservancy and Save Valley Conservancy – host two-thirds of the southern populations. There, buffalo recovered well from the devastating effects of the drought of 1992 (see East, Reference Dupuy1998).
Trends in Abundance over the Last Decades
In this chapter, we have presented the most recent information available on the abundance and distribution of the African buffalo. We have also presented the trends observed where such information was available, usually at the local level. To do this, we drew on the published scientific literature, and collected and compiled a substantial amount of information from the grey literature (unpublished reports). We also contacted numerous organizations and field experts, to whom we express our sincere thanks (see below).
Although we believe that this synthesis is the most comprehensive to date, it is still not exhaustive. The puzzle remains especially incomplete in areas of ongoing or recent armed conflict, or in the large, often inaccessible areas of tropical forest where buffalo populations are small and diffuse. Consequently, the absence of buffalo sightings in a given area does not mean that buffalo are absent, but rather that no presence information was reported to us despite our investigations and many queries in our network (AFbIG members and others). Conversely, sightings of buffalo in previously unrecorded areas (e.g. Congo Basin) are simply the result of access to previously unavailable information.
Making temporal comparisons is also complex because few protected areas are monitored on a regular basis using robust and standardized approaches. Access to information is also a challenge. Although most often funded by public bodies and/or intended for public bodies, wildlife count reports are rarely published. Reports also rarely present disaggregated data, making temporal comparisons by area difficult (e.g. abundance inside versus outside protected areas).
Despite these limitations, a brief summary of the situation and regional trends is presented below.
Savanna Buffalo
The savanna buffalo population is estimated in 2022 at over 595,000 individuals, after deduction of the 75,000 buffalo under intensive private management in South Africa (q.v.; Table 4.1). Its abundance is roughly equivalent to that estimated 25 years ago (625,000) by East (Reference Dupuy1998). With an estimated population of over 540,000 individuals, the Cape buffalo is by far the most abundant subspecies (91 per cent of the total), far ahead of the West (>20,000; 3 per cent) and Central (>34,000; 6 per cent) African savanna buffalo; for the forest buffalo, we do not dare to make a numerical assessment.
Table 4.1 Abundance of the savanna subspecies of the African buffalo (three savanna subspecies: brachyceros, aequinoctialis and caffer) based on the most recent data available and comparison with earlier global assessments.
East (Reference Dupuy1998) | Cornélis et al. (Reference Cornélis, Melletti, Korte, Melletti and Burton2014) | Cornélis et al. (Reference Cornélis, Melletti, Renaud, Fonteyn, Bonhotal, Prins, Chardonnet and Caron2023) | |
---|---|---|---|
S. c. brachyceros | >20,000 | >17,000 | >20,500 |
Benin | >2000 | 4600 | 8200 |
Burkina Faso | 1600 | 5000 | 5300 |
Cameroon | 3200 | 4000 | 2500 |
Gambia | Ex | Ex | Ex |
Ghana | C | 700 | 1400 |
Guinea | V | X | X |
Guinea-Bissau | X | U | U |
Côte d’Ivoire | 8300 | 900 | 1500 |
Mali | 120 | Ex | Ex |
Niger | 500 | 1200 | 1100 |
Nigeria | >200 | >170 | X |
Senegal | >4000 | 460 | 500 |
Togo | U/R | X | X |
S. c. aequinoctialis | >59,000 | >23,000 | >34,000 |
Central African Republic | 19,000 | 4000 | >4000 |
Chad | 1000 | 8000 | 16,000 |
DRC | 39,000 | 6000 | 9400 |
Eritrea | Ex | Ex | Ex |
Ethiopia | X | 4000 | 5000 |
South Sudan | >100 | U | X |
Sudan | X | ||
S. c. caffer | >545,000 | >447,000 | >540,000 |
Angola | <500 | X | X |
Botswana | 27,000 | 40,000 | 29,000 |
Burundi | 500 | Uk | X |
DRC | No data | 2000 | 600 |
Ethiopia | 2300 | 3600 | 10,000 |
Kenya | >20,000 | >17,000 | 42,000 |
Malawi | >3000 | Uk | 3000 |
Mozambique | 10,000 | 23,000 | 45,000 |
Namibia | 1000 | 6000 | 9000 |
Rwanda | 1200 | R | 3500 |
Somalia | U | Uk | X |
South Africa | 28,500 (*) | 52,000 (**) | 46,000 (***) |
Eswatini | U | Uk | R |
Tanzania | >342,000 | >189,000 | >240,000 |
Uganda | >20,000 | 23,000 | 38,000 |
Zambia | >40,000 | >29,000 | 41,000 |
Zimbabwe | 50,000 | 63,000 | 33,000 |
Total | >625, 000 | >487,000 | 595,000 |
Legend: (C): Common; (Ex): Extinct; (R): rare; (U): uncommon; (Uk): unknown; (V): occurs only as a vagrant; (X): definitely present but abundance unknown; (*; **, ***): estimates excluding the 2500, 26,000 and 75,000 buffalo in game ranches/farms, respectively.
Tanzania is the country where the Cape buffalo is the most abundant, with an estimated population of over 240,000 individuals (44 per cent of the Cape buffalo subspecies), followed by South Africa (46,000), Mozambique (45,000), Kenya (42,000) and Zambia (41,000).
It is worth noting that four ecosystems contain more than 50,000 savanna buffalo. Three of these ‘5-star’ ecosystems are in Tanzania: the Serengeti Ecosystem (~69,000; ~24,000 km2), the Selous–Mikumi Ecosystem (~67,000; ~74,000 km2) and the complex composed by the adjacent Katavi–Rukwa and Ruaha–Rungwa Ecosystems (~53,000; ~83,000 km2). The other ‘5-star’ ecosystem is the Kavango–Zambezi Transfrontier Conservation Area (KAZA, southern Africa; ~52,000; ~520,000 km2).
Despite the apparent constancy of Cape buffalo abundance on a global scale, contrasts appear on a national scale (Table 4.1). A comparison with estimates made 25 years ago (East, Reference Dupuy1998) suggests that some national buffalo stocks have increased significantly, such as those of Namibia (+800 per cent), Mozambique (+350 per cent), Ethiopia (+335 per cent), Rwanda (+190 per cent), South Africa (+60 per cent) and Uganda (+90 per cent). In contrast, some national buffalo stocks have declined substantially, such as in Tanzania (–30 per cent) and Zimbabwe (–34 per cent). However, these trends should be treated with great caution given the biases associated with these estimates. In Ethiopia, for example, part of the increase in numbers is due to the discovery of buffalo outside their previously established distribution range.
The same observation applies to the West African savanna buffalo. Despite a population apparently similar to that estimated 25 years ago, some countries have witnessed an increase in population (Benin: 310 per cent; Burkina Faso: 224 per cent; Niger: 127 per cent) and others a decrease (Senegal: –87 per cent; Côte d’Ivoire: –82 per cent). The largest buffalo population is located in WAP Regional Park (28,350 km2), Benin, Burkina Faso, Niger. This complex comprises 3 National Parks (‘W’, Arly and Pendjari) and several neighbouring trophy hunting areas, with a buffalo population estimated at about 15,000 individuals (Bouché et al., Reference Bouché, Nzapa Mbeti Mange and Tankalet2015; Ouindeyama et al., Reference Ouindeyama, Chevillot and Akpona2021). Secondary strongholds are located in Cameroon (Bouba Ndjidda–Bénoué–Faro NP and neighbouring trophy hunting areas, ~2500), Ghana (Mole NP, ~1400), Côte d’Ivoire (Comoé NP, ~1200) and Senegal (Niokolo–Koba NP, ~500) (see country sections for details).
Finally, the most worrying situation is probably that of the Central African savanna buffalo, which has nearly halved in abundance over the last 25 years. The collapse of the population in the Central African Republic (–80 per cent) has only been partially offset by the increase (albeit spectacular: +1600 per cent) in Chad (Zakouma NP), and to a lesser but promising extent by the recent recovery of populations in DRC (Garamba NP). Today, half of the residual population of this subspecies is located in a single protected area (Zakouma NP).
Forest Buffalo
As pointed out above, estimating the abundance of forest buffalo is challenging, not to mention ascertaining a trend. Indeed, in dense tropical forest, populations are spatially dispersed, in small herds, in very dense habitats, and are distributed over a very large geographical area.
In the residual forest block of West Africa, we obtained very little information on the presence of the forest buffalo. The forest buffalo is restricted to limited and isolated patches of forest with small populations. In this circumstance, it is likely that forest buffalo in West Africa might be decreasing in much of its distribution range due to a combination of poaching for bushmeat trade, habitat loss and degradation.
In Central Africa, our investigations have shown that the forest buffalo is still well represented in areas with low human density, from the Atlantic coast to south-east Cameroon and up to the border of CAR and Republic of Congo, both in protected areas and adjoining logging and hunting concessions. Of the 235 locations of forest buffalo that we have collected in Central Africa during our review, 45 per cent are located within the Greater TRIDOM-TNS, a vast contiguous block of mainly intact moist forest covering 250,000 km2 (11 per cent of Central African forest block) and straddling four countries (Cameroon, Central African Republic, Gabon and Republic of Congo; European Commission, Reference Erena and Bekele2015). This vast area is thus probably the most strategic stronghold for the conservation of the forest buffalo in Central Africa.
Conservation Status, Challenges and Opportunities
The latest 10-year update of the conservation status of the African buffalo has led to its downgrading from ‘Least concern’ to ‘Near threatened’ (IUCN SSC Antelope Specialist Group, 2008, 2019). The African buffalo is therefore now placed at level 2 on a seven-step threat risk scale. As we have just seen, this global conservation status masks significant regional disparities that result from different combinations of environmental and human factors. In the following sections, we look at the main drivers of these contrasted trajectories. As both the West and Central Africa buffalo are globally confronted with similar factors (and are also very close from a genetic and phenotypic perspective, see Chapter 3), we have grouped them together in a single section called ‘northern savanna buffalo’.
Northern African Savanna Buffalo (S. c. brachyceros and S.c. aequinoctialis)
The current distribution area of the northern African savanna buffalo is very fragmented and most populations are located within a few protected areas. This situation is the consequence of a strong anthropic pressure, in a context where poor soils limit biomass production (Chapter 5). The near extinction of the African buffalo in Nigeria, the most densely populated country in West Africa (Figure 4.11), is the culmination of similar processes taking place progressively throughout the region.
The protected areas in the savannas of West and Central Africa are particularly challenged by the increasing expansion of cash crops (e.g. cotton, groundnut) at their periphery, but also by the expansion of livestock husbandry. The massive movements of livestock in the immediate periphery and within protected areas has been a recurrent and growing problem in recent decades (Bouché et al., Reference Bouché, Lungren, Hien and Omondi2012; Aebischer et al., Reference Aebischer, Ibrahim and Hickisch2020). The increasing effective control of sleeping sickness (African trypanosomiasis) has facilitated the rising number of livestock (Gouteux et al., Reference Gluszek, Viollaz and Gore1994; Cuisance, Reference Cuisance1996; Reid et al., Reference Reid, Kruska and Deichmann2000; Courtin et al., Reference Courtin, Rayaissé and Tamboura2010), the geographical range of which was previously greatly reduced to the benefit of trypano-tolerant wild species such as the African buffalo (Chapter 9).
Against this backdrop, the conservation of wildlife in general and African buffalo in particular is highly dependent on effective governance and management systems. Unfortunately, in a global context of poverty, poor governance, insecurity, corruption and centralized management, most protected areas in West and Central Africa lack public and private investment (Scholte, Reference Scholte2022). This situation is amplified by the fact that protected area networks are globally oversized in Central Africa, which holds the world record for the highest number of protected areas over 10,000 km². For example, the complex of protected areas in the north of Central African Republic (Manovo-Gounda St Floris, Bamingui Bangoran and adjacent gazetted Trophy Hunting Areas) covers 42,000 km², which represents half of the size of the region (80,000 km²). Such conservation overstretches, when combined with one of the highest poverty levels in the world, poorly developed infrastructure and massive and regular insecurity outbreaks, makes the conservation dilemma acute in Central Africa. In this context, it is estimated that West and Central African protected areas operate with 10 per cent of the resources needed for their sustainable management (Scholte, Reference Scholte2022).
Despite this bleak picture, targeted conservation efforts have managed to stabilize or even substantially increase buffalo populations in a few protected areas, today considered strongholds for biodiversity conservation in West and Central Africa. Over the last few decades, these protected areas have benefited from the support of public donors (such as the European Union or USAID). This support is now amplified by private investments in the form of public–private partnerships in the long term. The most striking example is the non-governmental organization (NGO) African Parks, which is successfully committed in long-term public–private conservation partnerships with governments in several countries such in Benin (Pendjari and W NPs), DRC (Garamba NP), Chad (Zakouma NP, Siniaka Minia Faunal Reserve and Aouk Aoukalé Faunal Reserve) and Central African Republic (Chinko Wildlife Refuge and Vovodo Hunting Area). It is also worth noting that protected areas organized in well-connected complexes with mixed management regimes (such as National Parks buffered and interconnected by functional trophy hunting areas) also tend to have stable buffalo populations (e.g. W–Arly–Pendjari complex or Bouba Ndjidda–Benoue–Faro complex).
So far, the strategy of focusing such conservation efforts over time on a few strongholds while waiting for better days is paying off. In this context, supporting sustainably a few more relevant strongholds (in Senegal, Cameroon, South Sudan, Central African Republic or Ethiopia) would help secure northern savanna buffalo populations, and in turn, a representative sample of ecosystems and species in this subregion. Once political stability is restored, regaining lost space around strongholds by diversifying conservation models (hopefully more participatory and inclusive) and restoring full sovereignty to national administrations seems the most realistic option to target (Scholte et al., Reference Scholte, Pays and Adam2022).
Forest Buffalo (S. c. nanus)
In West Africa, habitat loss and degradation are major threats to forest buffalo. The Guinean forests that run from Sierra Leone to Cameroon cover approximately 93,000 km² of natural vegetation, which represents only 15 per cent of its original cover (Mittermeier et al., Reference Mittermeier, Gil and Hoffmann2005; Aleman et al., Reference Aleman, Jarzyna and Staver2018).
The situation is less critical in Central Africa, which has historically been less disturbed, and where less than 9 per cent of the rainforest area has been lost since 2000 (Dalimier et al., Reference Dahourou and Belemsobgo2022). However, since 2009, the annual rate of forest degradation has increased in all Central African countries. If the rate of forest degradation observed over the past 10 years continues, the Democratic Republic of Congo could lose 33 per cent of its undisturbed rainforest by 2050 (Vancutsem et al., Reference Vancutsem, Achard and Pekel2020) as a result of agricultural expansion (Perrings and Halkos, Reference Perrings and Halkos2015), infrastructure development and extractive industries (Malhi et al., Reference Malhi, Adu-Bredu and Asare2013).
Hunting for wild meat or bushmeat is also a threat to forest buffalo in West and Central Africa, where many rural populations depend on wildlife for meat (van Vliet et al., Reference Van Vliet, Cornélis, Beck, Mateo, Arroyo and Garcia2016). Although poorly assessed, subsistence hunting and poaching are likely to have a strong impact on the forest buffalo insofar as the larger (and thus more profitable) species are generally the most sought after, especially when sold in big city markets. For example, buffalo meat was reported to be among the most expensive meat among ungulates in Bangui (Central African Republic; Fargeot et al., Reference Fagotto2017) as well as in Kinshasa (DRC) and Brazzaville (Republic of the Congo; Gluszek et al., Reference Gluszek, Viollaz and Gore2021).
Insecurity and the presence of armed groups are known to greatly amplify the pressure on forest buffalo, especially because military weapons are more suitable for hunting buffalo than traditional 12-gauge guns. For example, poaching by armed groups during the periods of rebellion in Yangambi landscape (DRC) led to the complete extirpation of buffalo (van Vliet et al., Reference Van Vliet, Muhindo and Kambale Nyumu2018).
In West Africa, our investigations show that the forest buffalo are clearly dependent on conservation efforts (protected areas and wildlife laws) to prevent extinction. The lack of effective conservation measures currently leaves forest buffalo critically endangered. In contrast, the conservation status of the forest buffalo is less of a concern in Central Africa as it benefits from better preserved habitats and less anthropogenic pressure, particularly West of the Congo basin. Several protected areas also benefit from long term public–private partnerships such as Nouabalé–Ndoki and Odzala NPs in the Republic of Congo, or Salonga NP in the Democratic Republic of Congo (Scholte, Reference Scholte2022).
Finally, although logging is often detrimental to wildlife because of easier access for poaching (Kleinschroth at al., Reference Kleinschroth, Laporte and Laurance2019), it is likely that a moderate opening of the forest canopy allows the forest buffalo to access more profitable food resources, as shown for large primates (Bekhuis et al., Reference Bekhuis, de Jong and Prins2008; Haurez et al., Reference Grossmann, Lopes Pereira and Chambal2014). In this context, the developing forest certification in Central Africa opens up interesting conservation perspectives for wildlife in general and for forest buffalo populations in particular.
Cape Buffalo (S. c. caffer)
Our investigations show that the conservation status of the African buffalo remains satisfactory in most of the countries in its geographical range. However, with a few exceptions, the Cape buffalo is now mainly confined to protected areas. Despite increasing human pressure, the integrity of protected areas is better respected in Eastern and Southern Africa, where conflicts and insecurity are less prevalent than in Western and Central Africa. The Cape buffalo populations also globally benefit from better soils conditions, particularly in East Africa where volcanic soils provide more profitable forage (Chapter 5). However, eastern and southern Africa are subject to severe droughts that have repeatedly affected buffalo populations in recent decades (Prins and Sinclair, Reference Prins, Sinclair, Knight, Kingdon and Hoffmann2013; Cornélis et al., Reference Cornélis, Melletti, Korte, Melletti and Burton2014). In a context where the frequency and amplitude of these events could increase in the near future due to climate change, buffalo populations – a water-dependent species – could be strongly and durably affected in Eastern and southern Africa and beyond (Sintayehu, Reference Sintayehu2018).
The good conservation status of the fauna in general and the buffalo in particular has made it possible for several countries to develop a thriving industry based on nature tourism (viewing and hunting; Chapter 16). In addition, the successful development of community-based natural resources management (CBNRM) programmes since about 25 years in several countries of the Cape buffalo range is to some extent responsible for the rather good conservation status of the subspecies in the two regions, for example the CAMPFIRE programme in Zimbabwe; Game Management Areas in Zambia; communal conservancies in Namibia; and Wildlife Management Areas in Tanzania. The role of Trophy Hunting Areas is often overlooked in the success of wildlife management, especially buffalo. The private ownership of buffalo in game ranches and the private management of buffalo in trophy hunting areas are powerful drivers of thriving buffalo populations (Chapter 16). Many National Parks of the Eastern and southern regions are embedded in networks of Trophy Hunting Areas (another category of Protected Areas) functioning as buffer zones and socioecological corridors between National Parks. Such complexes make much stronger conservation tools than isolated National Parks. Within this context, mass translocation has become a common tool for wildlife management in southern Africa for either reintroduction of the species or reinforcement of small populations. A striking example is Mozambique, where large buffalo herds thriving in trophy hunting areas within the Marromeu complex and Niassa Special Reserve were translocated to Gilé and Zinave National Parks.