Recently, aquaculture industry is developing rapidly in the worldwide to meet the increasing demand for human consumption(Reference Xu, Tan and Kong1). Fish muscle constitutes the major edible portion of body(Reference Mommsen2), in which growth is involved in muscle fibre’s hyperplasia and hypertrophy(Reference Zhao, Xia and Zhang3). Emerging evidences showed indispensable amino acids (threonine and leucine) played a vital role in fish muscle growth(Reference Zhao, Jiang and Zhou4,Reference Zhao, Li and Jiang5) . As the building blocks of protein, tryptophan (Trp) is the basic nutrient in the aquafeeds formulation(Reference Ramos-Pinto, Martos-Sitcha and Reis6). Previous studies have reported that dietary Trp could improve fish growth and feed efficiency(Reference Mardones, Devia and Labbé7,Reference Zhang, Dong and Wang8) . However, the information concerning the effects of Trp on muscle growth has not been extensively investigated in fish.
Muscle growth is one of the most dynamic and plastic processes, which is regulated by several of genetic factors involved insulin-like growth factors (IGF), myogenic regulatory factors (MRF) and myostatin (MSTN)(Reference Aguiar, Vechetti-Júnior and De Souza9). IGF, being the main regulators of muscle growth in vertebrates(Reference Zanou and Gailly10), directly stimulate muscle cell proliferation, differentiation and hypertrophy(Reference Fuentes, Valdés and Molina11). MRF, such as myf5, MyoD, MyoG and MRF4, belong to basic helix-loop-helix transcription factors that regulate myogenesis(Reference Perry and Rudnick12). The myf5 and MyoD are essential for muscle lineage determination and cell proliferation, while MyoG and MRF4 contribute to the differentiation programme’s initiation and maintenance(Reference Vélez, Lutfi and Jiménezamilburu13). The MSTN is a member of the TGF-β superfamily responsible for the negative regulation of muscle growth(Reference Lee and Mcpherron14). A recent study reported that Trp promoted proliferation and differentiation by increasing MyoD and MyoG mRNA expressions in mice myoblasts(Reference Dukes, Davis and El15). Despite increased understanding fish muscle grow regulation by some of these factors(Reference Fuentes, Valdés and Molina11), there are few pieces of researches about the effect of Trp on IGF, MRF and MSTN in muscle.
Muscle growth is also primarily related to the deposition of muscle protein, which is primarily attributed to increasing protein synthesis(Reference Toth, Matthews and Tracy16). In fish, IGF-I and IGF-II mediate their functions by binding to its specific receptor (IGF-IR)(Reference Fuentes, Björnsson and Valdés17). Then, IGF-IR activates the phosphoinositide 3-kinase (PIK3Ca)/protein kinase B (AKT) signalling pathway, which induces a phosphorylation cascade resulting in protein synthesis increase(Reference Schiaffino and Mammucari18). As the downstream component of the PIK3Ca/AKT signalling pathway, the target of rapamycin (TOR) plays a crucial role in protein synthesis via regulating the phosphorylation of its downstream effectors ribosomal S6 kinase 1 (S6K1) and translation initiation factor 4E-binding protein 1(Reference Bodine, Stitt and Gonzalez19,Reference Jewell, Kim and Russell20) . Recent studies in fish reported that dietary methionine and leucine stimulated muscle protein synthesis by regulating some of these factors(Reference Zhao, Li and Jiang5,Reference Alami-Durante, Bazin and Cluzeaud21,Reference He, Chi and Tan22) . A previous report in piglet showed that dietary Trp deficiency decreased muscle protein synthesis rates(Reference Cortamira, Seve and Lebreton23). Newer studies in grass carp and mice muscle showed that Trp could improve muscle mass by up-regulating TOR and S6K1 mRNA levels(Reference Dukes, Davis and El15,Reference Jiang, Wen and Liu24) . However, there is little available information regarding the effects of Trp on muscle growth and IGF/PIK3Ca/AKT1/TOR/S6K1 signalling pathway in fish.
In the lifespan of fish, muscle is the main metabolic organ, easily suffering a high risk of oxidative stress(Reference Wen, Jiang and Feng25,Reference Martínez-Álvarez, Morales and Sanz26) . To defend against oxidative stress, fish develops the antioxidant system, which includes antioxidant enzymes (such as superoxide dismutase (SOD), catalase (CAT), glutathione peroxidase (GPx), glutathione reductase (GR), glutathione-S-transferase (GST)), and non-enzymatic defense (glutathione (GSH))(Reference Wen, Jiang and Feng25). It has been reported that transcriptional regulation of a variety of antioxidant genes was predominantly mediated by the Keap1 (kelch-like ECH-associated protein (1)/Nrf2 (NF-E2-related factor (2) signalling pathway in fish(Reference Giuliani and Regoli27). Available studies showed that dietary Trp could improve fish antioxidant capacity by regulating the Keap1/Nrf2 signalling pathway(Reference Jiang, Wen and Liu24,Reference Ji, Liang and Ren28,Reference Wen, Feng and Jiang29) . Moreover, muscle growth was also regulated by the Keap1/Nrf2 signalling pathway(Reference Murakami and Motohashi30,Reference Al-Sawaf, Fragoulis and Rosen31) . In C2C12 myoblasts, the GSH depletion could affect the MyoD transition(Reference Ardite, Barbera and Roca32). These researchers suggested that the antioxidant system might have positive effects on muscle growth. However, to date, the information about the effects of dietary Trp on muscle growth associated with the antioxidant capacity have not been clearly elucidated.
Hybrid catfish Pelteobagrus vachelli♀ × Leiocassis longirostris♂ has been widely farmed in China recently(Reference Zhao, Wu and Xu33). The present study is the part of a larger study and used the same growth trial as our previous study, which reported that dietary Trp could significantly improve growth performance and intestinal antioxidant capacity of hybrid catfish(Reference Zhao, Wu and Xu33). Therefore, the present study was performed to investigate the effects of dietary Trp on muscle growth, protein synthesis, and antioxidant capacity.
Materials and methods
All experimental protocols were approved by Animal Care Advisory Committee of Sichuan Agricultural University (DKY-B20141508).
Experimental diets and designs
Ingredients and proximate composition of the experimental diets were the same as our previous study(Reference Zhao, Wu and Xu33), as shown in Supplementary Table S1. Dietary amino acid profiles were presented in Supplementary Table S2. Briefly, dietary protein sources were supplied with soyabean meal, rapeseed meal, maize gluten meal and fish meal. Dietary carbohydrates and lipid sources were, respectively, supplied with maize starch and soyabean oil. Six experimental diets were formulated containing graded levels of Trp (2·6 (control), 3·1, 3·7, 4·2, 4·7, and 5·6 g Trp/kg diet). All diets were maintained isonitrogenous by supplementation of l-glycine. The diets were formulated to contain about 41·20 % crude protein and 6·50 % crude lipid. The ingredients were ground into powder through a 60-mesh sieve and then mixed the dry ingredients with the oil. Then, each diet was extruded in a twin-screw extruder (MY-165) with a 2-mm die. The processing conditions were as follows: 100 rpm screw speed, 127°C temperature, and 30–45 atm pressure. Floating extruded pellets were air-dried and stored at –20°C in plastic bags until being used.
Feeding trial
The feeding trial was the same as in our previous study(Reference Zhao, Wu and Xu33). Hybrid catfish were obtained from Rong Sen Corporation (Sichuan, China). Before the feeding trial, fish were reared in experimental conditions for 4 weeks. Then, 864 healthy hybrid catfish with an average initial weight of 21·82 (sem 0·14) g were randomly divided into eighteen tanks (2·00 m × 1·00 m × 1·05 m), resulting in forty-eight fish in each tank. Fish were fed with their respective diets to apparent satiation two times (08.00, 18.00) per day for 8 weeks. During the experimental period, water temperature was 25 (sem 3°C), dissolved oxygen > 5·0 mg/l, pH 7·0(sem 0·5), NH4 +-N < 0·50 mg/l and NO2-N < 0·05 mg/l. The feeding trial was completed under natural light and dark cycle.
Sample collection
At the end of the feeding trial, fish were starved for 24 h to evacuate the alimentary tract contents before sampling. Twelve fish were randomly collected from each tank and were anaesthetised in a benzocaine (50 mg/l)(Reference Jiang, Wu and Zhou34). Then six fish were slaughtered, muscle samples from the left side of 6 fish each tank were quickly obtained, frozen in liquid N2 and stored at –80°C until analysis according to Bohne et al.(Reference Bohne, Hamre and Arukwe35). Meanwhile, the muscle samples from the same fish’s right side were taken with a scalpel rapidly, fixed and preserved in the Bonn solution, and then made into paraffin sections. Six fish from each tank were dissected, and the muscle samples were obtained and stored at –20°C for biochemical analysis.
Biochemical analysis
The composition of muscle was measured according to the methods described previously(Reference Jiang, Wen and Liu24). In brief, moisture content was measured by drying the samples to a constant weight at 105°C in a drying oven. Crude protein content (n × 6·25) was estimated using the Kjeldahl system method. Crude lipid and ash contents were analysed, respectively, by Soxhlet extraction system and combusting samples in a muffle furnace at 550°C for 12 h.
The muscle samples used to determine enzyme activities, MDA and PC contents were each homogenised in 10 volumes (mg/ml) of ice-cold physiological saline solution and centrifuged at 6000 g for 20 min at 4°C. The supernatant was collected for analysis. Contents of MDA, PC and GSH were determined by Jiang et al. (Reference Jiang, Liu and Jiang36). The T-SOD, CAT, GPx, GST, GR, ASA, and AHR activities were determined according to the procedures described by our previous study(Reference Jiang, Feng and Liu37).
Analysis of muscle fibre number and cross-sectional area
Transverse white muscle (n 6 fish per treatment) sections (5 μm) were paraffin embedded and subjected to haematoxylin-eosin (H&E) staining as described to evaluate muscle morphology(Reference Dogra, Changotra and Wergedal38). Stained sections were visualised under an optic microscope and images were captured by a DS-Ril camera and software. Muscle fibre number and diameter were measured for about 300 adjacent muscle fibres in each fish section using Image Pro 6·0 software. White muscle fibres were distributed into three diameter classes (≤ 20, 20–50 or > 50 μm)(Reference de Almeida, Carvalho and Pinhal39). Muscle fibre density was calculated by measuring at least 900 muscle fibres for each fish (20×). Muscle fibre frequency was expressed as the number of fibres from per diameter class relative to the total number of fibres measured.
RNA extraction and quantitative real-time PCR
The procedures of RNA isolation, reverse transcription and quantitative real-time PCR were similar to those previously described in another study conducted in our laboratory(Reference Jiang, Wu and Zhou40). Total RNA was isolated from the muscle using RNAiso Plus kit (TaKaRa) according to the manufacturer’s protocol followed by DNAse I treatment. Samples were subjected to electrophoresis on 1 % agarose gels to confirm the integrity of RNA, and RNA concentration was assessed by the OD 260/280 and OD260/230 absorbance ratio. Subsequently, the 2 μg of total RNA was used to reverse transcribed into cDNA using the PrimeScript™ RT reagent Kit (TaKaRa) according to the manufacturer’s instructions. Specific primers were designed according to sequences of hybrid catfish cloned in our laboratory and the published sequences of hybrid catfish (online Supplementary Table S3). All of the real-time PCR analyses were performed in a CFX96 Real-Time PCR Detection System (Bio-Rad). The PCR mixture consisted of l μl of the first-strand cDNA sample, 0·5 μl each of forward and reverse primers from 10 μM stocks, 3 μl RNase free dH2O and 5 μl 2 × Ssofast EvaGreen Supermix (Bio-Rad). Cycling conditions were 98°C for 2 min, followed by 40 cycles of 98°C for 5 s, annealing at a different temperature for each gene for 10 s, and 72°C for 15 s. Melting curve analysis was generated following each real-time quantitative PCR assay to check and ensure the specificity and purity of all PCR products. Each sample was amplified in triplicate. Target gene mRNA concentration was normalised to the mRNA concentration of the reference genes β-actin and 18S rRNA and detailed methods refer to Vandesompele et al. (Reference Vandesompele, De and Pattyn41). The target and housekeeping gene amplification efficiency were calculated according to the specific gene standard curves generated from 10-fold serial dilutions, and all the efficiency of the primers approached 100 %. The expression results were calculated using the 2–ΔΔCt method, according to Livak et al. (Reference Livak and Schmittgen42).
Western blot analysis
Muscle tissues were homogenised in liquid N2 for protein extraction, and the protein abundance was determined with the western blot. Briefly, after the extraction of muscle, the total protein concentrations were determined using the BCA protein assay kit (Beyotime). The 40 μg protein was separated by SDS-PAGE and transferred to a PVDF membrane. The membrane was blocked for 1 h at room temperature and then incubated with primary antibody overnight at 4°C. The primary antibodies used in this study were protein kinase B (AKT) (1:1000; total and Ser473, Cell Signalling Technology Inc), TOR (1:2000; total and Ser2448, CST), S6K1 (1:2000; total and Thr389, CST), Nrf2 and Keap1 (1:2000, Zen Biotechnology). β-actin (1:1000; CST) and Lamin B1 (1:2000, Zen Biotechnology) were used as control protein for total and nuclear protein, respectively. The blots were washed five times for 3 min each, followed by 1 h incubation with appropriate secondary antibody in Tris Buffered Saline Tween 20 at room temperature. The blots were visualised using an ECL kit (Beyotime) according to the manufacturer’s instructions. This experiment was repeated at least three times and similar results were obtained each time.
Nuclear protein extraction and electrophoretic mobility shift assay
Nuclear protein was extracted from the muscle as described previously(Reference Jiang, Liu and Jiang36). The binding activity of Nrf2 was measured using a chemiluminescent electrophoretic mobility shift assay kit (Beyotime) according to the manufacturer’s protocol. The nuclear proteins were used for electrophoretic mobility shift assay after determining the protein concentration using the BCA protein assay kit. The sequence of the biotin-labelled oligonucleotide probes for ARE (Beyotime) was as follows: 5′-ACT GAG GGT GAC TCA GCA AAA TC-3′, 3′-TGA CTC CCA CTG AGT CGT TTT AG-5′ containing the acknowledged Nrf2 binding site. Then, labelled oligonucleotides were incubated in a reaction mixture that contained 2 μg of nuclear protein and DNA-binding buffer for 20 min. The reaction mixture was subsequently loaded in a 5 % non-denaturing PAGE, and the DNA/protein complexes were then transferred to a nylon membrane. Detection was performed using the ECL kit’s instructions (Beyotime). This experiment was repeated three times with similar results achieved.
Statistical analysis
All results were presented as means with their standard error of means. Data were subjected to a one-way ANOVA by Duncan’s multiple range tests to determine significant differences among the treatments at P < 0·05 with SPSS statistics version 20.0 (SPSS Inc.). A quadratic regression model was used to determine the optimal level of dietary Trp, according to Song et al. (Reference Song, Jiang and Liu43).
Results
Muscle composition, fibre density and morphometric analyses
Fish survival rate (>97 %) showed no differences among dietary Trp levels. Higher protein content of muscle was observed in fish fed 3·7 and 4·2 g Trp/kg diet compared with others (Table 1, P < 0·05). The lipid and ash contents were all significantly increased with dietary Trp levels up to 4·2 g Trp/kg diet, and thereafter declined (Table 1). Nevertheless, muscle moisture was not affected by dietary Trp levels (P > 0·05). Muscle fibre density increased with increasing dietary Trp levels up to 4·2 g/kg diet and decreased thereafter (P < 0·05, Fig. 1). The muscle fibre frequency distribution of the diameters within each treatment is presented in Table 2. The frequency distribution of ≤ 20 μm diameter muscle fibres in fish fed 4·2 g Trp/kg diet was higher than that in the other all groups (P < 0·05). There was a decreased muscle fibre frequency in class 20–50 μm with increasing dietary Trp levels up to 4·2 g Trp/kg diet (P < 0·05). In class > 50 μm, fish fed 4·2 g Trp/kg diet showed higher muscle fibre frequency than those fed others (P < 0·05).
Table 1. The muscle composition (%, wet-basis) of hybrid catfish fed diets with graded levels of Trp for 56 d
(Mean values and standard errors of the mean)
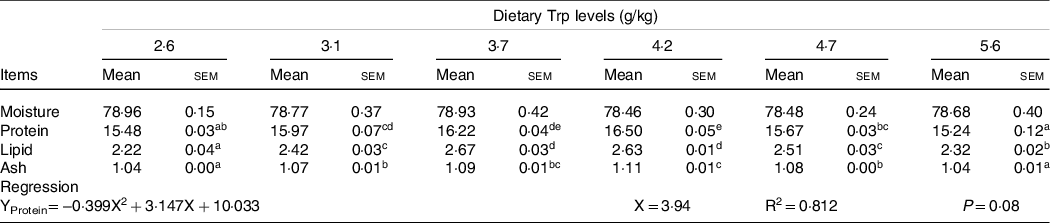
Three replicate groups with six fish in each group. Values within the same rows having different superscripts are significantly different (P < 0·05).
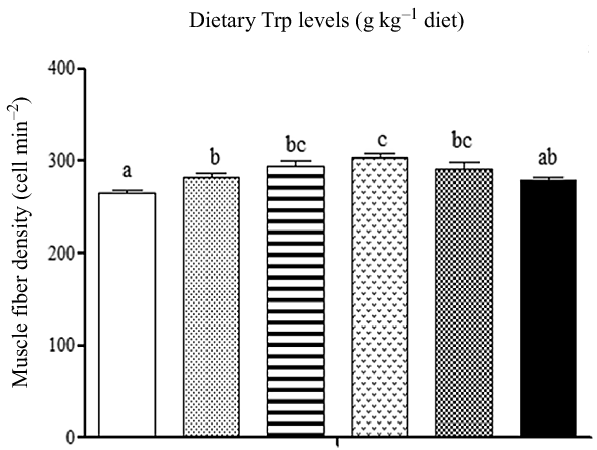
Fig. 1. The muscle fibre density (cell mm–2) of hybrid catfish Pelteobagrus Vachelli♀ × Leiocassis longirostris♂ fed diets containing graded levels of Trp (g/kg). Data represent means with their standard error of means, of three replicates. Values having different letters are significantly different (P < 0·05). , 2·6;
, 3·1;
, 3·7;
, 4·2;
, 4·7;
, 5·6.
Table 2. The muscle fibres cellularity (%, frequency) of hybrid catfish fed diets with graded levels of Trp for 56 d
(Mean values and standard errors of the mean)

Three replicate groups with three fish in each group; within each row, means not sharing a common superscript letter are significantly different (P < 0·05). d, diameter of muscle fibres.
Expression of muscle proliferation and differentiation-related genes
Effect of dietary Trp on the mRNA levels of genes involved in proliferation and differentiation of muscle is shown in Fig. 2. The PCNA mRNA level was the highest for fish fed 4·2 g Trp/kg diet (P < 0·05), and there was no significant difference among other groups. The myf5 mRNA level was the lowest in fish fed 5·6 g Trp/kg diet and highest in fish fed 4·7 g Trp/kg diet (P < 0·05). Moreover, fish fed 5·6 g Trp/kg diet showed lower MyoD1, MyoG and MRF4 mRNA levels than those fed others (P < 0·05). No notable differences were observed in different dietary Trp levels for the MyHC mRNA level. Fish fed 3·1 and 3·7 g Trp/kg diets had lower MSTN mRNA level than those fed other diet groups (P < 0·05).
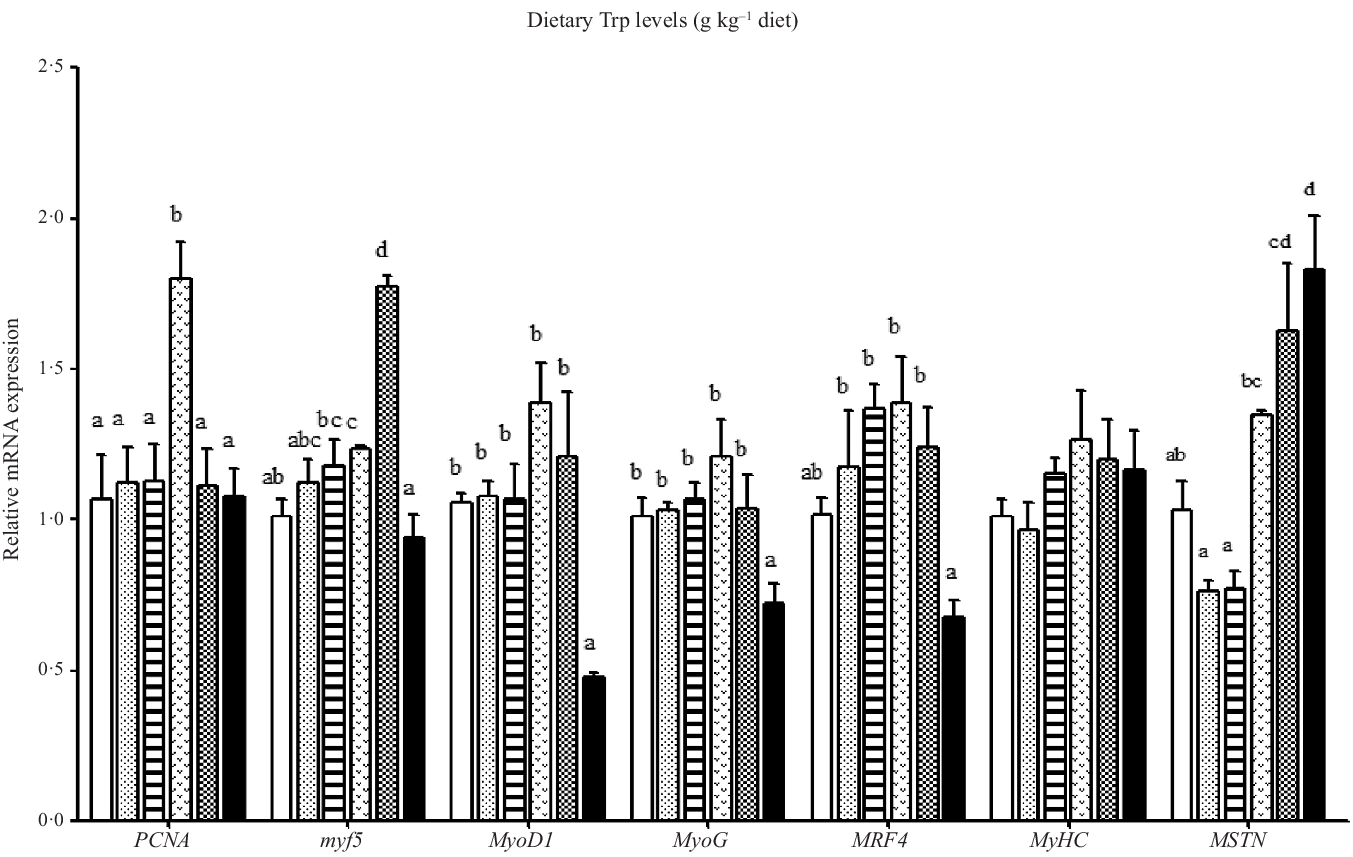
Fig. 2. Relative expression of PCNA, MRF, MyHC and MSTN genes in the muscle of hybrid catfish Pelteobagrus Vachelli♀ × Leiocassis longirostris♂ fed diets containing graded levels of Trp (g/kg). Data represent means with their standard error of means, of six replicates. Values having different letters are significantly different (P < 0·05). , 2·6;
, 3·1;
, 3·7;
, 4·2;
, 4·7;
, 5·6.
AKT-TOR signalling-related genes and proteins expression
As shown in Figs. 3 and 4, the mRNA levels of IGF-I and IGF-IR were gradually increased with increasing dietary Trp levels up 4·2 g/kg diet and decreased thereafter (P < 0·05). The IGF-II mRNA level was higher in fish fed 4·2 and 4·7 g Trp/kg diets than those fed other diet groups (P < 0·05). The PIK3Ca, TOR and S6K1 mRNA levels were significantly up-regulated with dietary Trp levels increased up to 4·2 g/kg diet and decreased thereafter. Differently, the mRNA level of AKT1 was not affected by dietary Trp (P > 0·05). The 4EBP1 mRNA level was decreased with increasing dietary Trp levels up to 4·2 g/kg diet and subsequently increased (P < 0·05).

Fig. 3. Relative expression of IGF-I, IGF-II and IGF-IR genes in the muscle of hybrid catfish Pelteobagrus Vachelli♀ × Leiocassis longirostris♂ fed diets containing graded levels of Trp (g/kg). Data represent means with their standard error of means, of six replicates. The different letters indicate significant differences among groups (P < 0·05). , 2·6;
, 3·1;
, 3·7;
, 4·2;
, 4·7;
, 5·6.

Fig. 4. Relative expression of PIK3Ca, AKT1, TOR, 4EBP1 and S6K1 genes in the muscle of hybrid catfish Pelteobagrus Vachelli♀ × Leiocassis longirostris♂ fed diets containing graded levels of Trp (g/kg). Data represent means with their standard error of means, of six replicates. Values having different letters are significantly different (P < 0·05). , 2·6;
, 3·1;
, 3·7;
, 4·2;
, 4·7;
, 5·6.
The protein levels of AKT, TOR and S6K1 in the muscle analysed with western blot are displayed in Fig. 5. The protein levels of p-AKT (Fig. 5(a)), p-TOR (Fig. 5(b)) and p-S6K1 (Fig. 5(c)) in the muscle were enhanced (P < 0·05) in fish fed with 4·2 g Trp/kg diet compared with those fed the 2·6 and 5·6 g Trp/kg diets.
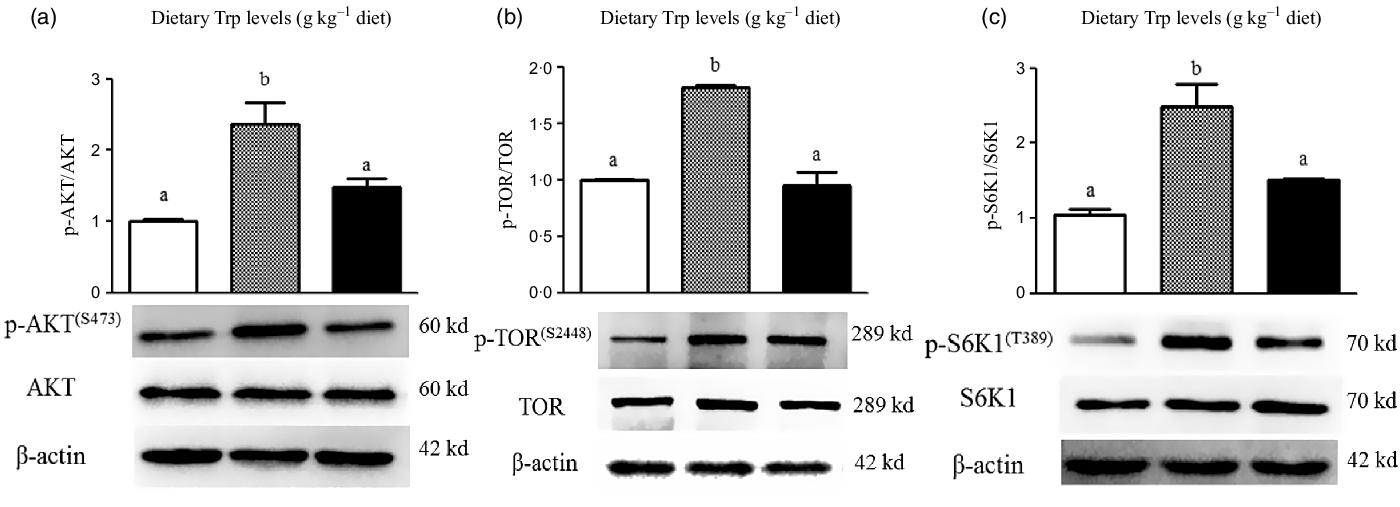
Fig. 5. Dietary supplementation with Trp activated the AKT-TOR signalling pathway in the muscle of hybrid catfish Pelteobagrus Vachelli♀ × Leiocassis longirostris♂. Protein abundances of p-AKT (, 2·6;
, 4·2;
, 5·6) (a), p-TOR (
, 2·6;
, 4·2;
, 5·6) (b) and p-S6K1(
, 2·6;
, 4·2;
, 5·6) (c) were determined. Data represent means with their standard error of means, of three replicates. Values having different letters are significantly different (P < 0·05).
Antioxidant-related parameters
The effects of dietary Trp on muscle antioxidant-related parameters are presented in Table 3. The MDA and PC contents were significantly decreased with increasing dietary Trp levels up to 4·2 g Trp/kg diet and then increased. No significant difference in T-SOD and GPx activities among treatments was detected. The CAT and ASA activities were increased with increasing dietary Trp levels up to 4·2 g Trp/kg diet and decreased thereafter (P < 0·05). Fish fed diets containing 3·7, 4·2 and 4·7 g Trp/kg diets had higher GR activity than those fed 2·6, 3·1 and 5·6 g Trp/kg diets. The GST activity was a maximum in fish fed 4·2 g Trp/kg diet, and minimal for fish fed 2·6 g Trp/kg diet (P < 0·05). The AHR activity was significantly increased with rising dietary Trp levels up to 3·7 g Trp/kg diet and decreased thereafter. Fish fed diet containing 4·2 g Trp/kg diet had higher T-GSH content than those fed 2·6 and 5·6 g Trp/kg diets. The quadratic regression analysis showed that there was significant quadratic association between PC, CAT, GPx, GST and dietary Trp levels (Y PC = 0·4572X 2–3·2596X + 8·9483, R 2 = 0·847; Y CAT = –0·0943X 2 + 0·8176X–1·3831, R 2 = 0·786; Y GPx = –0·7406X 2 + 7·4318X + 2·8817, R 2 = 0·868; Y GST = –3·3532X 2 + 30·126X–49·172, R 2 = 0·848).
Table 3. Malondialdehyde (MDA), protein carbonyl (PC) (nmol/mg protein) and glutathione content (GSH) contents (mg/g protein), total superoxide dismutase (T-SOD), catalase (CAT), glutathione-S-transferase (GST), glutathione peroxidase (GPx), glutathione reductase (GR), anti-superoxide anion (ASA), anti-hydroxyl radical (AHR) activities (U/mg protein) in the muscle of hybrid catfish fed with graded levels of Trp for 56 d
(Mean values and standard errors of the mean)

Three replicate groups with six fish in each group. Values within the same rows having different superscripts are significantly different (P < 0·05).
Antioxidant-related genes, GCLC mRNA expression and Keap1-Nrf2 signalling activity
The mRNA levels of the antioxidant-related genes are illustrated in Figs. 6 and 7. The CuZnSOD and CAT mRNA levels in fish fed 4·2 g Trp/kg diet were higher than those in fish fed others (P < 0·05). Compared with the 2·6 g Trp/kg diet, muscle GPx1a and GCLC mRNA levels were higher in fish fed 4·2 and 4·7 g Trp/kg diets (P < 0·05). The GST mRNA level was the lowest in fish fed 5·6 g Trp/kg diet (P < 0·05), and there was no significant difference among other groups. The Nrf2 mRNA level was significantly up-regulated in fish fed 3·1, 4·2 and 4·7 g Trp/kg diets. The Keap1 mRNA level was the highest in fish fed 5·6 g Trp/kg diet and the lowest in fish fed 3·1 g Trp/kg diet (P < 0·05).
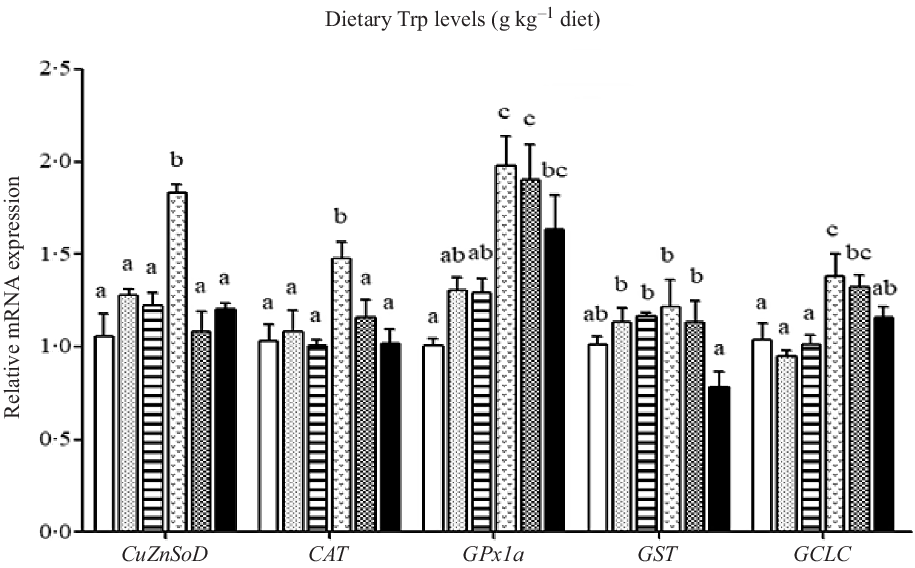
Fig. 6. Relative expression of CuZnSOD, CAT, GST, GPx1a and GCLC genes in the muscle of hybrid catfish Pelteobagrus Vachelli♀ × Leiocassis longirostris♂ fed diets containing graded levels of Trp (g/kg). Data represent means with their standard error of means, of six replicates. Values having different letters are significantly different (P < 0·05). , 2·6;
, 3·1;
, 3·7;
, 4·2;
, 4·7;
, 5·6.
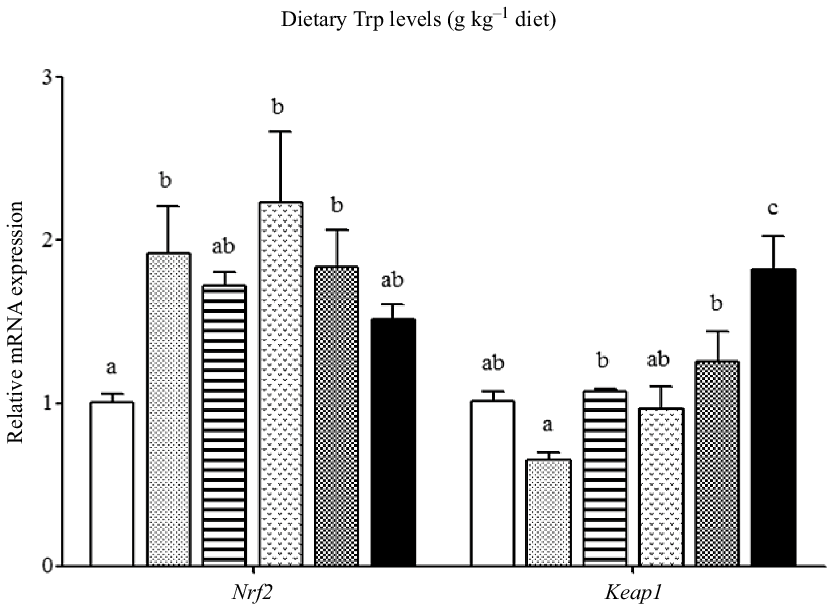
Fig. 7. Relative expression of Nrf2 and Keap1 genes in the muscle of hybrid catfish Pelteobagrus Vachelli♀ × Leiocassis longirostris♂ fed diets containing graded levels of Trp (g kg–1). Data represent means with their standard error of means, of six replicates. Values having different letters are significantly different (P < 0·05). , 2·6;
, 3·1;
, 3·7;
, 4·2;
, 4·7;
, 5·6.
The protein levels of Nrf2 and Keap1 are presented in Fig. 8. The protein levels of nuclear and cytosolic Nrf2 were significantly increased in fish fed 4·2 g Trp/kg diet (P < 0·05). Fish fed 4·2 g Trp/kg diet had lower Keap1 protein level than those fed others (P < 0·05). Additionally, electrophoretic mobility shift assay was applied to detect the antioxidant response element-binding activity of Nrf2. As shown in Fig. 9, the antioxidant response element-binding activity of Nrf2 was improved in fish fed 4·2 g Trp/kg diet.
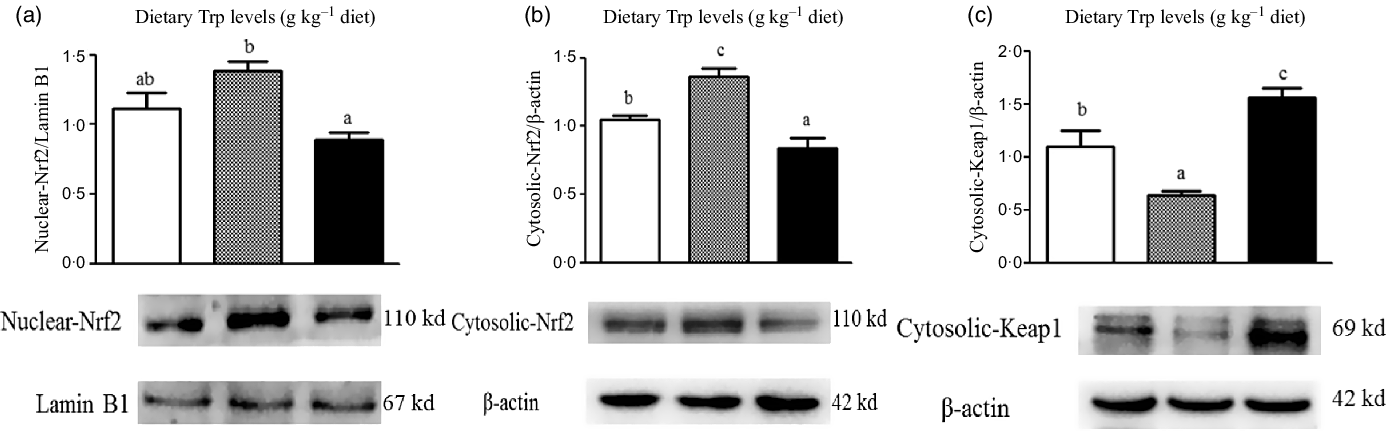
Fig. 8. Dietary supplementation with Trp activated the Keap1-Nrf2 signalling pathway in the muscle of hybrid catfish Pelteobagrus Vachelli♀ × Leiocassis longirostris♂. Protein abundances of Nuclear-Nrf2 (, 2·6;
, 4·2;
, 5·6) (a), cytosolic-Nrf2 (
, 2·6;
, 4·2;
, 5·6) (b)and cytosolic-Keap1(
, 2·6;
, 4·2;
, 5·6) (c) were determined. Data represent means with their standard error of means, of three replicates. Values having different letters are significantly different (P < 0·05).
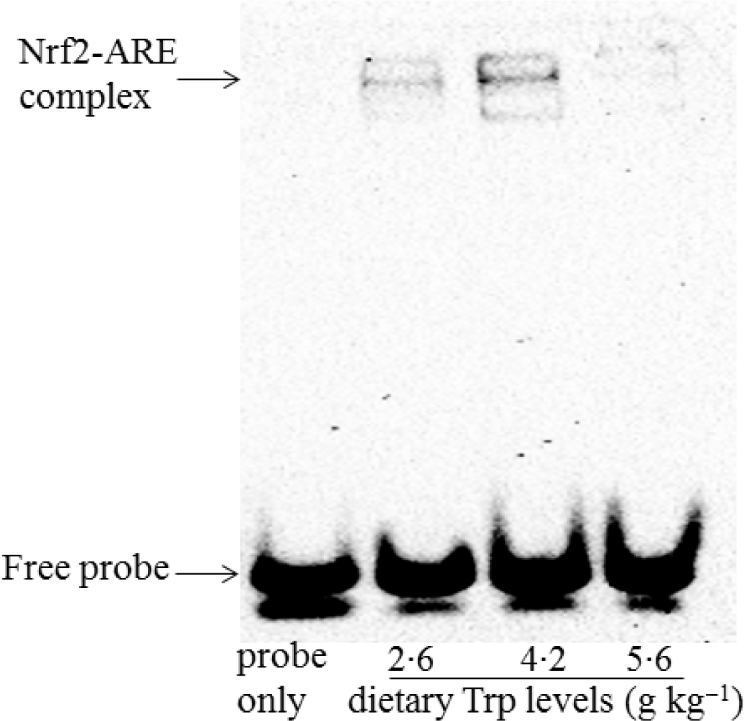
Fig. 9. The ability of Nrf2 binding to the ARE in the muscle of hybrid catfish Pelteobagrus Vachelli♀ × Leiocassis longirostris♂ fed diets containing graded levels of Trp (g/kg). Nuclear extracts were prepared and the biotin-labelled ARE-specific oligonucleotide–protein complexes were detected with an electrophoresis mobility shift assay. This experiment was repeated three replicates with similar results achieved. Representative images were shown.
Correlation analyses
Correlation analyses results are illustrated in online Supplementary Table S4. The frequency of ≤ 20 μm and > 50 μm muscle fibres was correlated positively with IGF-I (r ≤ 20 μm = 0·790, P = 0·062; r > 50 μm = 0·748, P = 0·087), IGF-II (r ≤ 20 μm = 0·970, P = 0·001; r > 50 μm = 0·863, P = 0·027), IGF-IR (r ≤ 20 μm = 0·922, P = 0·009; r > 50 μm = 0·902, P = 0·014), PCNA (r < 20 μm = 0·920, P = 0·009), myf5 (r ≤ 20 μm = 0·600, P = 0·208), MyoD1 (r ≤ 20 μm = 0·714, P = 0·111), MyoG (r > 50 μm = 0·867, P = 0·025) and MRF4 (r > 50 μm = 0·787, P = 0·063) mRNA levels. The muscle protein content was correlated positively with IGF-I (r 0·960, P = 0·002), IGF-II (r 0·605, P = 0·204), IGF-IR (r 0·771, P = 0·072), PIK3Ca (r 0·669, P = 0·146), AKT1 (r 0·434, P = 0·390), TOR (r 0·881, P = 0·020) and S6K1 (r 0·840, P = 0·031) mRNA levels, and correlated negatively with 4EBP1 (r −0·795, P = 0·059) mRNA level. The Nrf2 mRNA levels were correlated positively with CuZnSOD (r 0·829, P = 0·042), CAT (r 0·704, P = 0·118), GPx (r 0·728, P = 0·101), GST (r 0·771, P = 0·072), MyoD1 (r 0·886, P = 0·019), MyoG (r 0·714, P = 0·111), TOR (r 0·593, P = 0·214) and S6K1 (r 0·936, P = 0·006) mRNA levels. To the contrary, the Keap1 mRNA level was correlated negatively with CuZnSOD (r −0·543, P = 0·266), CAT (r −0·429, P = 0·397) and GST (r −0·785, P = 0·064) mRNA levels.
Discussion
As an important tissue in the body, muscle performs vital physiological functions in fish(Reference Valenzuela, Zuloaga and Poblete-Morales44). Previous studies have described the beneficial effect of Trp on immunity, intestinal flora and the nervous system(Reference Ji, Liang and Ren28,Reference Azeredo, Machado and Afonso45,Reference Hoseini, Pérez-Jiménez and Costas46) . However, to the best of our knowledge, there is no available information about the role of Trp in fish muscle growth. The present study, for the first time, demonstrated that dietary Trp had a positive effect on muscle growth in hybrid catfish.
Tryptophan improved the hyperplasia and hypertrophy of fish muscle
As an essential amino acid, Trp has been addressed the importance of impacting fish growth performance(Reference Zhao, Wu and Xu33,Reference Ji, Liang and Chisomo-Kasiya47) . The growth of fish is directly linked to muscle growth(Reference Jiang, Wen and Liu24). Muscle growth mainly results from the hyperplasia and hypertrophy of muscle fibres(Reference Zhao, Li and Jiang5,Reference Koumans, Akster and Booms48) . Muscle fibre density and frequency of fibre diameter are useful quantitative indicators of the hyperplasia and hypertrophy of muscle fibres(Reference Valente, Moutou and Conceicao49). The fibre density and the frequency of diameter ≤ 20 μm muscle fibres denoted fibre hyperplasia and the frequency of diameter > 50 μm muscle fibres marked fibre hypertrophy in fish(Reference de Almeida, Carvalho and Pinhal39,Reference Valente, Rocha and Gomes50) . In the present study, dietary Trp significantly increased muscle fibre density and muscle fibre diameter frequency in ≤ 20 μm or > 50 μm. These results indicated that dietary Trp could improve muscle growth by stimulating muscle fibre hyperplasia and hypertrophy in hybrid catfish. Nevertheless, how dietary Trp improve muscle growth, especially the hyperplasia and hypertrophy, is not fully understood.
Tryptophan improved the growth of fish muscle
The hyperplasia and hypertrophy of muscle are regulated by MRF and MSTN(Reference Koumans, Akster and Booms48,Reference Johnston51) . The MRF are responsible for the synchronised triggering of myosatellite cells activation, proliferation and differentiation. The PCNA, as a marker for entrance into the S phase of the cell cycle, is necessary for myosatellite cells activation(Reference Egan and Zierath52). The myf5 and MyoD1 regulate the proliferation of myoblasts and co-express during the proliferative phase, whereas the MyoG and MRF4 act on differentiation controlling myoblasts fusion to form myotubes(Reference Zanou and Gailly10,Reference Azizi, Nematollahi and Amiri53) . The present study showed dietary Trp significantly up-regulated PCNA, myf5, MyoD1, MyoG and MRF4 mRNA expressions in fish muscle. These results were in good agreement with a report on mice C2C12 muscle cells, where Trp significantly increased MyoG and MyoD1 mRNA expressions(Reference Dukes, Davis and El15). Furthermore, the frequency of ≤ 20 μm fibre was positively correlated with PCNA, myf5 and MyoD1 mRNA levels, and the frequency of > 50 μm fibre was positively correlated with the MyoG and MRF4 mRNA levels, suggesting that the increased muscle fibres hyperplasia and hypertrophy by dietary Trp might be associated with up-regulating the PCNA, myf5, MyoD1, MyoG and MRF4 mRNA levels. Previous studies have found that MSTN negatively regulates muscle growth(Reference Zhu, Liang and Li54). The present study showed dietary Trp decreased muscle MSTN mRNA level. Similar results were observed in mice C2C12 muscle cells(Reference Dukes, Davis and El15). All the results above indicated that dietary Trp could stimulate the hyperplasia and hypertrophy of fish muscle by regulating PCNA, MRF and MSTN expressions. However, more research is required to investigate the specific role of dietary Trp in regulating muscle growth.
The IGF play a crucial role in promoting muscle growth(Reference Azizi, Nematollahi and Amiri53). In fish, the IGF, including IGF-I and IGF-II, exert biological actions primarily through binding and activating IGF-IR(Reference Fuentes, Valdés and Molina11,Reference Azizi, Nematollahi and Amiri53) . The present results showed that dietary Trp significantly increased muscle IGF-I, IGF-II and IGF-IR mRNA expressions. Correlation analysis indicated that the frequency of ≤ 20 and > 50 μm muscle fibres was correlated positively with IGF-I, IGF-II and IGF-IR mRNA levels, indicating that increased muscle hyperplasia and hypertrophy by dietary Trp might partly be related to increased IGF-I, IGF-II and IGF-IR mRNA expressions. Similar results have been reported in mice, where Trp significantly up-regulated muscle IGF-I mRNA expression(Reference Dukes, Davis and El15). Meanwhile, IGF-I and IGF-II mRNA levels correlated positively with IGF-IR mRNA level, suggesting that IGF-I and IGF-II exert its biological functions by binding to IGF-IR. This result was consistent with previous studies in zebrafish. The MRF were regulated by the IGF-I and IGF-II(Reference Zanou and Gailly10). Correlation analysis indicated that IGF-I, IGF-II and IGF-IR mRNA levels were correlated positively with PCNA and MRF mRNA levels. These results, for the first time, showed that dietary Trp could improve muscle growth by regulating MRF expressions via IGF-I-IGF-II/IGF-IR signalling pathway. However, the exact relationship between fish muscle growth and IGF-I-IGF-II/IGF-IR signalling pathway also needed to be further verified.
Tryptophan improved the protein synthesis of fish muscle
Fish muscle growth is a complex and dynamic process that primarily regulated by protein synthesis(Reference Fuentes, Valdés and Molina11). Muscle protein content was strongly connected with the deposition of muscle(Reference Toth, Matthews and Tracy16). In the present study, dietary Trp significantly increased muscle protein content. Similar results have been reported in grass carp(Reference Jiang, Wen and Liu24) and swine(Reference Lin, Smith and Bayley55) muscle. Muscle protein deposition was largely attributed to the increase in protein synthesis(Reference Toth, Matthews and Tracy16,Reference Millward, Garlick and Nnanyelugo56) . The PIK3Ca/AKT1/TOR signalling pathway plays a key role in regulating muscle protein synthesis(Reference Schiaffino and Mammucari18,Reference Bodine, Stitt and Gonzalez19) . The AKT1 phosphorylation is an important marker for activation of the PIK3Ca/AKT1 signalling pathway(Reference Rommel, Bodine and Clarke57). In response to stimulation by the phosphorylation of AKT1, TOR phosphorylates 4EBP1 and S6K1(Reference Jewell, Kim and Russell20). The phosphorylation of 4EBP1 can inhibit protein translation initiation by competitively inhibiting eIF4G binding to eIF4E. The activation of S6K1 can promote protein synthesis(Reference Bodine, Stitt and Gonzalez19). Studies have reported that activating the PIK3Ca/AKT1/TOR signalling pathway contributed to fish muscle protein synthesis(Reference Zhao, Li and Jiang5,Reference Seiliez, Gabillard and Skiba-Cassy58) . The present study showed that dietary Trp increased the phosphorylation of AKT1, TOR, S6K1 and decreased 4EBP1 mRNA level in muscle. Furthermore, muscle protein content was correlated positively with PIK3Ca, AKT1, TOR and S6K1 mRNA levels and correlated negatively with 4EBP1 mRNA level, suggesting that dietary Trp increased muscle protein synthesis through PIK3Ca/AKT1/TOR signalling pathway. These results were in agreement with the recent study in mice, where Trp promoted muscle mass by up-regulating the mTOR and p70S6K expressions(Reference Dukes, Davis and El15). Jiang et al. also reported that fish fed the optimal Trp diet improved TOR mRNA expression and protein phosphorylation in the grass carp muscle(Reference Jiang, Wen and Liu24). The IGF-I and IGF-II could stimulate the activation of the PI3K/AKT1/TOR pathways in fish increasing protein synthesis(Reference Fuentes, Björnsson and Valdés17,Reference Johnston, Bower and Macqueen59) . The present results showed protein content was correlated positively with IGF-I, IGF-II and IGF-IR mRNA levels, which suggested that dietary Trp increasing muscle protein synthesis might be partly associated with IGF-I-IGF-II/IGF-IR signalling pathway. Further correlation analysis indicated that IGF-IR was correlated positively with PIK3Ca, AKT1, TOR and S6K1 mRNA levels, suggesting that dietary Trp could activate PIK3Ca/AKT1/TOR signalling pathway via IGF-I-IGF-II/IGF-IR. To our best knowledge, this study demonstrated for the first time that dietary Trp improved muscle growth by regulating protein synthesis via IGF-I-IGF-II/IGF-IR/PIK3Ca/AKT1/TOR signalling pathway.
Tryptophan improved the antioxidant function of fish muscle
Fish muscle has a high metabolic rate with consuming high oxygen and is easily to be subjected to oxidative stress(Reference Kenari, Regenstein and Hossenini60). Lipid peroxidation and protein oxidation are caused by oxidative stress, and the degree of oxidative stress can be estimated by the MDA and PC contents. In the present study, dietary Trp could effectively reduce MDA and PC contents. Similar results were reported in grass carp and hybrid catfish(Reference Jiang, Wen and Liu24,Reference Zhao, Wu and Xu33) . Oxygen-free radicals, such as superoxide anions (O2–) and hydroxyl radicals (OH–), are the main factors that cause oxidative damage(Reference Jiang, Chen and Liu61). The ASA and AHR activities are two indexes used to evaluate the total scavenging capacities of O2– and OH–, respectively(Reference Jiang, Wu and Zhou40). The present data showed dietary Trp increased fish muscle AHR and ASA activities, indicating total capacity of scavenging O2– and OH– was promoted in fish muscle. The O2– and OH– were scavenged mainly by enzymatic and non-enzymatic antioxidants in fish(Reference Kohen and Nyska62). The SOD, CAT, GST, GR, GPx and GSH are the major antioxidant enzymes and the non-enzymatic antioxidant. The present results showed that dietary Trp increased CAT, GR, GST and GPx activities, and GSH content. Similar results were found in grass carp muscle(Reference Jiang, Wen and Liu24). Correlation analysis indicated that AHR and ASA activities were correlated positively with CAT, GST, GR, GPx mRNA levels and GSH content. These results suggested that Trp improved the capacities of scavenging O2– and OH– via increasing the enzymatic antioxidant activities and non-enzymatic antioxidant content in fish muscle.
The activities of antioxidant enzymes and GSH content are closely related to their transcriptional levels. The present study showed that the expressions of CuZnSOD, CAT, GST and GPx1a were significantly up-regulated in fish fed 4·2 g Trp/kg diet. Similar results were reported in the muscle of grass carp, the kidney of blunt snout bream and the intestine of hybrid catfish(Reference Jiang, Wen and Liu24,Reference Ji, Liang and Ren28,Reference Zhao, Wu and Xu33) . However, the present study showed that dietary Trp increased the mRNA expression of CuZnSOD, but did not affect the activity of SOD. The reason may be that dietary Trp could not influence SOD translational level in muscle. Jiang et al. also demonstrated that Trp could not alter de novo SOD synthesis and thus did not influence its activity(Reference Jiang, Wen and Liu24). The GCLC, as the catalytic subunit of γ-glutamyl cysteine ligase, can limit and catalyse the biosynthesis of GSH from its precursor amino acids(Reference Yang, Magilnick and Lee63). In the present study, dietary Trp significantly up-regulated GCLC mRNA level in muscle, suggesting that Trp increased GSH biosynthesis by promoting GCLC gene transcription. In fish, Nrf2, which was inhibited by Keap1, plays a significant role in the regulation of the antioxidant enzyme-related genes expressions(Reference Jiang, Wen and Liu24,Reference Jiang, Yin and Li64) . The present study showed that Nrf2 mRNA and nuclear Nrf2 level were significantly improved in fish fed 4·2 g Trp/kg diet, while the expression of Keap1 and cytosolic Keap1 was down-regulated. Correlation analysis indicated that Nrf2 mRNA level was correlated positively with the antioxidant genes mRNA levels in fish muscle, whereas Keap1 mRNA level was correlated negatively with these genes mRNA levels. These results indicated that increased antioxidant genes transcription by dietary Trp might be related to the increased Nrf2 and decreased Keap1 transcription. Moreover, the Nrf2 mRNA level also was correlated positively with GCLC mRNA level and GSH content, suggesting that dietary Trp increased GSH content in a dependent of GCLC manner via promoting Nrf2 gene expression. The detailed manner needs further study. These results implied that dietary Trp improved muscle antioxidant enzymes activities and GSH content by regulating the antioxidant enzyme-related genes transcription via Keap1/Nrf2 signalling pathway.
Relationship between the antioxidant system and muscle growth
The analyses of the genome-wide distribution of Nrf2 have identified new sets of Nrf2 target genes whose products are involved in cell proliferation and differentiation(Reference Murakami and Motohashi30). In the present study, dietary Trp increased the muscle antioxidant capacity, proliferation and differentiation in vivo. The positive correlation was observed among MyoD1, MyoG and Nrf2 mRNA levels. These results suggested that dietary Trp improved muscle growth via regulating MyoG and MyoD1 mRNA levels might partly be related to the increased Nrf2 mRNA level. Zhao et al. demonstrated that Nrf2 could improve muscle growth by decreasing oxidative damage(Reference Zhao, Jiang and Zhou4). Al-Sawaf et al. also found that Nrf2 could promote muscle regeneration by regulating MyoD1 and MyoG expressions(Reference Al-Sawaf, Fragoulis and Rosen31). In addition, the non-enzymatic antioxidant defence is partly regulated by GSH in fish muscle. In the present study, the GSH content was correlated positively with MyoD1 and MyoG mRNA levels, suggesting that dietary Trp may affect MyoD1 and MyoG transcriptions via the non-enzymatic antioxidant system. Similarly, Ardite et al. reported that GSH depletion could affect the expression of MyoD and the transition of C2C12 myoblasts into myocytes(Reference Ardite, Barbera and Roca32). These results indicated that increased Nrf2 and GSH by dietary Trp might improve muscle growth by promoting hyperplasia and hypertrophy. Hence, we speculate that the antioxidant system may be involved in muscle growth. On the other hand, Nrf2 expression was modulated by many upstream molecules, such as TOR(Reference Jiang, Wen and Liu24). A previous study reported that TOR and S6K1 could improve Nrf2 expression in mice liver(Reference Bae, Sung and Oh65). In the present study, TOR and S6K1 mRNA levels were correlated positively with Nrf2 mRNA level. Similar results were observed in grass carp(Reference Jiang, Wen and Liu24). These findings suggest that the increased Nrf2 expression by dietary Trp might partly be associated with improved TOR expression. However, the detailed relationship between Trp in muscle growth and the antioxidant system is still unclear in fish and needs further investigation.
Conclusions
The present study, for the first time, showed that that dietary Trp improved muscle hyperplasia and hypertrophy by regulating muscle growth-related genes (IGF-I, IGF-II, IGF-IR, PCNA, MRF and MSTN) expressions, increased muscle protein synthesis via IGF-I-IGF-II/IGF-IR/PIK3Ca/AKT1/TOR signalling pathway. Moreover, dietary Trp improved muscle antioxidant enzymatic and non-enzymatic activities via Keap1/Nrf2 signalling pathway. Finally, based on muscle protein and MDA contents, the optimal Trp requirements of hybrid catfish (21·82–39·64 g) were estimated to be 3·94 and 3·93 g Trp/kg diet (9·57 and 9·54 g/kg of dietary protein), respectively.
Acknowledgements
This study was financially supported by National Key R&D Program of China (2019YFD0900200) and National Natural Science Foundation of China (31702362).
Q. J. and Y. Z. conducted the trial, performed the RT-PCR experiments, western blot and wrote the manuscript. J. J. and X-Q. Z. contributed to the design of the study. L. F. and W-D. J assisted in the manuscript preparation. X.-Y. W, S.-X. X and J. Z. assisted with all data analysis. Y. L. assisted with the trail. All authors read and approved the final manuscript.
The authors declare no conflict of interest.
Supplementary material
For supplementary materials referred to in this article, please visit https://doi.org/10.1017/S0007114521002828