Introduction
Human activities have contributed to increased global surface temperatures, which are currently 1.09°C above pre-industrial levels (IPCC 2022). Global climate change (CC) is recognized as a major threat to many species and to the integrity of whole ecosystems (Pereira et al. Reference Pereira, Leadley, Proença, Alkemade, Scharlemann, Fernandez-Manjarrés and Araújo2010, IPCC 2018, 2022). The impacts of CC on biodiversity are already evident for multiple taxa (Pereira et al. Reference Pereira, Leadley, Proença, Alkemade, Scharlemann, Fernandez-Manjarrés and Araújo2010, Pacifici et al. Reference Pacifici, Visconti, Butchart, Watson, Cassola and Rondinini2017) and include shifts in species ranges (e.g., Pounds et al. Reference Pounds, Fogden and Campbell1999), changes in phenology (e.g., Walther et al. Reference Walther, Post, Convey, Menzel, Parmesan, Beebee and Fromentin2002) and epidemic disease emergencies (e.g., Pounds et al. Reference Pounds, Bustamante, Coloma, Consuegra, Fogden, Foster and La Marca2006), which in turn may affect fitness, increasing extinction risk (Pounds et al. Reference Pounds, Bustamante, Coloma, Consuegra, Fogden, Foster and La Marca2006, Berriozabal-Islas et al. Reference Berriozabal-Islas, Ramírez-Bautista, Torres-Ángeles, Mota Rodrigues, Macip-Ríos and Octavio-Aguilar2020).
Assessments of the possible deleterious impacts of CC on species performances are crucial for designing conservation strategies. Research related to the CC vulnerability of species is rapidly increasing worldwide (Foden et al. Reference Foden, Young, Akçakaya, Garcia, Hoffmann, Stein and Thomas2019). Although different approaches have been proposed, trait-based assessments have been adopted by many researchers and conservation organizations (Young et al. Reference Young, Dubois and Rowland2015, Foden et al. Reference Foden, Young, Akçakaya, Garcia, Hoffmann, Stein and Thomas2019). These assessments are built on known or inferred associations between biological traits and possible negative impacts of changes in climatic conditions (Foden et al. Reference Foden, Young, Akçakaya, Garcia, Hoffmann, Stein and Thomas2019). Foden et al. (Reference Foden, Butchart, Stuart, Vié, Akçakaya and Angulo2013) developed a framework to assess the relative vulnerability of species to CC, and this has been used in several investigations with different biological groups, including corals (Foden et al. Reference Foden, Butchart, Stuart, Vié, Akçakaya and Angulo2013), amphibians (Foden et al. Reference Foden, Butchart, Stuart, Vié, Akçakaya and Angulo2013, Carr et al. Reference Carr, Hughes and Foden2014), reptiles (Carr et al. Reference Carr, Hughes and Foden2014, Böhm et al. Reference Böhm, Cook, Ma, Davidson, García and Tapley2016, Meng et al. Reference Meng, Carr, Beraducci, Bowles, Branch, Capitani and Chenga2016), birds (Foden et al. Reference Foden, Butchart, Stuart, Vié, Akçakaya and Angulo2013, Carr et al. Reference Carr, Hughes and Foden2014, Borges et al. Reference Borges, Ribeiro, Lopes and Loyola2019) and mammals (Carr et al. Reference Carr, Hughes and Foden2014). This framework allows three dimensions of CC vulnerability to be independently assessed: sensitivity, low adaptive capacity and exposure (Foden et al. Reference Foden, Butchart, Stuart, Vié, Akçakaya and Angulo2013). ‘Sensitivity’ in this sense is the lack of potential for a species to persist in situ given a certain CC scenario, and it is directly related to life-history traits. ‘Low adaptive capacity’ implies the inability of a species to endure the negative impacts of CC by dispersal and/or micro-evolutionary changes. ‘Exposure’ refers to the magnitude and rate at which a species’ physical environment is expected to change due to CC (Foden et al. Reference Foden, Butchart, Stuart, Vié, Akçakaya and Angulo2013, Carr et al. Reference Carr, Hughes and Foden2014).
Global vulnerability assessments provide comprehensive pictures of different taxa and facilitate comparisons at a large scale, while regional and country-level analyses are needed for conservation decision-making (Di Minin et al. Reference Di Minin, Soutullo, Bartesaghi, Rios, Szephegyi and Moilanen2017). Biological information of local populations is often available in local grey literature. Working at finer scales with local field researchers enables data gathering on valuable and variable attributes that would not be available otherwise (Grattarola et al. Reference Grattarola, Martínez-Lanfranco, Botto, Naya, Maneyro and Mai2020). Additionally, some species’ attributes are sensitive to scale (Ficetola et al. Reference Ficetola, Lunghi, Canedoli, Padoa-Schioppa, Pennati and Manenti2018).
In the case of ectothermic organisms such as amphibians and reptiles, humidity and temperature are of particular relevance as they may constrain the timing of several physiological and demographic processes (Wells Reference Wells2007, Sinervo et al. Reference Sinervo, Mendez-De-La-Cruz, Miles, Heulin, Bastiaans and Villagrán-Santa Cruz2010). Examples of CC impacts on the conservation status of many amphibians and reptiles have been reported elsewhere (e.g., Pounds et al. Reference Pounds, Fogden and Campbell1999, Reading Reference Reading2007). Studies assessing their vulnerability to future CC at global (e.g., Foden et al. Reference Foden, Butchart, Stuart, Vié, Akçakaya and Angulo2013, Böhm et al. Reference Böhm, Cook, Ma, Davidson, García and Tapley2016), regional (e.g., Carr et al. Reference Carr, Hughes and Foden2014) and local scales (e.g., Laufer Reference Laufer2012, Meng et al. Reference Meng, Carr, Beraducci, Bowles, Branch, Capitani and Chenga2016) suggest a range of possible scenarios in which some species would be imperilled. The vulnerability of Uruguayan herpetofauna to CC has been previously evaluated as well (Laufer Reference Laufer2012, Toranza et al. Reference Toranza, Maneyro and Brazeiro2012). However, over the last decade, Uruguay has undergone significant changes in land use, mostly agriculture intensification, exotic afforestation and urbanization (Brazeiro et al. Reference Brazeiro, Achkar, Toranza and Bartesaghi2020). In addition, recent taxonomic changes (e.g., reports of new species and the synonymization of others) and relevant data on life-history traits of many species have also accumulated. These suggest that an updated assessment of the Uruguayan herpetofauna’s vulnerability to CC is necessary.
We assessed the relative vulnerability of Uruguayan continental amphibians and reptiles to CC, including 48 amphibian and 64 reptile native species (Frost Reference Frost2021, Uetz et al. Reference Uetz, Freed and Hošek2021). We excluded alien species occurring in Uruguay: two reptiles (Hemidactylus mabouia and Tarentola m. mauritanica) and one amphibian (Lithobates catesbeianus). To conduct the assessment, we applied the global approach proposed by Foden et al. (Reference Foden, Butchart, Stuart, Vié, Akçakaya and Angulo2013) with proper adjustments for its use at this level. The evaluation was implemented through an expert elicitation process based on the Delphi method. This process allowed us to identify data gaps in life-history traits, discuss the challenges associated with the change of scale and compare our results with the International Union for Conservation of Nature (IUCN) Red List for Uruguay.
Methods
Study region
Located in the southern Neotropical region (Morrone Reference Morrone2015), Uruguay’s climate is temperate wet with average annual precipitation of 1200–1600 mm (statistical period 1980–2009) over a latitudinal range of 30–35°S. The average annual temperature is 17.7°C, varying from 19.8°C in the extreme north-west to 16.6°C over the south-eastern Atlantic coast (MGAP-FAO 2012). The landscape mostly consists of rolling plains, with some low hilly areas up to 513 m altitude, and it is part of the Pampas biome with influences from the Chacoan and Paranaense biogeographical provinces (MVOTMA 2010, Morrone Reference Morrone2014). Uruguay has a coastline of c. 670 km (Evia & Gudynas Reference Evia and Gudynas2000).
Climate change vulnerability framework
We assessed species vulnerability to CC using the sensitivity, low adaptive capacity and exposure dimensions of Foden et al. (Reference Foden, Butchart, Stuart, Vié, Akçakaya and Angulo2013). The most vulnerable taxa are those exposed to CC, presenting high sensitivity and low adaptive capacity (Fig. 1). Appropriate traits were selected for each dimension during the expert elicitation process (Tables 1 & 2 & Supplementary Appendix S1, available online). Species received the scores ‘low’, ‘high’ or ‘unknown’ for each trait. Those that scored ‘high’ in at least one of the traits of a dimension were classified as ‘high’ with regard to that dimension. Species qualifying as ‘high’ in all three dimensions were considered to be highly vulnerable to CC. Species were also classified as potential adapters (i.e., sensitive and exposed but highly adaptable), potential persisters (exposed with low adaptive capacity but not sensitive) or with high latent risk (sensitive with low adaptive capacity but currently not exposed; Fig. 1).

Fig. 1. Framework to assess vulnerability to climate change (CC): 1 – highly vulnerable species: sensitive, with low adaptive capacity and exposed to CC (greatest concern); 2 – potential adapters: sensitive and exposed species but highly adaptable; 3 – potential persisters: exposed species with low adaptive capacity but not sensitive; 4 – high latent risk: sensitive species with low adaptive capacity but not exposed at the moment. Modified from Foden et al. (Reference Foden, Butchart, Stuart, Vié, Akçakaya and Angulo2013).
Table 1. Traits of amphibians considered for the three dimensions of climate change vulnerability.
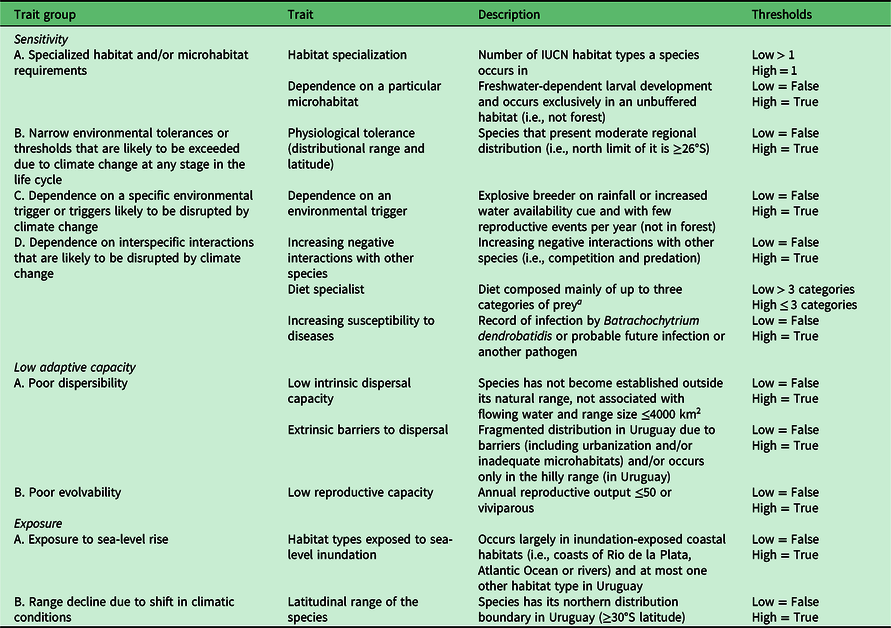
a Food categories: spiders, ticks, other mites, cockroaches, mantises, butterflies, moths, beetles, bees, aphids, cicadas, fleas, flies, dragonflies, ants, centipedes, millipedes, non-arthropod invertebrates, amphibians, fish and birds.
IUCN = International Union for Conservation of Nature.
Table 2. Traits of reptiles considered for the three dimensions of climate change vulnerability.

a Identified microhabitats: streams or ravines in Uruguayan hilly range; ephemeral ponds, vines, fallen trees, dead wood, tree hollows, trees at the water’s edge, gallery or riparian forests, anthills, termite mounds, dunes, open patches in grasslands, rocky areas and outcrops, cliffs and caves; freshwater or forest dependent.
b Food categories: leaf matter; fruit; seeds; nectar; a single taxonomic group of arthropod; a range of arthropods; other invertebrates; small mammals ≤300 mm snout–vent length; large mammals >300 mm snout–vent length; adult/subadult birds; bird eggs/juveniles; adult/juvenile reptiles; reptile eggs; adult amphibians; amphibian larvae; freshwater fish; faeces; and an ‘other’ category for anything outside of these parameters.
IUCN = International Union for Conservation of Nature.
For the assessment of sensitivity, we used the following trait sets: specialized habitat and/or microhabitat requirements; narrow environmental tolerance or thresholds that are likely to be exceeded due to CC at any stage in the life cycle; dependence on a specific environmental trigger or triggers likely to be disrupted by CC; and dependence on interspecific interactions that are likely to be disrupted by CC. For low adaptive capacity, we used the following trait sets: poor dispersibility; and poor evolvability. Finally, for exposure, we used the following trait sets: exposure to sea-level rise; and range decline due to shift in climatic conditions (described in Appendix S1).
Temperature and precipitation inferences were based on the local and regional climatic projections that rely on the ensemble of four general circulation models (ACCESS1.0, CanESM2, CCSM4 and HadGEM2) presented by Nagy et al. (Reference Nagy, Bidegain, Verocai and de los Santos2016), who considered two emissions scenarios (4.5 and 8.5 Representative Concentration Pathways (RCPs)) for 2050 (2040–2060; Appendix S1 & Figs S1 & S2). The cited authors also calibrated satellite data with tidal scales installed at different places over the Uruguayan coast. The coastal zones of Uruguay are considered to be among the most exposed to extreme events and sea-level rise in Latin America (Losada et al. Reference Losada, Reguero, Méndez, Castanedo, Abascal and Mínguez2013).
Expert elicitation and score integration
The trait-based vulnerability assessment was implemented through a structured elicitation process based on the Delphi method, combining expert judgement with data collection, in an anonymous and iterative way. Anonymity minimizes the social pressures of group approaches but poses the risk of a lack of accountability in the responses. This problem was limited through a face-to-face discussion workshop after the anonymous responses were received (Mukherjee et al. Reference Mukherjee, Hugé, Sutherland, McNeill, Van Opstal, Dahdouh-Guebas and Koedam2015).
The expert consultation process consisted of three stages: pre-elicitation (planning the consultation); the elicitation itself; and post-elicitation. The first stage involved the definition of objectives and gathering contextual information, identifying a group of experts, clarifying the number of rounds, selecting which items remained in successive rounds and determining how the level of consensus was going to be quantified (Diamond et al. Reference Diamond, Grant, Feldman, Pencharz, Ling, Moore and Wales2014, Mukherjee et al. Reference Mukherjee, Hugé, Sutherland, McNeill, Van Opstal, Dahdouh-Guebas and Koedam2015).
The number of rounds established a priori was two in order to avoid participant fatigue and a higher attrition rate (Powell Reference Powell2003). Groups of four experts on amphibians (Claudio Borteiro, Diego Baldo, Carlos Prigioni and Gabriel Laufer) and three experts on reptiles (the same experts as for amphibians except Diego Baldo) were invited to participate as co-authors. A primary session was conducted as an online workshop to introduce the methodology. Subsequently, we organized a second workshop to define the traits to include, in which we selected those relevant for the evaluation that we considered would offer useful information for scoring the Uruguayan herpetofauna. Additionally, the arbitrariness of some thresholds used to set the scores can be problematic (Foden et al. Reference Foden, Young, Akçakaya, Garcia, Hoffmann, Stein and Thomas2019). To avoid this, discussions were held to define the thresholds as clearly and objectively as possible. This resulted in the selection of seven traits for the sensitivity dimension of amphibians, three traits for low adaptive capacity and two for exposure (Tables 1 & S1). For reptiles, eight traits were selected for sensitivity, four for low adaptive capacity and two in the case of exposure (Tables 2 & S2).
For the first round, experts were provided with a document containing the methodology and a spreadsheet containing the list of species and traits to be considered. Each expert conducted an initial individual round of evaluation, in which every species was assigned scores of ‘low’, ‘high’ or ‘unknown’ for all traits based on published and grey literature, their own field knowledge or inference from related species (as occurred for the diets of Melanophryniscus atroluteus, Melanophryniscus devincenzii, Melanophryniscus lanogonei, Melanophryniscus pachyrhynus and Melanophryniscus sanmartini). The ‘unknown’ category was used when the expert did not feel confident in supporting a trait assignment using the data available, thus avoiding scoring with high uncertainty. The first round of responses was integrated, leaving for the second round of anonymous consultations only those species for which there was no full consensus (i.e., no total agreement of the experts for a given score). At the second and last round, experts were supplied with the anonymous answers of their counterparts from the first round and the same spreadsheet format previously used. By doing this, each participant could carefully reconsider their own answers in case of doubt.
For score integration, when the majority of the experts agreed on a given score, that score was assigned to the trait. When score assignments were tied, the traits were considered as ‘high’ when they competed with any other score and ‘low’ when the alternative was ‘unknown’. To account for the uncertainty, we repeated the analysis treating the ‘unknown’ scores as ‘low’ in an optimistic scenario (results in main manuscript; Tables S3–S8) and as ‘high’ in a pessimistic one (results in Tables S5, S6, S9 & S10).
Results
For most of the species and traits analysed, the available information enabled the assessment to be completed. Yet there were some important data gaps. For instance, in the case of amphibians, the trait ‘increasing susceptibility to diseases’ was scored as ‘unknown’ for 62.5% of the species (Fig. 2 & Tables S1 & S3). Reptile data gaps were more important for ‘generation length’ (73.4% of species) and, to a lesser extent, ‘temperature-dependent gender’ (12.5%; Fig. 3 & Tables S2 & S4). However, data gaps viewed at the species level were of less importance, providing strong support for the analysis.

Fig. 2. Proportions of amphibian species classified as ‘high’, ‘low’ or ‘unknown’ for each trait considered for the three dimensions of vulnerability: (a) sensitivity score, (b) low adaptive capacity score and (c) exposure score.

Fig. 3. Proportions of reptile species classified as ‘high’, ‘low’ or ‘unknown’ for each trait considered for the three dimensions of vulnerability: (a) sensitivity score, (b) low adaptive capacity score and (c) exposure score.
Seven amphibians and seven reptiles were classified as highly vulnerable to CC (i.e., qualifying as vulnerable in all three dimensions), representing 14.6% and 10.9%, respectively, of the species assessed from both groups (Fig. 1 & Table 3). One amphibian was categorized as a potential adapter (i.e., sensitive and exposed but adaptable). Six amphibians and 19 reptiles (12.5% and 29.7%, respectively) were presented as bearing high latent risk (sensitive with low adaptive capacity but currently not exposed). There were no species classed as potential persisters (exposed with low adaptive capacity but not sensitive), while 17 amphibians and 38 reptiles were only seen as sensitive (35.4% and 59.4%, respectively; Tables 3, S7 & S8).
Table 3. Number and percentage of species in each of the four climate change vulnerability categories. Numbers in parentheses represent a pessimistic scenario (i.e., treating ‘unknowns’ as ‘high’). Crosses represent dimensions classified as ‘high’, dashes represent dimensions classified as ‘low’.

a Numbers in parentheses represent the climate change vulnerability categories indicated in Fig. 1.
Regarding sensitivity, 64.6% of amphibians and 100% of reptiles were scored as highly sensitive to CC (Tables S1–S4). The traits that contributed the most for amphibians were ‘physiological tolerance’ (45.8% of the species) and ‘dependence on an environmental trigger’ (45.8%; Fig. 2), while those for reptiles were ‘dependence on an environmental trigger’ (98.4%), followed by ‘temperature-dependent gender’ (67.2%; Fig. 3).
Remarkably, most of the studied species were presented as being potentially adaptable to CC, as only 13 amphibians (27.1%) and 26 reptiles (40.6%) presented low adaptive capacity (Tables S1–S4). For amphibians, the trait that contributed the most in this regard was ‘low intrinsic dispersal capacity’, which scored high in 25% of the species (Fig. 2 & Table S3), with seven of them showing poor adaptability exclusively due to this. For reptiles, the trait that contributed the most in this regard was ‘low reproductive capacity’ (21.9%), with six species meeting the criteria for poor adaptability exclusively due to this trait. The next most common trait for reptiles scoring ‘high’ in this regard was ‘low intrinsic dispersal capacity’ (18.8%; Fig. 3 & Table S4).
Only eight amphibians (16.7%) and seven reptiles (10.9%) were rated as exposed to CC (Tables S1–S4). For amphibians, the two traits defined for this dimension (‘habitat types exposed to sea-level inundation’ and ‘latitudinal range of the species’) contributed equally (12.5% of species). For reptiles ‘latitudinal range of the species’ contributed the most (7.8%; Figs 2 & 3 & Tables S3 & S4).
Four of the seven amphibian species (57.1%) classified here as highly vulnerable to CC were also categorized as Threatened according to the IUCN Red List for Uruguay (& Table S7). On the other hand, only two of the seven reptile species classified herein as highly vulnerable to CC (28.6%) are locally threatened (Table S8).
Discussion
We identified 14 species of the continental Uruguayan herpetofauna as highly vulnerable to CC, with almost two-thirds of amphibians and all reptiles being highly sensitive. Many of these species are strongly influenced by specific environmental factors affected by CC. This is more evident in reptiles, which are highly dependent on weather/climate signals for reproduction (e.g., Balestrin & Cappellari Reference Balestrin and Cappellari2011, Verrastro & Rauber Reference Verrastro and Rauber2013). Similarly, nearly half of the local amphibian fauna are explosive breeders depending on the rainfall regime (Kolenc Reference Kolenc1987, Moreira et al. Reference Moreira, Knauth and Maltchik2014).
Highly vulnerable amphibians share some biological characteristics such as being explosive breeders with few reproductive events per year and small geographical ranges. Most of these species (M. langonei, M. montevidensis, M. sanmartini, Ceratophrys ornata, Odontophrynus maisuma and Physalaemus fernandezae) are local habitat specialists (García Reference García1972, Kolenc Reference Kolenc1987, Rosset Reference Rosset2008). Additionally, populations of M. montevidensis, C. ornata, O. maisuma and Nyctimantis siemersi seem to depend on coastal habitats (García Reference García1972, Prigioni & Garrido Reference Prigioni and Garrido1989, Rosset Reference Rosset2008). These frogs may be severely affected by an increase in sea level. Additionally, M. langonei and M. sanmartini dwell exclusively in hilly areas, and these species plus M. montevidensis, C. ornata and O. maisuma also depend on seasonal ephemeral environments (García Reference García1972, Prigioni & Garrido Reference Prigioni and Garrido1989, Borteiro et al. Reference Borteiro, Kolenc, Pereyra, Rosset and Baldo2010) that are very fragile and would be particularly affected by CC (Foden et al. Reference Foden, Butchart, Stuart, Vié, Akçakaya and Angulo2013).
On the other hand, it was suggested that some amphibians with northern distributions in the country are likely to expand their geographical ranges in a southwards direction (Toranza et al. Reference Toranza, Maneyro and Brazeiro2012). In the case of Dendropsophus minutus, Scinax nasicus, Scinax fuscovarius and Physalaemus riograndensis, local southwards expansions have been already observed (Laufer et al. Reference Laufer, Gobel, Kacevas, Lado, Cortizas, Carabio and Kolenc2021b). This phenomenon, if occurring in reptiles (i.e., ophidians), would be more difficult to observe due to the magnitude of the sampling effort required.
All of the highly vulnerable reptiles are dependent on weather or seasonal changes to initiate breeding or egg deposition (Balestrin & Cappellari Reference Balestrin and Cappellari2011, Verrastro & Rauber Reference Verrastro and Rauber2013). The habitat specialists Liolaemus wiegmannii and Liolaemus occipitalis depend on coastal areas, particularly the microhabitats of sand dunes (Etheridge Reference Etheridge2000), areas highly exposed to extreme events due to CC. Additionally, Phrynops williamsi may present sex determination mediated by temperature during egg incubation. Imbalance in the sex ratios of embryos has already been demonstrated in laboratory studies exposing the eggs of painted turtles (Chrysemys picta) to temperature fluctuations (Valenzuela et al. Reference Valenzuela, Literman, Neuwald, Mizoguchi, Iverson, Riley and Litzgus2019). Consequently, if thermal fluctuations rise with CC, a sex imbalance in populations could occur, leading to extinction (Böhm et al. Reference Böhm, Cook, Ma, Davidson, García and Tapley2016, Valenzuela et al. Reference Valenzuela, Literman, Neuwald, Mizoguchi, Iverson, Riley and Litzgus2019).
Important data gaps were identified, particularly on susceptibility to diseases. We considered this trait as ‘high’ if there was a previous record of skin infection by Batrachochytrium dendrobatidis or other pathogens, since this fungus has been implicated in population and species declines worldwide (e.g., Jani & Briggs Reference Jani and Briggs2014), and CC has been suggested as a potential trigger of chytridiomycosis (Pounds et al. Reference Pounds, Bustamante, Coloma, Consuegra, Fogden, Foster and La Marca2006). Several cases of infection are known for Uruguayan species (Borteiro et al. Reference Borteiro, Cruz, Kolenc and Aramburu2009, Reference Borteiro, Gobel, Kolenc, Laufer, Martínez Debat and Ubilla2018, Reference Borteiro, Kolenc, Verdes, Martínez Debat and Ubilla2019), and we are unaware of the epidemiological relevance of locally invasive and currently expanding American bullfrogs (Lithobates catesbeianus), carriers of amphibian chytrids (Laufer et al. Reference Laufer, Canavero, Núñez and Maneyro2008, Reference Laufer, Gobel, Borteiro, Soutullo, Martínez-Debat and de Sá2018) that also compete with and predate upon local species (Gobel et al. Reference Gobel, Laufer and Cortizas2019, Laufer et al. Reference Laufer, Gobel, Berazategui, Zarucki, Cortizas and Soutullo2021a). Among reptiles, there is sparse information regarding the impacts of life-history traits on species longevity and temperature-dependent sex determination of offspring, in spite of the latter trait’s relevance in a CC scenario.
We observed an overall correspondence (57.1%) between the level of concern in IUCN local categorizations of local amphibians and our vulnerability assessment to CC. While the local Red List considered all six species of Melanophryniscus as vulnerable to CC (following Zank et al. Reference Zank, Becker, Abadie, Baldo, Maneyro and Borges-Martins2014), we only considered M. langonei, M. montevidensis and M. sanmartini in this regard. By contrast, we did not find a clear association between threatened reptiles in the local Red List and those with high vulnerability to CC. Only two species among those classified here as highly vulnerable to CC (28.6%) are locally threatened according to the IUCN (L. wiegmannii and L. occipitalis; Table S12). However, the IUCN Red List for reptiles did not take CC as an explicit classification criterion, as in the published Red List for local amphibians (Carreira & Maneyro Reference Carreira and Maneyro2015). It is noteworthy that CC is only one of the agents of the global changes to which species are exposed as their conservation status is also a function of habitat loss due to human activities and losses to pet trade and consumption. Therefore, multi-factorial studies that combine these factors are needed to precisely define the status of these species (Ficetola & Maiorano Reference Ficetola and Maiorano2016).
When compared with previous local evaluations of the vulnerability of native amphibians to CC (Laufer Reference Laufer2012, Toranza et al. Reference Toranza, Maneyro and Brazeiro2012), only M. montevidensis has been consistently classified as highly vulnerable (Table S11). However, this species was classed as a potential adapter (sensitive and exposed but adaptable) at a global level (Foden et al. Reference Foden, Butchart, Stuart, Vié, Akçakaya and Angulo2013). M. langonei and M. sanmartini are considered vulnerable to CC in the present study as well as by Laufer (Reference Laufer2012). Despite the differences among the studies, Melanophryniscus species appear to be highly vulnerable to CC, which also accords with Zank et al. (Reference Zank, Becker, Abadie, Baldo, Maneyro and Borges-Martins2014). Regarding reptiles, only one species (L. wiegmannii) shared the classification of vulnerability with the previous local study by Laufer (Reference Laufer2012). Our study identified four additional amphibians and six additional reptiles as potentially highly vulnerable to CC.
We followed the approach of Foden et al. (Reference Foden, Butchart, Stuart, Vié, Akçakaya and Angulo2013) but made some adjustments that introduce caveats to consider, as we are applying an approach developed for global assessments to a local level. The challenges associated with changing the scale include adjusting the traits to the available information and modifying trait definitions to account for geographical variations, ecosystems and species’ habitats. For instance, regarding the trait ‘extrinsic barriers to dispersal’ used by Carr et al. (Reference Carr, Hughes and Foden2014) and Böhm et al. (Reference Böhm, Cook, Ma, Davidson, García and Tapley2016) to distinguish species restricted to high-altitude habitats (>1000 m above sea level), this is useless as such in Uruguay, where altitude is not critical to defining well-differentiated environments as in other ecoregions/biomes. Nevertheless, there are species exclusively associated with Uruguayan hilly areas (150–513 m), clearly defining low-altitude ecosystems. In this sense, we took into account those species that occur only in the hilly range (in Uruguay). Similarly, some authors have used for exposure an approach based on projections of the climatic variables of temperature and precipitation with arbitrary thresholds (Foden et al. Reference Foden, Butchart, Stuart, Vié, Akçakaya and Angulo2013, Carr et al. Reference Carr, Hughes and Foden2014, Meng et al. Reference Meng, Carr, Beraducci, Bowles, Branch, Capitani and Chenga2016), while we used the northern limits of species’ global ranges as a proxy of the area that will be exposed to changes of temperature and precipitation in the near future (i.e. by 2050). An advantage of this proxy is that it represents absolute rather than relative measures. These adjustments should better represent processes that occur at finer scales, such as the criteria used in the regional IUCN Red List (IUCN 2012). Lastly, we want to stress one overarching advantage of trait-based approaches: the use of the current understanding of species ecology and evolutionary biology to infer how they will respond to changes that might not have been experienced by species in the past (e.g., Norris Reference Norris2004). Species distribution models use statistical associations between patterns of abundance and demography and habitat and climatic characteristics within the range of conditions observed in the present or the past to predict possible responses of populations to future CC (e.g., Austin & Van Niel Reference Austin and Van Niel2011). By contrast, trait-based approaches inform management decisions on the basis of what ecological and evolutionary theories predict on how species will adapt to changing conditions (e.g., Carroll et al. Reference Carroll, Jørgensen, Kinnison, Bergstrom, Denison and Gluckman2014).
Our assessment using an expert consultation process might be biased because personal judgements may be overconfident (Moore & Healy Reference Moore and Healy2008), poorly calibrated, self-serving or not based on solid data, in turn leading to poor inferences (Martin et al. Reference Martin, Burgman, Fidler, Kuhnert, Low-Choy, Mcbride and Mengersen2012). Additionally, here we considered expert assessments as being of equal weight, while the data provided by each researcher depend on their degree of knowledge regarding each particular species (Marti et al. Reference Marti, Mazzuchi, Cooke, Hanea, Nane, Bedford and French2021). To overcome these difficulties, we conducted a face-to-face workshop after the rounds of anonymous consultations. However, we consider the Delphi method and the posterior data synthesis to be a useful framework as it allows for the gathering of more and better information than if we had only relied on published sources. In addition, this process enables the systematizing of a large quantity of data for further analysis (Knol et al. Reference Knol, de Hartog, Boogaard, Slottje, van der Sluijs and Lebret2009). Finally, the involvement of experts in conservation evaluations provides a means to bridge the widely recognized research–implementation gap in management and conservation science (Knight et al. Reference Knight, Cowling, Rouget, Balmford, Lombard and Campbell2008).
In this study, we classed species into different categories of vulnerability that could be at risk in the near future due to CC, and we identified the traits associated with this risk. These are species in which conservation efforts should be concentrated; the highly vulnerable species need to be the highest priority (Fig. 1). Species-specific studies on longevity, temperature-dependent sex determination, physiological tolerance and the effects of B. dendrobatidis on native amphibians would be valuable to better inform the outputs of future CC vulnerability assessments. We contend that our adjustment of an approach developed for global assessments to a local scale is also applicable to CC vulnerability assessments in other regions.
Supplementary material
For supplementary material accompanying this paper, visit https://doi.org/10.1017/S0376892922000418.
Acknowledgements
We thank the editors of this journal and anonymous reviewers whose comments and suggestions greatly improved the quality of the manuscript.
Financial support
This work was supported by Agencia Nacional de Investigación e Innovación (ANII), Award number POS_NAC_2018_1_151656 and the Programa de Desarrollo de las Ciencias Básicas (PEDECIBA). GL, CB and AS are members of the Sistema Nacional de Investigadores (SNI), Uruguay.
Competing interests
The authors declare none.
Ethical standards
None.