Introduction
The global cancer consensus 2018 reported that breast cancer was the second most common cancer in the world next to lung cancer, and it was placed first among women. Female breast cancer patients had the highest mortality rate compared to any other cancers. Reference Bray, Ferlay, Soerjomataram, Siegel, Torre and Jemal1 Adjuvant radiotherapy is the standard of care in early breast cancer patients and has the potential to reduce the mortality rate by around 15% compared to no radiotherapy. Reference Sedlmayer, Sautter-Bihl and Budach2 Radiotherapy in association with chemotherapy increases the disease-free survival and overall survival of breast cancer patients. Reference Gianni, Dafni and Gelber3,4 However, this increases the risk of cardiac toxicity especially in patients treated for left-sided breast cancers. Reference Offersen, Højris and Overgaard5 Studies found that even low amounts of radiation doses can cause late cardiac toxicity. Reference Tanguturi, Lyatskaya and Chen6,Reference Vuong, Garg, Bourgeois, Yu, Sehgal and Daroui7,Reference Kenneth, Ulf, Kristina and Anders8 However, the substructures of heart responsible for cardiac toxicity, the pathogenesis and the accurate dose–response correlations have not been well established. Currently, different radiotherapy techniques are being employed to treat carcinoma of breast. These techniques include three-dimensional conformal radiotherapy, intensity-modulated radiotherapy (IMRT), volumetric-modulated arc therapy (VMAT) and helical tomotherapy (HT). The conventional field-in-field-based forward IMRT has been considered the standard technique for many years. Reference Mendez, Louie and Moreno9,Reference Osman, Hol, Poortmans and Essers10 However, achieving the high-dose constraints to heart in free breathing (FB) conditions necessitates the utilisation of inverse planning IMRT, VMAT or HT at the cost of a high integral dose to the area.
Gated radiotherapy with the breath hold can also be used effectively to reduce the respiratory induced anatomical structure variations in breast radiotherapy. Deep inspiration breath hold (DIBH) technique showed the potential advantage in reducing the heart and lung doses for left breast carcinoma radiotherapy. Reference Osman, Hol, Poortmans and Essers10,Reference Pham, Ward and Latty11,Reference Duma, Baumann and Budach12 Hypo-fractionated radiotherapy is the current standard of breast radiotherapy in many countries. The heart and lung doses are the primary constraints in left-sided breast radiotherapy for both conventional and hypo-fractionated radiotherapy. Radiation-induced cardiac toxicity is related to the mean dose received by the heart, and it is reported by many authors. Reference Taylor, Povall and McGale13,Reference Abouegylah, Braunstein and El-Din14,Reference Darby, Ewertz and McGale15 Few authors demonstrated that left anterior descending artery doses have more correlation with cardiac toxicity along with the heart mean dose. Reference Abouegylah, Braunstein and El-Din14,Reference Darby, Ewertz and McGale15,Reference Bergom, Rayan and Brown16,Reference Wang, Wei and Zheng17 Different techniques are in use to reduce the heart and lung doses effectively. One among them is DIBH technique.
The conventional field-in-field (FiF) technique is the standard delivery method of radiotherapy for breast cancer. Also, VMAT can deliver the treatment in time equal to the tangential fields and can increase the patient throughput compared to conventional IMRT. The introduction of flattening filter free (FFF) beams in linear accelerators showed the significant reduction of treatment time for stereotactic radiosurgery and stereotactic body radiotherapy. The modern-day high-performance computers are equipped with high-precision dose calculation algorithms which enable to create the complex modulation with FFF beams.
In the Lancet 2020, a phase 3 trial was published by Brunt et al., that a dose of 26 Gy delivered in 1 week (FAST-Forward trial) has the same effect as of 3 weeks hypo-fractionated therapy and has the advantage of reduced cost and time for patients as well as health care society. Reference Brunt, Haviland and Wheatley18 The dose constraints followed in this FAST-Forward trial are very stringent and achieving the same in FB radiotherapy is really challenging as planning target volume (PTV) is close to heart and lung. DIBH may be considered to overcome this issue. Hence, we attempted to demonstrate how the DIBH is beneficial for left-sided breast cancer in association with FFF VMAT.
Aims of this study are to determine the effect of DIBH on FAST-Forward trial for left-sided breast radiotherapy dosimetrically using tangential FiF technique and to evaluate the benefits of FFF-based VMAT (FFF-VMAT) against FF-based VMAT (FF-VMAT) for both FB and DIBH in comparison with FiF.
Methods and Materials
Patient characteristics
We selected a total of 15 patients with left-sided breast cancer prospectively for this study, who underwent breast conservative surgery with no positive nodes, who were registered in our department from March 2020 to November 2020. All these patients were able to adopt the DIBH procedure where the patient had to hold their breath for at least 15 seconds. All these patients were belonging to the age group of 35–60 years (mean 42 years). All were treated in DIBH condition using FFF VMAT.
Simulation
Patients were immobilised in supine position with arms raised above head using thermoplastic masks and vacuum cuisine bags. Two thermoplastic masks were prepared for each patient as per our institutional protocol. One was in normal FB condition and another one was in DIBH condition. Before the immobilisation process, all patients were coached for DIBH.
All patients underwent computed tomography (CT) simulation in same setup as of immobilisation for both FB as well as DIBH. CT scans of slice thickness 3 mm in both conditions were acquired in Siemens biograph 16-slice CT scanner (Siemens Medical Systems, Concord, CA, USA). DIBH scans were acquired using the Real-time Position Management™ (RPM) system (Varian Medical Systems, Palo Alto, CA, USA) using an infrared camera and reflector. In RPM, 5 mm breathing window level (BHW) was used with base amplitude value of 1 cm to 1·5 cm depending on patient’s breathing pattern as shown in Figure 1.
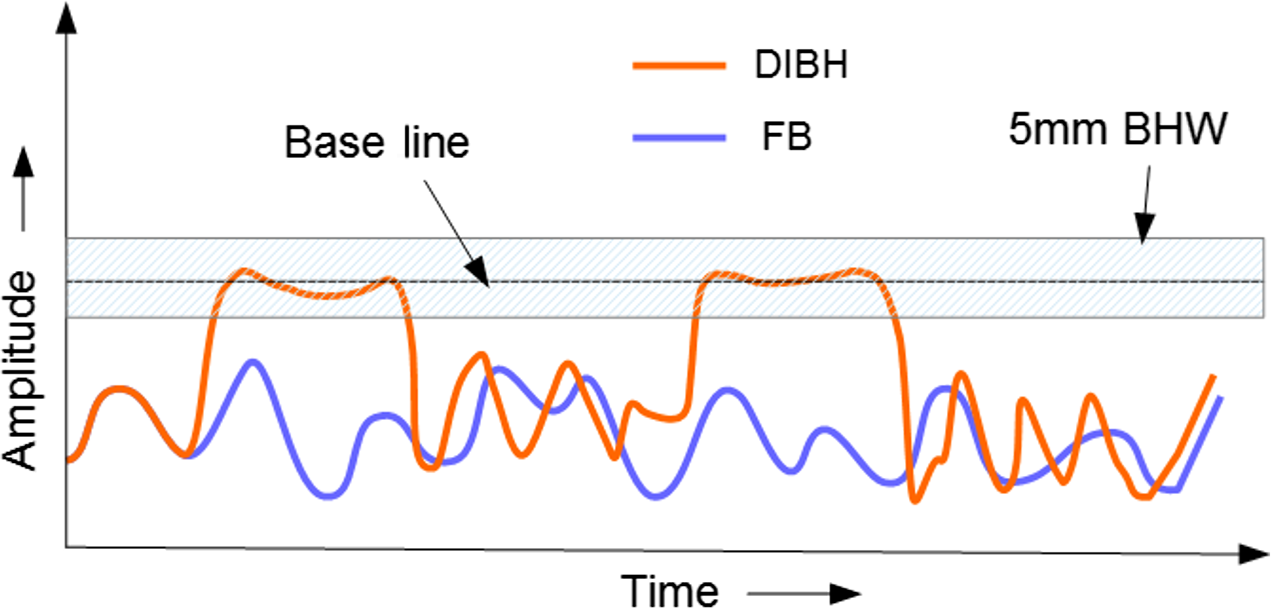
Figure 1. Schematic representation of breathing pattern in DIBH and FB.
Planning
A senior radiation oncologist contoured the breast PTV and other critical structures according to the radiotherapy oncology group breast contouring guidelines and also the FAST-Forward trial recommendation was adopted in PTV and organ at risk overlap region. Reference Brunt, Haviland and Wheatley18,19 All the structures were delineated on both DIBH and FB-based CT datasets in Eclipse (Varian Medical Systems, Palo Alto, USA) treatment planning system (TPS) version 11.0.
Dose of 26 Gy in five fractions with daily dose of 5·2 Gy was prescribed to the PTV as per FAST-Forward Trial. On each CT datasets in FB and DIBH, three plans (FiF, FF-VMAT, FFF-VMAT) were generated in Eclipse (Varian medical systems, Palo Alto, USA) TPS version 11.0. The volume dose computation was performed using anisotropic analytical algorithm (AAA) with calculation grid size of 2·5 mm. Dose constraints of all structures under study were given below as per FAST-Forward trial.
For PTV,
-
Volume receiving 95% of the prescribed dose (V95%) should be at least 90% while 95% is ideal
-
Volume receiving 105% of the prescribed dose (V105%) should not exceed at least 7% while 5% is considered ideal
-
Volume receiving 107% of the prescribed dose (V107%) should not exceed 2%
-
Maximum dose (Dmax) should not exceed 110% of the prescribed dose and preferably inside the PTV
For Heart,
-
Volume receiving 1·5 Gy (V1·5 Gy) should be less than 30%
-
Volume receiving 7 Gy (V7 Gy) should be less than 5%
For Lung Left,
-
Volume receiving 8 Gy (V8 Gy) should be less than 15%
For Normal Breast and Lung Right,
-
Mean dose as low as reasonably achievable
Field-in-field
FiF plans were created using two tangential fields (310°, 130°), and the sub fields were used to get the desired dose distribution in both FB and DIBH datasets as shown in Figure 2. The sub fields then merged with the actual fields having same geometric parameters except the MLC positions in order to reduce the mode up time required for each field. Either 6 MV photon beam alone or the combination of 6 MV and 15 MV beams were utilised depending on patient anatomy.

Figure 2. Field geometry arrangements and dose distribution on FB and DIBH CT datasets for FiF, FF-VMAT, FFF-VMAT plans in axial view for patient number 1.
Volumetric-modulated arc therapy
VMAT plans were generated using the progressive resolution optimizer-3 with 6 MV-FF and 6 MV-FFF, respectively, for FF-VMAT and FFF-VMAT in TPS. In step 1, FF-VMAT plans were generated using the upper and lower dose constraints depending on the structures in FB dataset. The same dose constraints were used to create the FFF-VMAT plan in FB condition.
In step 2, FF-VMAT plans were generated on the DIBH datasets using the upper and lower dose constraints. The same constraints were given as input to generate the FFF-VMAT plans in DIBH condition. Two complimentary partial arcs were used to create the VMAT plans in clock-wise (CW) and counter clock-wise (CCW) directions as shown in Figure 2. CW arc starts at 305° and ends at 145° with collimator rotation of 10°, while CCW arc starts at 145° and ends at 305° with collimator rotation of 350°. We used the priority rank 1 for PTV, 2 for heart, 3 for lung left, 4 for normal breast and 5 for lung right dose constraints for this study.
Dosimetric evaluation
Cumulative dose volume histograms and 3D dose distributions were used for evaluation of plans. For evaluation of PTV, a structure called PTV evaluation (PTV Eval) was created by reducing the PTV by 5 mm from the body surface. For PTV, V95%, V107%, maximum dose received by 0·1 cc (D0·1 cc) of the PTV, conformity index of 95% dose coverage (CI95) and homogeneity index (HI) were evaluated. CI and HI were calculated using the following formula. For heart, V1·5 Gy and V7 Gy were used to evaluate the plans, while for lung left was evaluated using V8 Gy. Lung left and normal breast volumes were analysed using mean dose (Dmean) received by them.


where V95% – volume covered by 95% – isodose line; VPTV – volume of the PTV; D5% – maximum dose received by 5% of the PTV volume; D95% – maximum dose received by 95% of the PTV volume.
Deliverability
Point and planar dose measurements were performed to analyse the deliverability of all plans. Point dose measurements were done using CC13 (IBA Dosimetry, Gmbh, Germany) ionisation chamber and polystyrene phantom RW3 (IBA Dosimetry, Gmbh, Germany) with dimensions (30 cm x 30 cm x 10 cm). Verification plans for plans were created on phantoms with same geometry as of actual plans. The variation between the measured dose in the point of measurements and the TPS predicted dose was calculated.
Planar measurements were done using I-MatriXX (IBA Dosimetry, Gmbh, Germany) array of detector with multicube phantom of dimensions [34 cm (w) x 31·2 cm (l) x 22 cm (h)]. 2D gamma index analysis (GIA) with 3% dose difference (DD) and 3 mm distance to agreement (DTA) criteria was used to compare the measured and TPS predicted dose map in the plane of measurement. Reference Low, Harms, Mutic and Purdy20 The total monitor units (MU) of all fields in each plan and beam ON time (time from first field beam ON to last field beam OFF) were analysed to compare the efficiency of delivery.
Statistical analysis
To find the statistical significance of difference between two sets of planning techniques or breathing conditions, we used the student’s paired t-test with 95% confidence level in Microsoft Excel version 2016. The null hypothesis set was that both were having same population mean. The probability value (p-value) of less than or equal to 0·05 is considered as significant difference from the hypothesis.
Results
Plans that satisfied the dose constraints closely in each technique were taken for the comparison. For patient number 1, the dose distribution achieved in all plans in FB and DIBH is shown in Figure 2. The average DVH of all 15 patients for the structures PTV Eval, Heart, Lung Left, Lung Right and normal breast is shown in Figure 3.

Figure 3. Average DVH of all 15patients under the study.
For each parameter of structures, a total of six plan data were acquired. Two groups were created based on FB and DIBH. There were three plans under each group based on planning techniques as FiF, FF-VMAT and FFF-VMAT for all 15 patients. The total average of all 15 patients for each parameter is reported in Table 1 as mean ± 1 standard deviation (SD).
Table 1. Dosimetric results of PTV and OARs of all 15 patients

Patient-specific quality assurance test was done using point and planar dose measurements. The variation between TPS predicted dose in point of measurement and the actual measured dose is tabulated in Table 1. The results of planar dose comparison using GIA with criteria 3% DD and 3 mm DTA are tabulated in Table 1. Also, the gamma analysis workspace of patient number 1 for all plans in DIBH is given in Figure 4. The plan efficiency parameters MU and beam ON time are given in Table 1.

Figure 4. Gamma analysis workspace for FiF, FF-VMAT, FFF-VMAT plans in DIBH.
Intra-group ((FiF versus FF-VMAT versus FFF VMAT) statistical analysis between different planning techniques and inter-group (FB versus DIBH) analysis between same planning techniques were done. The p-values for all parameters for inter- and intra-group analyses are given in Table 2.
Table 2. p-values of intra-group and inter-group analyses

Discussion
Hypo-fractionated radiotherapy wherever possible is the most desirable method of treatment in many anatomical sites and in current practice and especially in the pandemic situations like COVID-19. Whole-breast radiotherapy using hypo-fractionated radiotherapy dose regimens of 40 Gy in 15 fractions or 42·5 Gy in 16 fractions is the current standard of care for the treatment of early breast cancer. Reference Coles, Aristei and Bliss21 A phase-3 trial was conducted by Brunt et al. by comparing the 26 Gy delivered in five fractions over a period of 1 week (FAST-Forward trial) with 40 Gy in 15 fractions (START-B trial), and they found FAST-Forward trial was non-inferior to 40 Gy in 15 fractions over 3 weeks. Reference Brunt, Haviland and Wheatley18
In this study, the role of DIBH on FAST-Forward trial (26 Gy in 5 fractions over 1 week) for three different planning techniques (FiF, FF-VMAT, FFF-VMAT) were studied and compared with FB. We were able to achieve optimal clinical goals for PTV in DIBH for all plans as well as in FB. For parameter PTV V95%, FiF technique showed higher dose coverage compared to VMAT plans in both DIBH and FB. Intra-group comparisons showed significant differences between FiF and VMAT plans for FB (0·039, 0·022, respectively, for FF-VMAT and FFF-VMAT) and DIBH (0·017, 0·042, respectively, for FF-VMAT and FFF-VMAT) at the cost of conformity. With respect to CI in intra group, VMAT plans were better compared to FiF with p-value of 0·003 for both FF-VMAT and FFF-VMAT in FB condition while less than 0·001 for FF-VMAT and 0·002 for FFF-VMAT in DIBH condition. Considering the high dose per fraction, dose conformity can also be a factor affecting the treatment outcome. Homogeneity index for PTV showed no significant differences for both intra- and inter-group analyses. Statistically insignificant differences were noticed for all PTV parameters in inter-group analysis as shown in Table 2.
In DIBH, heart V7 Gy and V1·5 Gy were lesser than FB for both VMAT plans. The differences in heart V7 Gy were highly significant with p-value of 0·09, 0·03 for FF-VMAT (FB versus DIBH) and FFF-VMAT (FB versus DIBH), respectively. There were no significant differences for V7 Gy in intra-group analysis, which means it had no effect due to the planning technique but depends on the breathing condition. DIBH reduces the V7 Gy to heart by 26·1% for FiF, 40·9 % for FF-VMAT and 49·5% for FFF-VMAT compared to FB as shown in Table 1. At the same time, V1·5 Gy was very less for FiF plans compared to VMAT plans. Heart V1·5 Gy dose constraints were achieved better for FiF followed by FFF-VMAT and FF-VMAT in both FB and DIBH groups. DIBH had reduced the heart V1·5 Gy by 14·1% for FiF (0·382), 24·6% for FF-VMAT (0·010) and 30·86% FFF-VMAT (0·036). The dose constraints given for heart were hard to achieve in FB with conventional contouring adopted for FAST-Forward trial.
We found no significant differences for left lung in both intra- and inter-group comparison for all techniques. The gap generated between chest wall and heart by DIBH was filled with lung tissue and hence the benefit of increased lung volume was compromised by the increased movement into the treatment port. Reference Oechsner, Düsberg and Borm22 Normal breast and right lung showed better sparing in FiF than VMAT plans in both FB as well as DIBH. The inter-group analysis showed that the impact of DIBH was not statistically significant with respect to normal breast and right lung mean doses. The delivery parameters, point and planar dose measurements, showed good agreement with the planned dose and measured dose for all plans.
The MU were lower with FiF compared to VMAT plans [0·023 (FF-VMAT), 0·029 (FFF-VMAT) in FB; 0·015 (FF-VMAT), 0·025 (FFF-VMAT) in DIBH]. Beam ON time for FFF-VMAT was ∼11% less than FiF and ∼65% less than FF-VMAT for both FB as well as DIBH. High dose per fraction (5·2 Gy) and high-dose rate (1400 MU/minutes for FFF) make it possible to deliver the treatment in shorter time for FFF-VMAT plans. Hence, the time taken for DIBH gating can be well managed with FFF-VMAT plans with better conformity. Also, the heart to chest wall distance was reproducible in DIBH compared to FB. Reference Kenneth, Ulf, Kristina and Anders8 Hence, in DIBH, dose can be delivered as per plan to critical structures as well as PTV compared to FB. Reference Yamauchi, Mizuno, Itazawa and Kawamori23 This phenomenon should be weighed high when we are delivering high dose per fraction of few fractions (1–5 fractions).
The limitations of this study are the smaller sample size, accuracy of AAA dose calculation algorithm to account the gradient electron density of lung tissues. Reference Oechsner, Düsberg and Borm22,Reference Fogliata, Nicolini, Vanetti, Clivio, Winkler and Cozzi24 The combined use of DIBH and FFF-VMAT to deliver the FAST-Forward trial dose of 26 Gy in five fractions might be considered as a better option in situations like COVID-19 due to its ability to deliver the high dose precisely in shorter time. In future, we would like to elaborate this study using Monte Carlo and Accuros XB dose calculation algorithms along with different BHW levels.
Conclusion
It is evident that VMAT offers better conformal dose distribution than FiF technique for both breathing patterns. Among the VMAT plans, FFF-VMAT delivers the dose in less time as the dose per fraction is 5·2 Gy. Moreover, the DIBH offers significant reduction in heart dose with similar doses to left lung, right lung and normal breast. Thus, combination of DIBH and FFF-VMAT is the treatment choice in using the FAST-Forward trial dose and fractionation scheme for left-sided breast cancer.
Acknowledgements
None.