BACKGROUND: HPV AND CERVICAL CANCER
After more than 30 years since human papillomavirus (HPV) was detected in cervical cancer tissue [Reference Durst1], there is little doubt that persistent cervical infections by approximately 15 high-risk HPV (hrHPV) genotypes cause virtually all cervical cancer and its immediate precursor lesions, cervical intraepithelial neoplasia grade 3 (CIN3) and adenocarcinoma in situ (AIS) (‘cervical precancer’). HPV meets all Hill's criteria for causation [Reference Hill2]: strength, consistency, specificity, temporality, biological gradient, plausibility, coherence, experiment, and analogy.
The natural history of HPV and cervical carcinogenesis can be represented by a simple, causal schema composed of four, reliably measured stages: (1) HPV acquisition, (2) HPV persistence (vs. clearance), (3) progression to precancer, and (4) invasive cancer [Reference Schiffman3]. HPV infection is very common, perhaps universal, among sexually active populations but on a per infection basis is an uncommon cause of cancer. Most (~90%) HPV infections are benign and are cleared or controlled within 2 years; there is now evidence that some infections may become quiescent (latent) or undetectable [Reference Rositch4, Reference Strickler5], although the mechanism of HPV latency is probably immunological control and differs from that of herpes simplex virus. However, the fraction of HPV infections that become latent is unknown. The cancer risk for new HPV infections or recrudescent HPV infections from latency in older women is uncertain due to the low or absent hormones thought to contribute to the carcinogenic process [Reference Plummer, Peto and Franceschi6].
HPV16 and HPV18 cause approximately 70% of all cervical cancers, with HPV16 causing about 55–60% and HPV18 about 10–15% [Reference de Sanjose7–Reference Wheeler9]. Ten other hrHPV genotypes contribute incrementally to most of the remaining 30% or so of cervical cancer [Reference de Sanjose7–Reference Wheeler9] while a small percentage is attributable to other ‘borderline’ hrHPV or even low-risk HPV genotypes [Reference de Sanjose7–Reference Guimera10]. That is to say, there appears to be more of a biological continuum for HPV genotypes causing cervical cancer than a clear-cut distinction between carcinogenic and non-carcinogenic HPV [Reference Schiffman, Clifford and Buonaguro11]. Importantly, while the prevalence of specific HPV genotypes detected in cervical cancer tumour tissue vary somewhat, generally speaking the same HPV genotypes cause cervical cancer everywhere in the world with very little variation in their summary ranking of importance from region to region [Reference de Sanjose7]. Moreover, there is no evidence of a significant genetic predisposition for cervical cancer. Thus, HPV is an equal opportunity killer and the risk of cervical cancer is directly related to amount and timing of exposure to HPV infection [Reference Plummer, Peto and Franceschi6, Reference Maucort-Boulch, Franceschi and Plummer12], the likelihood of persistence per infection, and access to preventive services [Reference Freeman and Wingrove13]. The good news is that interventions that target HPV should be equally effective all over the world.
The key step in cervical carcinogenesis is overt, measurable hrHPV persistence, which even after a year or two strongly predicts the development of surrogates of cervical precancer, CIN3 or AIS [Reference Castle14, Reference Kjaer15]. Biologically, productive HPV infections, those that make more virus, become transforming but the exact mechanism(s) by which that occurs is not well understood. Epidemiologically, the longer an infection persists, the greater the risk for development of precancerous cellular changes in the epithelium and for the development of frank malignancy. At some unknown average duration, HPV persistence probably becomes synonymous with cervical precancer although the latter may go undetected and may even regress.
The transition between the precancer and micro-invasive cancer is imperfectly understood because of the less than perfect sensitivity of colposcopy and biopsy to detect, and errors in the pathological diagnosis of cervical precancer; this is especially so in the earliest and smallest precancerous lesions with low malignant potential that must arise from the persisting infection [Reference Schiffman and Rodriguez16]. If a small precancer is undetected, it may enlarge laterally and accumulate somatic genetic changes, both increasing its invasive potential. Finally, untreated precancerous lesions in older women (median age 38 years) – about 15 years after the earliest, smallest precancerous lesions can be found in the population by screening – have about a 30% risk of becoming invasive over the next 30 years [Reference McCredie17, 18]. The carcinogenic process for cancer to develop from incident HPV infection on average takes time – approximately 5–10 years at a minimum and 20–25 years on average. A recent statistical modelling analysis [Reference Vink19] estimated that the progression time from CIN2/3 to invasive cervical cancer was 23·5 years, an estimate that may have been affected by the inclusion of CIN2, which is known to be an equivocal diagnosis of precancer and may reflect diagnostic error in distinguishing between CIN1 and CIN3 [Reference Darragh20], resulting in a longer time interval.
High-risk HPV infection, especially HPV16, also causes a significant proportion of vaginal, vulvar, anal, penile, and head-and-neck (e.g. oropharyngeal) cancer. Taking all HPV-related cancers into consideration, HPV causes about 5% of all cancers globally [Reference Forman21] and approximately 3-3.5% by HPV16.
FIRST GENERATION OF HPV VACCINES: BI- AND QUADRIVALENT
The discovery of hrHPV as the necessary cause of cervical cancer has led to revolutionary advances in cervical cancer prevention, including prophylactic HPV vaccination. HPV vaccination has demonstrated high degrees of efficacy with maximum effectiveness, and by extension cost-effectiveness, that is guided by the underlying natural history of HPV infection and cervical carcinogenesis and the importance of infection prevention in young adolescents who are the least likely to have been exposed.
The viral capsid is 50–55 nm in diameter, and has an icosahedral symmetry of T = 7 [Reference Bernard22]. Each viral particle contains 360 copies of the major capsid protein L1 and 72 copies of the minor capsid protein L2, organized into 72 capsomere complexes of L1 pentamers and a single copy of L2 at the centre of the capsomere. Recombinant expression of the L1 gene, with or without L2, will self-assemble under the proper conditions into a virus-like particle (VLP) that resembles the naturally occurring capsid. The HPV L1 VLP constitutes current prophylactic HPV vaccines.
Efficacy and effectiveness
In 2006, the U.S. Food-and-Drug Administration (FDA) approved the first commercially available prophylactic HPV vaccine, Gardasil (Merck & Co., USA), for the primary prevention of infections by HPV16 and HPV18 as well as HPV6 and HPV11, the two HPV genotypes that cause ~90% of genital warts (Condyloma acuminata) [quadrivalent HPV vaccination (4vHPV)]. In 2007, the Centers for Disease Control and Prevention's Advisory Committee for Immunization Practices (ACIP) recommended routine HPV vaccination of women aged 9–26 years [Reference Markowitz23] and the American Cancer Society recommended routine vaccination of women aged 9–18 years [Reference Saslow24] with 4vHPV. In 2010, a second HPV vaccine for the primary prevention of infections by HPV16 and HPV18, Cervarix [bivalent HPV vaccination (2vHPV)] (GSK, Belgium), was approved by the FDA and recommended by the ACIP [25]. In addition, 4vHPV vaccination of males aged 9–26 years was U.S. FDA-approved in October of 2009 and subsequently recommended by the ACIP in 2010 [26]. A comparison of HPV vaccines is given in Table 1.
Table 1. Basic information for the three U.S. Food and Drug Administration-approved prophylactic HPV vaccines, Cervarix (2vHPV), Gardasil (4vHPV), and GARDASIL 9 (9vHPV).
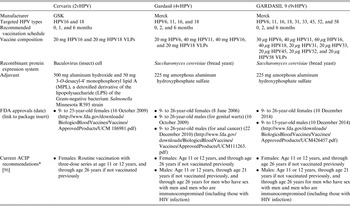
VLP, Virus-like particle.
* The vaccination series can begin at age 9 years.
These approvals and recommendations by all licensing and regulatory agencies were based on the overwhelming evidence from the pivotal clinical trials for FDA licensure. In these trials, in the according-to-protocol (AP) analysis, both 4vHPV [Reference Kjaer27, Reference Munoz28] and 2vHPV [Reference Lehtinen29, Reference Herrero30] demonstrated near perfect efficacy (>90%) in preventing infections and related abnormalities and precancerous lesions (CIN2, CIN3, AIS) caused by the vaccine-targeted HPV genotypes in women naive to those same HPV genotypes. High-grade CIN lesions were used as the surrogate endpoint for cancer prevention because the rarity of cancer in a young adult populations and it would have been unethical not to screen and treat precancerous lesions and allow them to progress to cancer. In the whole cohort of enrolled women, the intention-to-treat (ITT) analysis, the efficacy was significantly lower. The AP and ITT analyses of 4vHPV and 2vHPV for the prevention of incident, targeted HPV type-related cervical intraepithelial neoplasia grade 2 or higher (CIN2+) diagnoses are summarized in Figure 1.

Fig. 1. A comparison of vaccine efficacy against targeted HPV type-related (purple bars) and all cervical precancer (CIN2, CIN3, or AIS) (orange bars) by 2vHPV [Reference Lehtinen29] and 4vHPV [Reference Munoz28], for the HPV-naive populations (TVC-naive and ATP, respectively) and the entire vaccinated cohort (TVC and ITT, respectively). TVC, total vaccine cohort; ATP, attention to protocol; ITT, intention to treat.
The difference between AP and ITT results is primarily because 4vHPV [Reference Garland31] and 2vHPV [Reference Hildesheim32] do not treat pre-existing HPV infections and related conditions. Thus, the proportion of women who benefit from HPV vaccination decreases as more women are exposed to HPV infections, the likelihood of which increases with increasing age (Fig. 2), which is a proxy for time since sexual initiation. HPV vaccination will still provide protection against incident targeted HPV infections and related disease in sub-groups uninfected by any of the target HPV types but population effectiveness and therefore the cost-effectiveness decreases with increasing age of vaccination [Reference Kjaer27, Reference Herrero30]. Thus efficacy approximately equals population effectiveness in HPV-naive populations whereas efficacy is greater than population effectiveness in sexually active women, the difference of which is a function of total exposure to infection by the targeted HPV types. As the median age of sexual initiation is 15–17 years in many populations, the World Health Organization (WHO) recommends vaccination programmes to target 9- to 13-year-old girls [33], and CDC recommends routine HPV vaccination for females and males aged 11–12 years [26].

Fig. 2. A comparison of efficacy against targeted HPV type-related cervical precancer (CIN2, CIN3, or AIS) by 2vHPV [Reference Herrero30] and 4vHPV [Reference Kjaer27] for the HPV-naive populations (TVC-naive and ATP, respectively) and the entire vaccinated cohort (TVC and ITT, respectively), stratified by age group [<17 years (blue bars), 18–20 years (red bars), and ⩾21 years (green bars)]. TVC, total vaccine cohort; ATP, attention to protocol; ITT, intention to treat.
It is not known is whether vaccination of persons who are already infected by the targeted HPV genotypes blocks transmission to their partners, as the result of the infectious virions bound with neutralizing antibodies. Even among dually infected partners, neutralizing antibodies could break the cycle of reinfection, reducing the residence time of infection in a sexual couple, and also reducing the risk of transmission to other partners outside of the pair. If so, there could be additional benefit to vaccinating pre-exposed populations that could not be addressed in the efficacy trials that dealt only with direct impact of HPV vaccination. For example, studies of HPV vaccination in partners of couples and in sexual networks could examine its impact on infection transmission and dynamics in a population.
There is evidence that 2vHPV and perhaps 4vHPV induce cross-protection against non-target HPV genotypes. Clinical trials of 2vHPV have shown a consistent and significant effect against other HPV genotypes, especially HPV31 and HPV45 [Reference Wheeler34]. These two non-targeted HPV genotypes are the two HPV genotypes most closely genetically related to HPV16 and HPV18, respectively, adding biological plausibility to the observed effect. Protection against these untargeted HPV types appears to last for 4–5 years [Reference Malagon35]. There is also some evidence of a weak cross-protective effect of 2vHPV vaccination against HPV6 and HPV11 [Reference Szarewski36], the two additional types targeted by Gardasil. The cross-protective effect of 4vHPV vaccination against HPV31, 33, and 45 appears to be weaker than 2vHPV although the differences do not appear to be significant and may be due to trial design [Reference Malagon37]. Recent population data from Australia suggest that there is a real and measurable effect of Gardasil vaccination on non-targeted HPV genotypes in the general population [Reference Tabrizi38].
One of the criticisms regarding the evidence for benefit of HPV vaccination is the lack of data on its direct impact on the incidence of invasive cervical cancer. It was not practical or ethical to have clinical trials for cervical cancer endpoint and time since the implementation of HPV vaccine has not been long enough to see declines in cervical cancer in the general population yet. However, it is worth noting that there has never been a clinical trial to show directly that Pap testing reduces the incidence of cervical cancer and yet there is little doubt that effective implementation of Pap testing, with timely and effective management and treatment, reduces its incidence. If Pap testing prevents cervical cancer by the timely detection and then treatment of cervical precancerous lesions, and Pap testing was used to measure the reduction in cervical precancerous lesions caused by HPV vaccination, it stands to reason that HPV vaccination reduces cervical cancer risk and will eventually reduce cervical cancer incidence. HPV vaccination has already led to population reductions of Pap-detected CIN2/3 [Reference Baldur-Felskov39–Reference Hariri41].
Another misconception about HPV vaccination is its relative benefit in a given population. The benefit of HPV vaccination using either HPV2 or HPV4 vaccines relates to the prevalence of HPV16 and HPV18 in cancers, not their infection prevalence in specific populations. A number of reports have mistakenly concluded that HPV vaccination will be less effective in specific populations based on studies of HPV16 and HPV18 prevalence in the general population or even in small studies of cervical precursor abnormalities [Reference Vidal42]. What matters is the prevalence in cervical cancers since the goal ultimately is cancer prevention and the aforementioned large international study [Reference de Sanjose7] shows that populations are largely alike with respect to the attributable fraction of HPV16/18 in cervical cancer. So consistent is this attributable fraction in cervical cancer from the general (HIV-uninfected) population that it probably does not need to be studied further, i.e. evaluated from country to country. However, the prevalence of these types in the general population and women with precancerous lesions does inform on the impact of HPV vaccination on reducing the number of women referred to colposcopy or getting treatment for CIN2/3, respectively, where screening is available.
Humoral immune responses
Immunogenicity studies have shown that:
-
(1) HPV VLPs invoke a strong humoral immune response, with antibody titres that are typically 10- to 100-fold greater than those induced by natural infection [Reference Safaeian43, Reference Villa44].
-
(2) Immunization at younger age results in higher antibody titres than in older age and the age-specific antibody titres remain higher with immunization at younger ages than older ages (e.g. the titres of 17-year-olds vaccinated at the age of 12 years are higher than those 17-year-olds vaccinated at the age of 15 years) [Reference Block45–Reference Dobson48].
-
(3) Antibody titres are slightly higher in males than females [Reference Block45, Reference Petaja49].
-
(4) 2vHPV, due presumably to its adjuvant, induces greater antibody titres vs. HPV16 and HPV18 and invokes higher titres of cross-protective antibodies against untargeted HPV genotypes than 4vHPV [Reference Einstein50–Reference Draper54].
-
(5) More doses increase the antibody titres although the differences between two and three doses are less pronounced than between one and 2 doses [Reference Safaeian43, Reference Dobson48, Reference Villa55].
-
(6) Antibody titres appear to be lower for HPV18 than for HPV16 [Reference Safaeian43, Reference Villa55], although it is unclear whether this is a qualitative/quantitative difference in responses or a difference due to the type-specific assays.
-
(7) For 2vHPV and 4vHPV, antibody titres plateau a year or two after the peak titres following immunization, and to date remain fairly stable 8–9 years after immunization, well above those antibody responses resulting from natural infection, and continue to protect against incident infection by targeted HPV genotypes [Reference Ferris56, Reference Naud57]. However, a direct comparison of seropositivity and antibody titres for the two vaccines after 60 months show that 100% of women vaccinated with 2vHPV remain seropositive whereas only ~66% of women vaccinated with 4vHPV remain seropositive; furthermore, among the seropositives, the titres remain higher following 2vHPV than 4vHPV vaccination [Reference Takacs58].
There is no clearly identified threshold for protection, in part due to the lack of breakthrough incident events following HPV vaccination in clinical trials with serological measures. However, the much lower, naturally induced antibody titres provide partial protection [Reference Wentzensen59–Reference Castellsague61], which suggests that antibody titres are directly involved with, or are a surrogate of, protection and that there is a threshold effect. The correlation between cross-protection against incident HPV31 and antibody titres following immunization by 2vHPV also supports these conclusions [Reference Safaeian62].
Immune responses to HPV vaccination appear robust to different immunization schedules. Different vaccination schedules do not appear to greatly alter the overall immune response [Reference Safaeian43, Reference Neuzil63]. Given that there is some evidence for at least partial protection following one or two doses of HPV vaccination [Reference Kreimer64, Reference Herweijer65], timing of HPV vaccine doses can be tailored based on school schedules or other considerations to increase coverage and compliance that will likely offset the negligible or no impact on the population effectiveness due to different scheduling.
In addition, co-administration of HPV vaccines with other important vaccines, such against hepatitis A, hepatitis B, meningococcal vaccine, or tetanus, diphtheria, and acellular pertussis (TDAP) vaccine, does not result in inferior immune responses to HPV compared to HPV vaccination alone [Reference Noronha, Markowitz and Dunne66]. Based on these data, it is reasonable to suggest that co-administration of HPV vaccine with other adolescent vaccines would be highly effective. Given how long it would take to show efficacy following childhood (vs. adolescent) HPV vaccination even against persistent HPV infection, it seems likely that immunogenicity/safety bridging studies will have to suffice as evidence of efficacy for co-administration of HPV vaccine with other child vaccines, as it did in young adolescents leading to recommendations of HPV vaccination in 9- to 15-year-olds who were not included in the efficacy trials.
Duration of protection
The duration of protection remains an outstanding question that may influence the decision about whether to vaccinate. There is now evidence that an experimental monovalent HPV16 vaccine administered in early trials [Reference Rowhani-Rahbar67], and 2vHPV [Reference Naud57] and 4vHPV [Reference Ferris56] in more recent trials resulted in durable immune responses with antibody titres significantly above those resulting from natural infection and prevent incident infection for at least 8–9 years. It seems unlikely that the clinical trials will provide sufficient follow-up data on duration. Registries and nested population-based studies that allow linkage of administrative data of HPV vaccination and screening outcomes may be the only way to answer the question.
The complementary question is how long does the protection need to last to achieve a high-level of population effectiveness? It may take several generations’ undergoing HPV vaccination to answer this question directly. However, a hypothetical exercise can be undertaken to estimate a minimum duration of protection for maximum population effectiveness. In most populations, the HPV prevalence curve peaks about 5 years after the median age of sexual initiation in a population; it then declines and levels off 5–10 years after that. If all prevalently detected infections were incident, the majority of the cancer-causing infections would therefore be acquired in that first 10–15 years after the median age of sexual initiation. However, prevalent infections in older women are increasingly likely to be due to persistent HPV infection [Reference Castle68] and not preventable by prophylactic HPV vaccination, which suggests that even a greater proportion of causal infections occur in that 10- to 15-year window of time following sexual initiation. Thus, if HPV vaccination occurs approximately 5 years before the median age of sexual initiation, prophylactic HPV vaccines would likely need to protect for about 20 years to achieve most of its population effectiveness.
In populations where the prevalence of HPV increases with age [Reference Franceschi69], it is unclear whether these HPV infections are truly incident, emerging latent, persistent infection, or a mixture. Also it is unclear whether the elevated prevalence of HPV infection in older women correlates with cancer risk, although generally speaking HPV prevalence does correlate with population risk of cervical cancer [Reference Maucort-Boulch, Franceschi and Plummer12]. Thus necessary duration of protection in these populations is unknown and population-based cohorts in these populations are needed to understand the natural history of these infections in older women.
Finally, it is unknown whether natural exposure to targeted HPV genotypes after vaccination boosts immunity. Seropositive populations have higher antibody titres in response to HPV vaccination than seronegative populations [Reference Villa44, Reference Giuliano47]. If subsequent exposure after immunization serves as a booster, duration of protection may be prolonged, especially in those engaged in higher-risk behaviours such as having more sexual partners and therefore more exposure to HPV and therefore are more likely to need protection.
The Australian experience
Australia was the first country to implement a government-funded, population-based HPV vaccination programme and has served as an exemplar for how HPV vaccination can rapidly reduce the burden of HPV-related disease in the general population. In April 2007, following a technical review by Australian Technical Advisory Group on Immunisation (ATAGI) and cost-effectiveness evaluation by the Pharmaceutical Benefits Advisory Committee, the National HPV Vaccination Programme of Australia launched a school-based HPV vaccination programme using 4vHPV in females aged 12–13 years. From July 2007 until December 2009, there was also a catch-up HPV vaccination programme using 4vHPV for all females aged 14–26 years. Since 2013, males have similarly been eligible for routine vaccination at age 12–13 years, with a 2-year catch-up programme for males aged 14–15 years.
By 2012, before the introduction of HPV vaccination in males, one-, two-, and three-dose HPV vaccination coverage achieved in the school programme (age 12–17 years) was 83%, 78%, and 70%, respectively, and for women aged 18–26 years in the community catch-up programme was 55%, 44%, and 32%, respectively. Consequently, Australia has already observed declines in HPV infection and disease. There have been rapid declines in HPV prevalence of the target HPV genotypes [Reference Tabrizi70], high-grade cervical abnormalities [Reference Gertig40, Reference Crowe71], and genital warts [Reference Read72, Reference Donovan73] in the general population. The greatest declines have been observed in younger women who are the most likely to be vaccinated and least likely to have been pre-exposed to targeted HPV types.
Notably, new diagnoses of genital warts have declined substantially in attendees at sexual health clinics across Australia, with a 93% decline in young women aged <21 years between 2007 and 2011. The observations on the population impact of HPV vaccination in Australia have been confirmed for the incidence of cervical precancer in Denmark [Reference Baldur-Felskov39], genital warts in Sweden [Reference Herweijer65], and HPV prevalence [Reference Markowitz74] and high-grade cervical disease [Reference Hariri75] in the United States. A recent systematic review and meta-analysis of the population effects of HPV vaccination has shown consistent reductions in the prevalence of HPV16 and HPV18 and anogenital warts in females aged <20 years [Reference Drolet76]. In populations with high coverage (>50%) of HPV vaccination, there have also been reductions in other HPV types in females aged <20 years due to cross-protection, and anogenital warts in males aged <20 years [Reference Drolet76].
In addition to the reductions in genital warts in young vaccinated women living in Australia, there has been a concomitant 82% decline in genital warts in age-matched heterosexual males, suggesting herd protection, while no reductions have been observed in age-matched heterosexual men aged ⩾30 years or any homosexual males [Reference Ali77]. The inference is that there is a significant direct effect of HPV vaccination on the incidence of genital warts in the young women targeted for HPV vaccination and an indirect effect due to herd protection in their male sexual partners. There is now evidence that unvaccinated women of the same cohort of women living in Australia have a lower prevalence of targeted HPV genotypes presumably due to herd protection [Reference Tabrizi38].
Male vaccination
The efficacy and safety of 4vHPV was also evaluated in 4065 men [3463 heterosexual and 602 men who have sex with men (MSM)], aged 16–26 years [Reference Giuliano78, Reference Palefsky79]. Within 1 month after the third dose of vaccine, >97% of participants randomly assigned to the vaccine arm seroconverted for the targeted HPV genotypes. The vaccine was highly effective in the HPV-naive, AP population, with a vaccine efficacy for genital lesions related to HPV6, 11, 16, or 18 of 90%. The vaccine efficacy in the ITT population was significantly lower at 66% as would be expected. The vaccine efficacy for prevention of anal intraepithelial neoplasia (AIN) in the MSM population was 78% in the AP population and 50% in the ITT population. The vaccine efficacy for preventing persistent anal HPV infection by the targeted HPV genotypes was 95% in AP population and 59% in the ITT population.
Protection at non-genital sites
Nested studies within the U.S. National Cancer Institute's (NCI) Costa Rica Vaccine Trial (CVT) were conducted to study the impact of 2vHPV on prevalent anal [Reference Kreimer80] HPV16/18 at the 4-year visit at the end of the main trial. In the AP population, women who received 2vHPV vaccination had an 85% reduction in the prevalence of anal HPV16/18 infection vs. those who received hepatitis A vaccine placebo, a reduction in prevalence that was comparable to what was observed for cervical HPV16/18 [Reference Kreimer80]. These data are consistent with the aforementioned clinical trials showing that 4vHPV prevents anal HPV infection in MSM.
Similarly, HPV vaccination was highly effective in reducing the prevalence of oral HPV16/18 at 4 years after vaccination compared to those who received the hepatitis A vaccine placebo [Reference Herrero81]. Taken together, HPV vaccination induces systemic, neutralizing antibodies that through secretion and exudation following disruption of the mucosal barrier [Reference Lowy and Schiller82] suffuse/cover all mucosal surfaces and protect against incident HPV infection.
Immunosuppressed populations
There has been interest in evaluating HPV vaccines in immunosuppressed populations because of their elevated risk of HPV-related cancers [Reference Grulich83]. Immunogenicity and safety of 4vHPV has been evaluated in HIV-infected populations [Reference Giacomet84, Reference Wilkin85] and transplant populations [Reference Gomez-Lobo86, Reference Kumar87]. A trial of immunogenicity and safety of 2vHPV in HIV-infected females is underway [88]. To date, there are no trials for efficacy in either population registered at clinicaltrials.gov. It would be useful to know whether HPV vaccination by either or both vaccines reduces cancer risk in HIV-infected populations and whether the degree of immunosuppression influences protection. This is a particularly relevant question in some low-resource settings where there may be less access to antiretroviral therapy (ART). It may be useful to treat the HIV infection and partially reconstitute the immune system prior to vaccination, and once vaccinated, maintenance of ART may sustain protection against HPV [Reference Okwen89].
Number of doses
There are now data demonstrating that fewer than three doses of 2vHPV and 4vHPV provide at least partial protection against incident HPV infection and related disease. In a post-hoc analysis in the United States. NCI's CVT, one, two, and three doses of 2vHPV resulted in equal protection against incident, 1-year or longer persistent HPV16/18 infection over the 4-year period of the trial [Reference Kreimer64]. These results for 2vHPV were confirmed in a second population [Reference Kreimer90].
In an open cohort of 1 million women aged 10–24 years followed-up for 6 months and living in Sweden [Reference Herweijer65], the risk of genital warts decreased by ~40% with each dose of HPV vaccination (0 vs. 1, 1 vs. 2, 2 vs. 3), with an overall reduction of 80% by three doses of 4vHPV vs. none. However, it has been argued [Reference Kreimer, Safaeian and Hildesheim91] that the risk reduction for condyloma by number of doses was the same after a 12-month buffer period, perhaps suggesting that there was a bias in that those who had fewer doses were more likely to have prevalent infections.
Yet, the difference in the immune response between the first and second doses is much greater than the second and third doses, and it seems likely that two doses will be needed to ensure effective long-term protection, if antibody titres are a measure of protection and/or durability, which has yet to be demonstrated. Using a 6-month interval vs. a 1-month interval between the first and second dose for 2vHPV increased antibody titres [Reference Safaeian43], and a 6-month interval vs. a 2-month interval between the first and second dose of 4vHPV provided between protection against genital warts [Reference Blomberg92]. There may also be differences in antibody avidity with time and presumably with number of doses but one study did not find that antibody avidity was strongly correlated with in vitro neutralization, so the importance of avidity in immune protection is not well understood [Reference Kemp93].
Under the assumption that antibody titres are important, younger age at vaccination and the AS04 adjuvant in the 2vHPV vaccine may meaningfully increase antibody titres compared the alum adjuvant in 4vHPV [Reference Takacs58] and make a single dose of HPV vaccine more effective. Clinical trials may be needed to determine the long-term effectiveness of a single dose for any of the vaccines but such a trial cohort will need to be large and followed long enough to demonstrate with sufficient statistical power that a single dose provides durable protection beyond the few years of protection already demonstrated. However, by the time these data are available, there may be sufficient evidence based on observational data from trials [Reference Kreimer80, Reference Kreimer90] and vaccine programmes to answer this question.
Indeed, the WHO already has endorsed the use of two-dose HPV vaccination to increase compliance with and reduce costs of HPV vaccination [94]. Prior to the WHO recommendation, several countries such as Mexico and Chile have adopted strategies of delaying the third dose until 60 months, with the idea that the first two doses are critical and the third dose ultimately may not be necessary; in 2015, Mexico will switch to a two-dose schedule [95]. The balance between effectiveness vs. dose regimen remains unresolved as does the question of whether fewer doses in more young women is a better public health intervention than all doses in fewer women. Modelling may be needed to determine the most cost-effective distribution of HPV vaccine doses when three or even two doses per person are not universally achievable.
Adverse events/safety
The WHO [33], ACIP [Reference Petrosky96], National Advisory Committee on Immunisation (NACI) (Canada) [97], ATAGI [98], National Health Service (NHS) (UK) [99], and other regulatory agencies continue to recommend HPV vaccination because it is effective, cost-effective, and safe.
The safety of HPV vaccination has been evaluated carefully in several countries that have implemented such programmes. In nearly 300 000 women aged 10–17 years living in Denmark and Sweden who received approximately 700 000 doses of the vaccine [Reference Arnheim-Dahlstrom100], there was no evidence of increased risk of autoimmune events, neurological events, or venous thromboembolism. In Ontario, Canada, which started school-based HPV vaccination in 8th grade girls, 133 confirmed adverse events following immunization (AEFI) were reported while 691 994 HPV4 vaccine doses were distributed in the school-based programme during 2007–2010 [Reference Harris101], a rate of 1·9/10 000 doses. Primarily, the AEFI were ‘allergic reaction–dermatologic/mucosa’ (25%), ‘rash’ (22%), and ‘local/injection site reaction’ (20%), while 26% of reports had a non-specific event of ‘other severe/unusual events’ selected. Of the 10 serious AEFI reported, only seven were confirmed and attributable to HPV vaccination.
In the United States, a study of adverse events in women aged 9–26 years receiving healthcare at one of seven managed care organizations, revealed that there was no increased risk of Guillain–Barré Syndrome (GBS), stroke, appendicitis, seizures, syncope, allergic reactions, and anaphylaxis and only a slight, non-significant increase in venous thromboembolism (VTE) [Reference Gee102]. The CDC reported based on the Vaccine Adverse Event Reporting System that, from June 2006 to March 2013, approximately 56 million doses of HPV vaccination were distributed in the United States, resulting in 21 194 AEFIs; 92% were non-serious and most commonly were syncope (fainting), dizziness, nausea, headache, fever, and urticaria (hives) [103]. Of the 8% that were considered serious AEFIs, headache, nausea, vomiting, fatigue, dizziness, syncope, and generalized weakness were the most frequently reported symptoms.
As stated by the WHO's Global Advisory Committee on Vaccine Safety (GACVS) on 12 March, 2014 [104]:
the GACVS continues to closely monitor the safety of HPV vaccines and, based on a careful examination of the available evidence, continues to affirm that its benefit-risk profile remains favourable. The Committee is concerned, however, by the claims of harm that are being raised on the basis of anecdotal observations and reports in the absence of biological or epidemiological substantiation. While the reporting of adverse events following immunization by the public and health care providers should be encouraged and remains the cornerstone of safety surveillance, their interpretation requires due diligence and great care. As stated before, allegations of harm from vaccination based on weak evidence can lead to real harm when, as a result, safe and effective vaccines cease to be used.
SECOND GENERATION: NONAVALENT
On 10 December 2014, the U.S. FDA approved a second-generation vaccine, GARDASIL 9 (9vHPV), which targets five additional HPV genotypes: HPV31, 33, 45, 52, and 58, in addition to types 6, 11, 16 and 18 [105]. The FDA approval of 9vHPV was based primarily on data from five clinical trials, including a randomized clinical trial in approximately 14 000 women aged 16–26 years comparing 9vHPV to 4vHPV [Reference Joura106]. Key findings for 9vHPV vs. 4vHPV included: (1) 97% reduction in the combined incidence of high-grade cervical, vulvar, and vaginal disease caused by HPV types 31, 33, 45, 52, and 58; (2) non-inferior seroconversion and geometric mean antibody titres for HPV6, 11, 16, and 18; (3) numerically higher rates of injection site reactions; and (4) immunobridging studies of 9- to 15-year-old adolescent girls and boys showed non-inferior seroconversion and geometric mean antibody titres for all nine HPV types (6, 11, 16, 18, 31, 33, 45, 52, 58), compared to 16- to 26-year-old women [105]. Long-term follow-up studies are ongoing to access immunogenicity, effectiveness, and safety of 9vHPV.
The ACIP [Reference Petrosky96] recently made the following recommendations for the use of 9vHPV. ACIP recommended that routine HPV vaccination is initiated at age 11 or 12 years but can be started as young as age 9 years for females and males. Vaccination is also recommended for females and males aged 13–21 and women aged 22–26 years who have not been vaccinated previously or who have not completed the three-dose series. Males aged 22–26 years may be vaccinated. Vaccination of females is recommended with 2vHPV, 4vHPV (as long as this formulation is available), or 9vHPV. Vaccination of males is recommended with 4vHPV (as long as this formulation is available) or 9vHPV. The ACIP recommended the use of 9vHPV in males without direct clinical evidence of protection but based on the data from the 4vHPV trials in men [Reference Giuliano78, Reference Palefsky79] and the similarities in the natural history of HPV infection in the cervix and the anus.
An open-label, randomized trial in 11- to 15-year-old males and females of 9vHPV with co-administration vs. a 1-month delay in vaccination with a diphtheria, tetanus, acellular pertussis, and inactivated poliomyelitis vaccine demonstrated similar rates of adverse events and non-inferiority in immunogenicity for all vaccinogens [Reference Kosalaraksa107]. Like the first-generation HPV vaccines, 9vHPV might be co-administered with other childhood vaccines, potentially simplifying the delivery of HPV vaccination.
THIRD GENERATION: L2-BASED VACCINES
Immunization with L2 peptides or proteins elicits cross-reactive, neutralizing antibodies [Reference Roden108–Reference Gambhira111], making a L2-based immunogen a promising candidate for a broad-spectrum, pan-HPV vaccine. Moreover, because a L2 vaccine would not be based on the relatively complex HPV VLP production, a L2-based vaccine might be easier and cheaper to produce, thereby potentially becoming more readily accessible to lower-resourced populations who need prophylactic HPV vaccination the most.
However, the major challenge in developing a L2-based vaccination has been in eliciting a strong immune response to L2. While L2 and L1 can be recombinantly co-expressed and will assemble into a HPV VLP, L2 is immunologically subdominant to L1 [Reference Roden108], as is evident from the lack of L2 seropositivity in the general population. Several approaches have been taken to enhance immunity: (1) recombinant expression of a L2 fusion construct composed of the neutralizing epitopes for multiple HPV genotypes [Reference Jagu112], (2) display on the HPV L1 VLP [Reference Varsani113, Reference Schellenbacher, Roden and Kirnbauer114], (3) adeno-associated virus type 2 VLP [Reference Nieto115, Reference Nieto116], (4) tobacco mosaic virus VLP [Reference Varsani117, Reference Smith118], and (5) Qβ bacteriophage [Reference Tyler119, Reference Tyler120]. While many of these strategies are promising, none are yet in clinical trials according to clinicaltrials.com, suggesting that any of these candidate HPV L2-based vaccines are at least 10 years from being available for general use. Given that HPV L1 VLP-based vaccines are highly efficacious, will have an almost a 20-year head start in their introduction, and are becoming increasingly more affordable, the question then becomes whether a promising L2-based vaccine will ever be commercially viable.
WHO SHOULD GET VACCINATED AND WHY
It is increasingly apparent that HPV vaccination is highly preventative for incident infection and related disease by targeted HPV genotypes in adolescent and adult females, adolescent males, and probably adult males. That is, efficacy is very high in any group of HPV-naive people. So who should get vaccinated? Clearly, population effectiveness decreases with increasing age, as a proxy for previous exposure, and women experience a greater direct burden of HPV-related cancers than men. Therefore, cost-effectiveness analyses and resource utilization should be the guiding lights. Comparisons of the cost-effectiveness consistently show that HPV vaccination is more cost-effective in adolescent females than in adult women [Reference Burger121–Reference Tsu, Murray and Franceschi123] or in adolescent males [Reference Burger124–Reference Brisson127]. There have been no analyses of adult females vs. adolescent males to guide the next highest priority. In our opinion, the order of vaccination priority should be adolescent females, adolescent males, adult females, and finally adult males.
In high-resource settings, there will be an inclination towards vaccinating more rather than fewer people. Ideally, for every sexual pairing, one person (vs. 0 or 2) will be effectively vaccinated to achieve good and rapid population effectiveness and cost-effectiveness. It is clear from the Australian experience that 80% coverage achieves rapid direct effects and subsequent indirect effects via herd protection within few years. In the context of high coverage in females, adding male vaccination will likely increase effectiveness but only incrementally. It likely will accelerate the population impact and reach subgroups, such as MSM, who may not glean the benefits of herd protection from vaccinating only females. It is more cost-effective to increase coverage in females than including males [Reference Burger124], such that up to US$350 per additional female vaccinated could be invested, nearly doubling the cost of vaccinating females, and it would be still more cost-effective than vaccinating males [Reference Chesson128]. It is worth noting that cross-protection in women and herd protection of males may be minimal when coverage of females is <50% [Reference Drolet76].
9vHPV vaccines, because the cancer prevention benefits will be increased, should will be slightly more cost-effective at all ages and genders than 4vHPV, provided that their long-term safety and durability are comparable and the cost is similar to 4vHPV. However, the relative cost-effectiveness between young vs. old and women vs. men should remain similar, perhaps favouring female over male vaccination a bit more than the first-generation vaccines, since the other hrHPV genotypes contribute more to causing cervical cancer than to causing HPV-related cancers at other anatomical sites found in men and women. As noted by the ACIP [Reference Petrosky96],
Introduction of 9vHPV in both males and females was cost saving when compared with 4vHPV for both sexes in a cost-effectiveness model that assumed 9vHPV cost $13 more per dose than 4vHPV … . Because the additional five types in 9vHPV account for a higher proportion of HPV associated cancers in females compared with males and cause cervical precancers, the additional protection from 9vHPV will mostly benefit females.
The decisions if and who to vaccinate are local and should be based on cancer burden, cost-effectiveness, and available resources. A major debate of whether men should get vaccinated remains unresolved. Given the rapid impact of 4vHPV vaccination of females on genital wart rates in men living in Australia, it seems unlikely that HPV vaccination of men by any HPV vaccine is good value when there is excellent coverage in women, unless the HPV vaccine for men is deeply discounted to reflect its lesser health benefit. Such was the case for Australia [Reference Conway and Regan129]. One approach that might balance protection and cost is to vaccinate all eligible women and target high-risk subgroups of men, such MSM and those infected with HIV, especially in the former if HPV vaccination reduces the reservoir of HPV in this male subgroup by blocking transmission.
Populations that are underserved by current cervical cancer screening programmes may be less likely to receive HPV vaccination, as has been observed in the United States [Reference Jemal130]. As a result, cancer health disparities may widen with the introduction of HPV vaccination. If those populations are isolated socially or geographically, they may not benefit from the herd protection experienced by the general population as observed in the MSM population living in Australia. Programmes that specifically target these populations, who experience a disproportionate burden of cervical cancer, may have the greatest potential to reduce the cervical cancer burden in high-resource setting [Reference Scarinci131]. For example, mother–daughter campaigns could be developed to screen the mothers and vaccinate the daughters, with the notification of the screening results and management of positive results for the mothers being done in conjunction with the second dose of vaccine being given to their daughters.
SCREENING IN THE HPV VACCINATION ERA
Although prophylactic HPV vaccination may be the ultimate cervical cancer prevention strategy, current vaccines prevent infections but do not treat pre-existing infections and conditions [Reference Garland31, Reference Hildesheim32]. Therefore, even if universal female HPV vaccination could be rapidly deployed, there would still be several generations of at-risk, HPV-infected women who would not benefit from and would be unlikely to be targeted for HPV vaccination. Without robust screening, millions of women will die of cervical cancer before the impact of HPV vaccines on cervical cancer is observed [Reference Gage and Castle132]. Thus, secondary prevention by cervical cancer screening will be needed for the foreseeable future. Moreover, 2vHPV and 4vHPV provide only partial protection against cervical cancer.
However, cervical cancer screening in the HPV vaccination era will need to change in the absence of the two most carcinogenic HPV genotypes. As a consequence, positive screening tests will be less predictive of CIN3 and cervical cancer (CIN3+) because the point prevalence of CIN3+ will be reduced by ⩾50% CIN3+ while the test positivity will be reduced by ⩽30% [Reference Wright133–Reference Khan135]. Moreover, HPV16/18-related cervical cancer occurs at an earlier age than those related to other HPV genotypes [Reference de136] so the risk of cancer is lowered too. Less predictive screening means a poorer benefits-to-harms ratio.
Screening can be changed in several ways to help restore benefits-to-harms ratio in 2vHPV- or 4vHPV-vaccinated populations. First, screening can be started at an older age than is currently implemented, since population risk of cervical cancer will be lower in HPV-vaccinated (HPV16- and HPV18-negative) populations and cervical cancer caused by other HPV genotypes happens in women at median age of ~5 years older than cervical cancer due to HPV16 and HPV18. For example, many screening programmes screen unvaccinated women aged <25 years, despite the lack of evidence of benefit in this age group [Reference Sasieni, Castanon and Cuzick137], and vaccinated women in this age group would be even less likely to benefit from screening given their lower cancer risk. Second, using the principle of equal management for equal risk [Reference Katki138], longer intervals between screens and/or follow-up in management could be considered. This would allow more benign infections and related abnormalities to clear without detection and intervention while shifting the focus to persisting HPV infections that carry a significant risk of progression [Reference Castle14, Reference Kjaer15]. Finally, new, more specific biomarkers could be used to triage screen-positive women to help differentiate between benign hrHPV infections or related cytological abnormalities and clinically important hrHPV infections that have or will cause CIN3+. The most promising of these biomarkers include p16/Ki-67 immunocytochemistry [Reference Ikenberg139], E6 oncoprotein detection [Reference Qiao140], and HPV viral genome methylation [Reference Mirabello141, Reference Wentzensen142].
In mid-adult women, vaccination might be used to reduce the need for screening. Using a Vaccinate and Screen strategy [Reference Franceschi143], older women would be vaccinated and then screened 1 year after vaccination for the presence of hrHPV in the cervix. Any woman testing positive for hrHPV at follow-up will very likely have persistent hrHPV and be at high risk of having or developing CIN2+ [Reference Castle14, Reference Kjaer15], and could therefore be treated aggressively. Using a Screen and Vaccinate strategy [Reference Franceschi143], women would first be screened for hrHPV, with those testing hrHPV positive undergoing follow-up management or treatment. Logistically, all women would at least get the first dose before hrHPV status is known although it would be less beneficial to hrHPV-positive women directly although, as discussed above, it might reduce transmission to others. Either the whole population or those who tested hrHPV negative would then get 1–2 additional doses.
In both scenarios, vaccinated, low-risk women are protected against the acquisition of new infections by the highest risk HPV genotypes and might never need to be screened again, or at a much lower frequency than if they had not been vaccinated. Women who tested positive would be screened until safety against cervical cancer was assured, e.g. testing hrHPV negative once or twice. Both approaches could be promising strategies, especially in lower-resource settings that may not be financially able to sustain multiple rounds of screening but the relative cancer risk reduction, cost, and cost-effectiveness of vaccination vs. a second-round of screening is needs to be evaluated. In high-resource settings, mid-adult women might choose between continued screening every 3–5 years or HPV vaccination followed by 1–2 screens until the age of 65 or 70 years. These approaches might be best realized with the 9vHPV if its protection is durable and its costs are comparable to 2vHPV and 4vHPV.
In HPV-naive populations vaccinated with the 9vHPV vaccine, the question will be whether to screen at all. Most, or if there is cross-protection vs. the hrHPV not targeted by 9vHPV, virtually all CIN3+ will be prevented. The few remaining CIN3 due to borderline hrHPV or low-risk HPV genotypes may rarely if ever become invasive cancer. These HPV genotypes can still cause significant numbers of minor cytological [Reference Kovacic144, Reference Wentzensen145] and histological [Reference Joste146] abnormalities that have little clinical importance but would be picked up by screening. Thus, the harms to the patients and costs would be disproportionately high compared to the benefits to the patients. Speculatively, a single screening of mid-adult women around the age of 35 or 40 years may be valuable if it leads to detection of early-stage cervical cancer caused by hrHPV types not covered by 9vHPV of or those caused by borderline hrHPV, and perhaps other female reproductive tract cancers [Reference Kinde147] if the lead-time detection of the latter provides significant health benefit (e.g. reduced mortality).
GLOBAL HEALTH
The real challenge and opportunity for HPV vaccination is global access. Worldwide, there are currently about 290 million females aged 9–13 years [148] and only a small proportion of them have or will receive HPV vaccination. Over the next 5 years, at least an equal number of females will ‘age into’ that target group, i.e. more than 500 million early adolescent women would benefit from vaccination in the next 5 years.
There are many societal and behaviour barriers to adoption of HPV vaccination: cost, healthcare infrastructure, patient, parent, and provider education, and cultural acceptance, to name a few. The one that looms largest is cost. A recent global analysis of HPV vaccination in 179 countries [Reference Jit149] showed that HPV vaccination of young girls is very cost-effective [below gross domestic product (GDP) per head] in 156 (87·2%) countries and cost-effective in 17 (9·5%) countries (less than three times GDP per head). HPV vaccination was not cost-effective in six (3·4%) countries (mostly in the Eastern Mediterranean region) with low reported incidence of cervical cancer compared to other countries with similar income. However, changing societal and cultural norms for sexual behaviours in the extended Middle East and Northern Africa region may increase the risk of HPV-related cancers [Reference El-Kak150, Reference Vaccarella, Bruni and Seoud151], and HPV vaccination in those six low-risk countries eventually may be cost-effective as well. Using two doses (vs. three) and/or using 9vHPV, if its cost is comparable to the other vaccines, may make HPV vaccination an even better value, with the noted caveats discussed above. These data support universal HPV vaccination of 9- to 13-year-old females.
Yet, HPV vaccination remains a significant healthcare investment for low- and middle-income countries (LMICs), especially those that are not eligible for Global Alliance for Vaccine and Immunization (GAVI) support. Countries that are below $1580 gross national income per capita Footnote † (‘GAVI eligible’) and demonstrate competency to deliver the HPV vaccine (e.g. 2-year demonstration project or implementation of a national programme and achieved at least 70% coverage with the third dose of diphtheria-tetanus-pertussis vaccine) can receive GAVI support for HPV vaccination for as long as the country remains GAVI eligible. GAVI pays US$4.5 per dose and provides the vaccine to the GAVI-eligible country minus a small contribution of ~US$0.25 per dose for which the country is responsible. GAVI has stated its intent to switch to a two-dose vaccination schedule in the near future. However, for the lowest-income countries, the main financial burden will not be the actual cost of the vaccine, regardless of GAVI support, but the cost of infrastructure, delivery, and any monitoring and evaluation activities.
In 2011, following a national education and awareness campaign, Rwanda was the first LMIC to implement a national HPV vaccination programme [Reference Binagwaho152]. Rwanda achieved 93% three-dose coverage with 4vHPV vaccination of 6th-grade females in its first year. High coverage was achieved through school-based vaccination and community outreach to those females who were absent from or not enrolled in school. The roll-out of HPV vaccination nationally through the Merck Donation Programme (2011–2013) served as the ‘demonstration’ necessary to become a GAVI-supported programme in 2013.
For the other, GAVI-ineligible countries, cost becomes a much more significant barrier to implementation, and volume purchasing strategies and financing programmes for these countries are desperately needed. The Pan-American Health Organization (PAHO), through their Revolving Fund, can provide HPV vaccine to Latin American Countries at US$12 per dose [153], but even with using a two-dose schedule this still represents ~1% of the GDP per capita for people living in the lowest-income Latin American countries [154] – countries like Bolivia and Guyana with cervical cancer incidence rates approaching rates observed in Sub-Saharan Africa [Reference Ferlay155] would benefit greatly from HPV vaccination. Many LMICs also have the lowest percentage of GDP spent on healthcare (all <10% and some <3% [156]), so that HPV vaccination would represent a significant proportion of the total healthcare expenditures.
HPV vaccination also represents a long-term healthcare investment, with the main return on investment, the reduction of HPV-related cancer, not coming for 20 years after vaccination. In many of LMICs that would benefit the most from HPV vaccination, there are no monitoring and evaluation programmes and no population-based cancer registries to provide data on the impact – and value – of HPV vaccination. In the context of governments that change every few years, HPV vaccination may be a difficult sell, especially in the face of other, more immediate healthcare needs.
Most important, the sole reliance on Pap-based screening for cervical cancer, as advocated by some [Reference Suba and Raab157], will never address the global need for cervical cancer prevention. Without adopting alternative strategies to Pap such as prophylactic HPV vaccination for primary prevention and sensitive molecular hrHPV testing for screening and secondary prevention, between 25 and 50 million women will get cervical cancer in the next 50 years, ⩾60% of whom will die from it [Reference Gage and Castle132]. There are now safe and effective HPV vaccines and we have global responsibility to enable access to these vaccines for all young adolescent women and perhaps some or all young adolescent males, depending on costs and available resources. In the future, with universal adolescent female HPV vaccination, women need not die of this highly preventable cancer, nor men and women of the other HPV-related cancers.
ACKNOWLEDGEMENTS
None.
DECLARATION OF INTEREST
Dr Castle has received commercial HPV tests for research at a reduced or no cost from Roche, Qiagen, Norchip, Arbor Vita Corporation, BD, and mtm. He has been compensated financially as a member of a Merck Data and Safety Monitoring Board for HPV vaccines. He has been paid as consultant for BD, Gen-Probe/Hologic, Roche, Cepheid, ClearPath, Guided Therapeutics, Teva Pharmaceutics, Genticel, Inovio, and GE Healthcare, and has received honoraria as a speaker for Roche and Cepheid.
Dr Maza has no commercial relationship with any entity that produces or distributes HPV-related products.