Artichoke (Cynara scolymus L.) is an ancient herbaceous perennial plant, originating from the Mediterranean area, which today is cultivated all over the world. The leaves of artichoke have been widely used in herbal medicine as a choleretic and diuretic since ancient times (Bruneton, Reference Bruneton1995).
Several studies have demonstrated the efficiency and safety of artichoke extracts in the treatment of hepato-biliary disfunction and digestive complaints in animals (Adzet et al. Reference Adzet, Camarasa and Laguna1987; Speroni et al. Reference Speroni, Cervellati, Govoni, Guizzardi, Renzulli and Guerra2003) and in human subjects (Kraft, Reference Kraft1997; Kirchhoff et al. Reference Kirchhoff, Beckers, Kirchhoff, Trinczek-Gartner, Petrowitz and Reimann1994). Several clinical investigations showed the ability of artichoke extract to prevent the oxidative modification of blood lipoproteins and to reduce blood cholesterol levels (Kirchhoff et al. Reference Kirchhoff, Beckers, Kirchhoff, Trinczek-Gartner, Petrowitz and Reimann1994; Gebhardt, Reference Gebhardt1998, Reference Gebhardt2002; Zapolska-Downar et al. Reference Zapolska-Downar, Zapolski-Downar, Naruszewicz, Siennicka, Krasnodębska and Kolodziej2002; Shimoda et al. Reference Shimoda, Ninomiya, Nishida, Yoshino, Morikawa, Matsuda and Yoshikawa2003). Moreover leaf extracts have been reported to show antioxidative and protective properties against hydroperoxide-induced oxidative stress in cultured rat hepatocytes (Gebhardt, Reference Gebhardt1997; Miccadei et al. Reference Miccadei, Bugianesi, Di Venere, Cardinali, Linsalata, Foddai and Maiani2004). Artichoke beneficial effects seem due to the biochemical constituents, mainly polyphenols (chlorogenic acid (CGA), mono- and di-caffeoylquinic acids and flavonoids such as luteolin and apigenin glycosides), sugars and inulin. The health-promoting prebiotic effects of artichoke inulin were related to the stimulation of bifidobacterial growth in the intestine (Roberfroid et al. Reference Roberfroid, Van Loo and Gibson1998; Lopez-Molina et al. Reference Lopez-Molina, Navarro-Martinez, Melgarejo, Hiner, Chazarra and Rodríguez-Lopez2005). The characterization and quantification of bioactive molecules in artichoke have been studied extensively (Wang et al. Reference Wang, Simon, Aviles, He, Zheng and Tadmor2003; Schütz et al. Reference Schütz, Kammerer, Carle and Schieber2004; Zhu et al. Reference Zhu and Zhang2004) while little is known on the bioavailability and bioactivity of these substance from natural or supplemental sources. Polyphenols are abundant micronutrients in our diet and evidence for their role in the prevention of degenerative disease is emerging. Bioavailability greatly differs from one polyphenol to another, so that the most abundant polyphenols in our diet are not necessarily those leading to the highest concentration of active metabolites on target tissues (Manach et al. Reference Manach, Scalbert, Morand, Rémésy and Jiménez2004, Reference Manach, Scalbert, Morand, Rémésy and Jiménez2005).
The aim of this study was to evaluate the absorption and metabolism of bioactive molecules after oral consumption of cooked edible portion of artichoke heads in human subjects.
Materials and methods
Chemicals and reagents
All solvent reagents were HPLC or Optima grade; common reagents were purchased from Sigma (St Louis, MO, USA), Carlo Erba (Milan, Italy) and BDH Laboratory Supplies. (Poole, Dorset, UK) CGA, caffeic acid (CA), ferulic acid (FA), apigenin (Lyon Nord, France) and luteolin were purchased from Sigma, dihydrocaffeic acid (DHCA) from Extrasynthese (Lyon Nord, France) and dihydroferulic acid (DHFA) from AVOCADO Research Chemicals Ltd (Morecambe, Lancashire, UK).
Subjects
Five healthy non smoking subjects (two males and three females), aged 25–32 years, body weight 61·0 (sd 9.5) kg and BMI 21·5 (sd 1·5) kg/m2, were recruited on the basis of their normal blood values and clinical anamnesis from the National Institute for Research on Food and Nutrition staff (INRAN, Rome).
Each subject signed an informed consent approved by the Ethical Committee of INRAN. Several studies have shown significant effects of treatment with a small number of subjects (Hollman et al. Reference Hollman, van der Gaag, Mengelers, van Trijp, de Vries and Katan1996; McAnlis et al. Reference McAnlis, McEneny, Pearce and Young1999; Cremin et al. Reference Cremin, Kasim-Karakas and Waterhouse2001; Rechner et al. Reference Rechner, Spencer, Kuhnle, Hahn and Rice-Evans2001a; Olthof et al. Reference Olthof, Hollman and Katan2001; Bugianesi et al. Reference Bugianesi, Salucci, Leonardi, Ferracane, Catasta, Azzini and Maiani2004).
Subjects did not use any drug or vitamin supplementation. During the three days before the experimental day, subjects were asked to follow a controlled diet limiting fruit and vegetables consumption to not more than 100 g/day, and avoiding artichokes, carrots, tomatoes, apples, pears, peaches, cherries, strawberries, blueberries, tea, coffee, wine, beer, chocolate and all their derived products. Food consumption during the three days of wash out was surveyed by a dietitian to check the diet balance by a record and recall method.
Study design
After fasting overnight, baseline blood samples were collected from each subject. After blood collection, all subjects consumed 61·7 (sd 4·4) g edible artichoke (cultivar Violetto di Provenza), steam cooked with 5·5 (sd 0·4) g extra virgin olive oil and venous blood sample collection was repeated at 0·5, 1, 2, 4, 6 and 8 h from the experimental test meal consumption.
Total energy and macronutrient content were calculated by using Food Composition Tables (National Institute for Research on Food and Nutrition, 2000). CGA, mono- and di-caffeoylquinic acids, cynarin (1,3-dicaffeoylquinic acid), luteolin-glycoside and apigenin-glycoside contents of the experimental meal are reported in Table 1.
Table 1 Polyphenol contents of the experimental meal. (Mean values and standard deviations for replicate analyses on cooked artichoke)

The edible portion of artichoke heads was chosen on the basis of analysed polyphenols content of cultivar selected for the study. Artichoke heads, consumed by all participants, were collected raw and cooked in order to evaluate the polyphenol contents. Table 2 shows replicate analyses of artichoke heads both raw, and cooked as mg/100 g edible portion.
Table 2 Selected polyphenols analysis on raw and cooked Cynara scolymus L (cultivar Violetto di Provenza) heads. (Mean values and standard deviations)
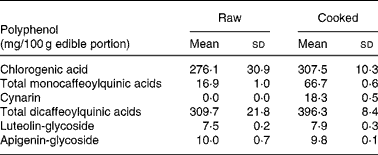
Artichoke analysis
About 25 g raw and cooked heads of artichoke were homogenized in a 250 ml flask by adding 150 ml MeOH and were extracted by stirring, heating and refluxing for 90 min with regular swirling.
The solutions obtained were filtered and the residues were extracted twice after adding 100 ml MeOH by stirring, heating and refluxing for 30 min with regular swirling. Pooled extracts were evaporated by rotavapor and reconstituted by adding 100 ml H2O–MeOH (1:1, v/v).
The determination of polyphenols in artichoke heads was carried out in agreement with Di Venere et al. (Reference Di Venere, Linsalata, Calabrese, Pieralice, Bianco and Lattanzio2005) and the quantification was performed by HPLC using a liquid chromatograph equipped with binary gradient pump and spectrophotometric photodiode array detector (Agilent Technologies S.P.A., Milan, Italy). The Hewlett Packard Chem Station (Rev. A. 06.03) software was used for spectra and data processing. An analytical Phenomenex (Torrance, CA, USA) Ultracarb 5 ODS (30) column (4·6 × 250 mm) was used for peak separation and the elution profile was reported by Lattanzio & Van Sumere (Reference Lattanzio and Van Sumere1987). The method recovery performance was assayed by addition of suitable amounts of standards prior to the extraction of the solid samples. Recovery rates of the method were found to be >85 % for caffeoylquinic acids as well as luteolin and apigenin glycosides.
The method precision was assayed by three series of extractions, each performed in triplicate using one different artichoke head. The percentage relative standard deviation for the normalized peak areas of all evaluated compounds was found to be < 5 % (data not shown).
Table 3 Pharmacokinetic parameters of total chlorogenic acid (CGA), caffeic acid (CA), ferulic acid (FA), dihydrocaffeic acid (DHCA) and dihydroferulic acid (DHFA) absorption in human plasma after consumption of cooked Cynara scolymus L. (cultivar Violetto di Provenza) heads (Mean values and standard deviations)

Cmax, maximum concentration; AUC0-last, area under the curve from 0 to the last sampling time; t Max, time to reach Cmax.
Plasma samples
Blood samples were drawn into vacutainers containing EDTA as anticoagulant. Blood samples were centrifuged at 3600 g for 10 min at 4°C, plasma was separated and stored at − 80°C until analysis. In all plasma samples, the following target compounds: CGA, CA, DHCA, FA, DHFA, apigenin and luteolin were analyzed in duplicate with and without enzymatic hydrolysis to detect free and conjugated form. Single phenol levels were evaluated by HPLC with electrochemical detector before and after enzymatic hydrolysis as described by Bugianesi et al. (Reference Bugianesi, Salucci, Leonardi, Ferracane, Catasta, Azzini and Maiani2004) with slight modification. Briefly for free forms, 100 μl ascorbic acid solution (1 %) was added to 0·5 ml plasma and after vortexing and protein precipitation by 500 μl 3 mol/l HCl–MeOH (1:1, v/v), free phenols were extracted by adding 2 ml ethyl acetate, followed by stirring and sonication (2–3 min) before centrifugation at 1700 g for 5 min. The extraction procedure was repeated twice and the two organic layers were combined and evaporated. The residue was dissolved into 200 μl mobile phase (phosphate buffer pH 2·8 and methanol (1:1, v/v)). Total phenols were evaluated after adding 100 μl ascorbic acid solution (1 %), 100 μl 0·2 m acetic acid and 50 μl β-glucoronidase Type HP2 from Helix Pomatia (Sigma-Aldrich Srl, Milan, Italy). After incubating at 37°C for 1 h proteins were precipitated by adding 700 μl 3 mol/l HCl–MeOH (1:1, v/v), and total plasma phenols were extracted as previously described for free forms. Blank reagents were performed for free and total phenols forms evaluation. Quantitative analyses were performed on 50 μl extract using an ESA–HPLC system (ESA, Chelmsford, MA, USA). The HPLC system used consisted of an ESA MODEL 540 refrigerated autoinjector (4°C), an ESA MODEL 580 solvent delivery module with two pumps, an ESA 5600 eight-channels coulometric electrode array detector and the ESA coularray operating software which controlled all the equipment and carried out data processing. A Supelcosil LC-18 column (25 cm × 4·6 mm, 5 μm) with a Perisorb Supelguard LC-18 (Supelco, Milan, Italy) was used. Chromatography was performed at 30°C, at a flow rate of 0·8 ml/min using the following solvent system: solvent A, 0·02 mol NaH2 PO4-H2O adjusted to pH 2·8 with 85 % orthophosphoric acid; solvent B, methanol. The linear gradient used consisted of 10 % solvent B, increasing to 30 % over 7 min and to 33 % over 28·5 min, increasing to 45 % over 19·5 min, held for 8·5 min and reaching the final condition of 100 % 24 min later, after that returning to 10 % solvent B over 3 min and maintaining this condition for 4 min. The flow rate of the eluent was constant at 0·8 ml/min and the setting potentials were 60, 120, 200, 340, 480, 620, 760 and 900 mV. Sample peaks were analyzed by matching target peaks with standard peaks on the basis of their retention time and on accuracy ratio between adjacent channels. The correlation coefficient for all calibration curves was >0·99. Spiked samples were processed and analyzed exactly as described earlier. Mean recovery of polyphenol standards added to plasma (n 3) was >80–118 % for CGA, DHCA, CA, DHFA and FA. The method showed a good reproducibility with coefficient of variation within days < 5 % and between days < 9 %. Limits of detection were 2·5 ng/ml for CGA, DHCA, DHFA and FA and 2·7 ng/ml for CA.
Statistics
Data are given as the mean and standard deviation. Statistical analysis was performed using the non-parametric Friedman ANOVA test and the Wilcoxon matched pairs test. Differences were considered significant at P < 0·05. The computer program used was STATISTICA for Windows (release 4·5; StatSoft Inc., Vigonza PD, Italy).
Results
The composition of both raw and cooked artichoke heads is reported in Table 2. In order to evaluate the effect of domestic cooking on the content of polyphenols, the artichoke heads were analyzed before and after cooking, observing slight increase in CGA content. In addition, after cooking, the increasing of mono- and di-caffeoylquinic acids and the appearance of cynarin were observed. There were no differences in luteolin-glycoside and apigenin-glycoside content. The evaluation of polyphenol content in raw food has been widely studied and optimized on different food matrixes (Hertog et al. Reference Hertog, Hollman and Venema1992). Several studies have shown the influence of heating treatments on antioxidant content and their stability (Brenes et al. Reference Brenes, García, Dobarganes, Velasco and Romero2002; Lombard et al. Reference Lombard, Peffley, Geoffriau, Thompson and Herring2005; Innocenti et al. Reference Innocenti, Gallori, Giaccherini, Ieri, Vincieri and Mulinacci2005; Rubinskiene et al. Reference Rubinskiene, Viskelis, Jasutiene, Viskeliene and Bobinas2005; Budic-Leto et al. Reference Budic-Leto, Lovric, Kljusuric, Pezo and Vrhovsek2006).
Fig. 1 shows the plasma concentrations of target compounds after consumption of cooked artichoke heads. Maximum concentration of 6·4 (sd1·8) ng/ml for CGA was reached after 1 h and declined until its disappearance within 2 h (P < 0·05).
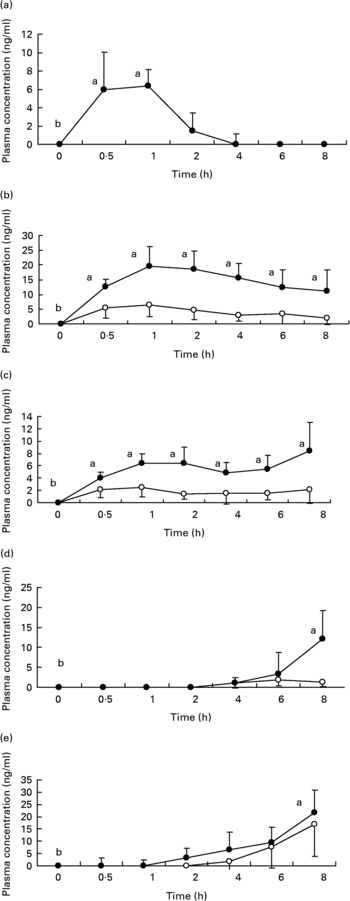
Fig. 1 Plasma concentrations of (a) chlorogenic acid; (b) caffeic acid; (c) ferulic acid; (d) dihydrocaffeic acid and (e) dihydroferulic acid after consumption of cooked Cyanara scolymus L. (cultivar Violetta di Provenza) heads. Values are means for five subjects with standard deviations shown by vertical bars. ○, plasma concentration of unconjugated polyphenols; ●, plasma concentration of conjugated plus uncongugated polyphenols. a,bWilcoxon test for comparison a v. b, P < 0·05.
Peak plasma concentrations of 19·5 (sd 6·9) ng/ml for total CA were reached within 1 h while FA plasma concentrations showed a biphasic profile with 6·4 (sd 1·5) ng/ml and 8·4 (sd 4·6) ng/ml within 1 h and after 8 h respectively. We observed a significant (P < 0·05) increase with respect to baseline for both CA and FA after administration of cooked head of artichoke during the entire experimental time.
Increase of DHCA and DHFA total levels was observed within 8 h, to 12·1 (sd 7·2) ng/ml (P < 0·05) and 21·8 (sd 9·2) ng/ml (P < 0·05) respectively.
Representative chromatograms in Fig. 2 show the absorption profiles in one subject of target artichoke compounds at baseline and during the entire experimental time compared to specific standards. The unnamed peaks have to be considered unknown.
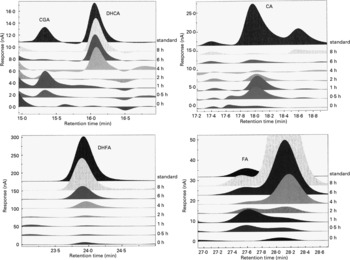
Fig. 2 Representative chromatograms of the absorption profiles in one subject of target artichoke compounds (CGA, chlorogenic acid; DHCA, dihydrocaffeic acid; CA, caffeic acid; DHFA, dihydroferulic acid; FA, ferulic acid) at baseline and during the entire experimental time compared to specific standards. The unnamed peaks have to be considered unknown.
No circulating plasma levels of luteolin and apigenin were present.
Discussion
The current study shows that some artichoke compounds are able to cross the gastric barrier; our findings demonstrate that artichoke target compounds, after crossing the intestinal barrier, reach biologically effective concentrations in the bloodstream. It is well known that most classes of polyphenols are sufficiently absorbed to have the potential to exert biological effects. For example, quercetin after consumption of a meal containing onions, catechins after tea consumption, isoflavones after soya consumption and caffeic acid after drinking red wine, reach micromolar concentrations in blood (Abu Amsha Caccetta et al. Reference Abu Amsha Caccetta, Croft, Puddey, Proudfoot and Beilin1996; Hollman et al. Reference Hollman, van Trijp, Buysman, van der Gaag, Mengelers, de Vries and Katan1997; Maiani et al. Reference Maiani, Serafini, Salucci, Azzini and Ferro-Luzzi1997; Scalbert & Williamson, Reference Scalbert and Williamson2000; Manach et al. 2002). Bioavailability depends on several endogenous and exogenous factors; the endogenous seem to be related to food matrix, size, chemical structure and ingested amount, the exogenous to digestive enzyme activities and bile excretion, and biotransformations related to liver, kidney, gastrointestinal epithelium and gut microbial flora.
The mean time to reach maximum concentration of 6·4 (sd1·7) ng/ml for CGA is surprising. Some authors have observed a fast absorption of CGA after oral administration of pure CGA and honeysuckle flower extract in rat (Lafay et al. Reference Lafay, Gil-Izquierdo, Manach, Morand, Besson and Scalbert2006) and rabbit (Yang et al. Reference Yang, Yuan, Li, Chen and Li2004) respectively. Unmodified CGA also appears in human urine (Cremin et al. Reference Cremin, Kasim-Karakas and Waterhouse2001; Olthof et al. Reference Olthof, Hollman and Katan2001; Ito et al. Reference Ito, Gonthier, Manach, Morand, Mennen, Rémésy and Scalbert2005). On the contrary, other authors have failed to detect intact CGA in plasma after its administration as a pure compound in rats (Azuma et al. Reference Azuma, Ippoushi, Nakayama, Ito, Higashio and Terao2000) or after ingestion of coffee in human subjects (Nardini et al. Reference Nardini, Cirillo, Natella and Scaccini2002). The rapid plasma detection of CGA could be due to absorption of intact CGA through the stomach or the small intestine barrier. Lafay et al. (Reference Lafay, Gil-Izquierdo, Manach, Morand, Besson and Scalbert2006) have identified unchanged CGA in both the gastric vein and aorta showing that the stomach could be involved in CGA absorption in rats. Olthof et al. (Reference Olthof, Hollman and Katan2001) have indicated that one third of ingested CGA was absorbed in the small intestine in human subjects. Nevertheless, our low plasma CGA levels suggest that the largest portion of CGA and caffeoylquinic acids have undergone biotransformations.
CGA and caffeoylquinic acid hydrolysis, and their metabolites release, could begin in the small intestine. In addition, Andreasen et al. (Reference Andreasen, Kroon, Williamson and Garcia-Conesa2001) have shown that esterases with activity similar to esters of the major dietary hydroxycinnamates are distributed throughout the intestinal tract of mammals.
The observed CA and FA plasma levels could originate from hydrolysis of both CGA and caffeoylquinic acids, that represent the predominant polyphenol forms in the test meal.
Free plasma levels were low with respect to total forms of CA and FA (Wittemer et al. Reference Wittemer, Ploch, Windeck, Müller, Drewelow, Derendorf and Veit2005); CA and FA conjugates reached the maximum concentration within 1·5 h. This result seems to suggest that the hydrolysis of active compounds of artichoke heads should have partially occurred in the small intestine (Lafay et al. Reference Lafay, Gil-Izquierdo, Manach, Morand, Besson and Scalbert2006; Azuma et al. Reference Azuma, Ippoushi, Nakayama, Ito, Higashio and Terao2000; Nardini et al. Reference Nardini, Cirillo, Natella and Scaccini2002).
On reaching the large intestine, unchanged CGA is hydrolyzed by colon enzymes into various aromatic acid metabolites before absorption (m-coumaric acid, derivatives of phenylpropionic and benzoic acids; Booth et al. Reference Booth, Emerson, Jones and Deeds1957; Rechner et al. Reference Rechner, Spencer, Kuhnle, Hahn and Rice-Evans2001a). Several metabolites have been identified after ingestion of CGA or CA, namely FA, isoFA, DHFA and vanillic acid in human subjects (Rechner et al. Reference Rechner, Pannala and Rice-Evans2001b).
The colonic microflora has been designated as the major metabolic site for the reactions of the cleavage of ester or glycoside bonds, releasing free hydroxycinnamic acids (Plumb et al. Reference Plumb, Garcia-Conesa, Kroon, Rodhes, Ridley and Williamson1999; Couteau et al. Reference Couteau, McCartney, Gibson, Williamson and Faulds2001; Rechner et al. Reference Rechner, Spencer, Kuhnle, Hahn and Rice-Evans2001a).
CA, released by gut microflora, was absorbed and transported to the liver for o-methylation to FA (Masri et al. Reference Masri, Robbins, Emerson and De Eds1964; Scheline, Reference Scheline1991; Gumbinger et al. Reference Gumbinger, Vahlensieck and Winterhoff1993; Moridani et al. Reference Moridani, Scobie, Jamshidzadeh, Salehi and O'Brien2001). Nevertheless the gut microflora could be able to catalyze the o-methylation of CA to yield FA and isoFA (Chesson et al. Reference Chesson, Provan, Russell, Scobbie, Richardson and Stewart1999).
From our findings, the mean time to reach maximum concentration for DHCA and DHFA was 6–8 h (Wittemer et al. Reference Wittemer, Ploch, Windeck, Müller, Drewelow, Derendorf and Veit2005). The decrease of CA levels within 6 h and the simultaneous increase of DHCA and DHFA in the bloodstream seem to suggest that CA, released after hydrolysing CGA and caffeoylquinic acids by microflora, was metabolized into DHCA before absorption (Peppercorn & Goldman, Reference Peppercorn and Goldman1971). This result could indicate the gut as probable and predominant location of hydroxycinnamate esters metabolism (Andreasen et al. Reference Andreasen, Kroon, Williamson and Garcia-Conesa2001, Couteau et al. Reference Couteau, McCartney, Gibson, Williamson and Faulds2001).
Absorbed DHCA could be methylated into DHFA and then dehydrogenated into FA in the liver (Moridani et al. Reference Moridani, Scobie, Jamshidzadeh, Salehi and O'Brien2001). Nevertheless our results indicate that the same reaction mechanisms could take place in the large intestine, particularly dehydrogenation by the colon flora and methylation inside the colon wall. This may contribute to explaining the biphasic concentration profile observed in our samples for total FA.
Moridani et al. (Reference Moridani, Scobie and O'Brien2002) have reported that CA and DHCA biotransforming reactions, such as o-methylation, GSH conjugation, hydrogenation and dehydrogenation, take places in the liver catalyzed by P450 cytocrome. On the other hand, some researchers suggest that CA and DHCA methylation into FA and DHFA respectively may occur in the gut (Booth et al. Reference Booth, Emerson, Jones and Deeds1957; Chesson et al. Reference Chesson, Provan, Russell, Scobbie, Richardson and Stewart1999; Ranganathan & Ramasarma, Reference Ranganathan and Ramasarma1974). In both rats and human subjects, dietary plant phenolics can undergo metabolism to form reactive intermediates by catechol-o-methyltransferases present in the liver, also in the kidneys and gastrointestinal tracts both stomach and intestine (Nissinen et al. Reference Nissinen, Linden, Schultz, Kaakkola, Mannisto and Pohto1988; Schultz & Nissinen, Reference Schultz and Nissinen1989; Mannisto et al. Reference Mannisto, Tuomainen and Tuominen1992).
The lack of circulating levels of luteolin and apigenin could be due to their low concentrations in the test meal administered. Several factors may explain the variability of the polyphenols bioavailability, such as the food matrix or background diet. In addition inter-individual variations are also important, because some people might have different levels of metabolizing enzymes or transporters, enabling more efficient absorption of bioactive compounds.
In conclusion, our study shows for the first time the absorption pathways of hydroxycinnamic acids after consumption of edible cooked artichoke in human subjects. These results should be supported by other investigations to confirm the biological activity of cooked artichoke in human subjects and to better understand the mechanism of food phenolic metabolism. With the current conflict existing in the understanding of the metabolism of hydroxycinnamic acids further research is required.
Acknowledgements
We thank Dr Sergio Corelli for his medical assistance during the study, Ms Lara Palomba for her assistance during the preparation of the paper, Dr Federica Intorre for statistical analyses and Dr Angela Cardinali for her contribution to the study. Finally, thanks are also given to all study participants. This study was supported by MiPAF.