Introduction
Chickpea (Cicer arietinum L.) is an important winter crop in eastern Australia, especially in dryland regions (Chauhan et al. Reference Chauhan, Allard, Williams, Williams, Mundree, Chenu and Rachaputi2017). After lupin (Lupinus spp.), chickpea is Australia’s second-most important pulse crop, occupying an area of 0.37 million ha with an annual production of 360 million kg and a productivity of 970 kg ha−1 (ABARES 2022). There is an increased interest among growers for chickpea cultivation in Australia due to its high export potential and low water requirement. Besides this, chickpea crops restore soil fertility and help to break the life cycle of cereal root diseases, such as crown rot (Berger et al. Reference Berger, Turner, Siddique, Knights, Brinsmead, Mock, Edmondson and Khan2004; GRDC Reference Chauhan, Allard, Williams, Williams, Mundree, Chenu and Rachaputi2017).
Chickpea is a slow-growing crop that can be adversely affected by weed competition at an early growth stage (Blackshaw et al. Reference Blackshaw, Lemerle, Mailer and Young2002; Campbell Reference Campbell2016; Mohammadi et al. Reference Mohammadi, Javanshir, Khooie and Mohammadi2005). In eastern Australia, the chickpea crop is grown using wide row spacings (50- to 100-cm wide), and Brassicaceae weeds, such as African mustard (Brassica tournefortii Gouan), turnipweed [Rapistrum rugosum (L.) All.], and African turnipweed (Sisymbrium thellungii O.E. Schulz), commonly infest chickpea crops (Beech and Leach Reference Beech and Leach1989; GRDC 2017). Total revenue losses due to the infestation of B. tournefortii in Australia have been estimated to be about AU$10.6 million annually (Llewellyn et al. Reference Llewellyn, Ronning, Clarke, Mayfield, Walker and Ouzman2016). There is no information about the revenue losses due to infestations of R. rugosum and S. thellungii in the cropping regions of Australia.
Sisymbrium thellungii is an emerging weed in winter crops of eastern Australia, where its infestation is more common in northern New South Wales and southern and central Queensland (Scott and Panetta Reference Scott and Panetta1993). The seed production potential of S. thellungii is very high. In chickpea, one S. thellungii plant could produce >10,000 seeds plant–1 (Widderick et al. Reference Widderick, Keenan and Walsh2014). In another study, it was found that early cohorts (May) of S. thellungii produced >90,000 seeds plant−1 (unpublished data).
Plants of S. thellungii can produce a large number of seeds (4,000 seeds plant−1) under severe water-stress conditions, indicating its adaptation to drought (Mahajan et al. Reference Mahajan, George-Jaeggli, Walsh and Chauhan2018). In a field study, the seed persistence of this weed was less than 2 yr, and emergence was observed from May to June (unpublished data). A previous study on the seed germination ecology of S. thellungii revealed that its highest germination occurred at 20/10 C (day/night temperature), suggesting its high adaptability to winter environmental conditions (Mahajan et al. Reference Mahajan, George-Jaeggli, Walsh and Chauhan2018). This information suggests that the biological attributes of this weed are favorable for its increased infestation in winter crops, such as chickpea. In Australia, resistance to Group 2 herbicides has been reported in S. thellungii (Wills et al. Reference Wills, Walker and Adkins1996). Because herbicide options for broadleaf weed control in chickpea are limited, S. thellungii infestation in chickpea is a concern for its potential negative impact on crop yield.
Brassica tournefortii is a winter weed and is widely distributed throughout Australia (Alemseged et al. Reference Alemseged, Jones and Medd2001). This weed is well adapted to low-rainfall regions and is therefore a threat to chickpea production in dry regions. In a pot study, this weed produced up to 3,000 seeds plant−1 when grown at the 25% water-holding capacity of the soil (Mahajan et al. Reference Mahajan, Singh and Chauhan2020). Without crop competition in field conditions, B. tournefortii produced 10,000 seeds plant−1; however, seed production was reduced by 30% and 80% when grown in competition with chickpea and wheat (Triticum aestivum L.), respectively (Mahajan et al. Reference Mahajan, Singh and Chauhan2020). This information suggests that the seed production potential of this weed is greater when it interferes with chickpea compared with wheat.
Brassica tournefortii has the ability to germinate at a wide range of temperatures (15/5 to 35/15 C, day/night), suggesting that this weed could emerge in no-till chickpea fields at crop emergence time and provide significant competition (Singh et al. Reference Singh, Mahajan, Singh and Chauhan2021). In a field study, it was observed that B. tournefortii emerged in multiple cohorts from March to October (autumn, winter, and spring), indicating its adaptation to varying environmental conditions (Mahajan et al. Reference Mahajan, Singh and Chauhan2020). Its biological attributes, such as high seed production, seed-shattering tendency, and potential to emerge under a wide range of environmental conditions, favor the emergence and establishment of this weed in eastern Australia (Mahajan et al. Reference Mahajan, Singh and Chauhan2020; Singh et al. Reference Singh, Mahajan, Singh and Chauhan2021). Furthermore, the long persistence (>2 yr) of B. tournefortii seeds in shallow soil layers could increase the infestation of this weed in no-till systems (Mahajan et al. Reference Mahajan, Singh and Chauhan2020).
In wheat, a low density of B. tournefortii (5 plant m−2) could reduce the yield by 10% (Chauhan et al. Reference Chauhan, Gill and Preston2006; Gill et al. Reference Gill, Borger and Chauhan2022). However, such information on chickpea yield is limited under Australian conditions. Furthermore, evolved resistance of this weed to Group 2 herbicides makes it a serious concern for its infestation in chickpea (Alemseged et al. Reference Alemseged, Jones and Medd2001).
Rapistrum rugosum has a wide distribution throughout Australia (Ohadi et al. Reference Ohadi, Mashhadi and Tavakol-Afshari2011; Osten et al. Reference Osten, Walker, Storrie, Widderick, Moylan, Robinson and Galea2007; Whish et al. Reference Whish, Sindel, Jessop and Felton2002). Recently, it has become more prevalent in northern cropping regions of Australia (Manalil and Chauhan Reference Manalil and Chauhan2019). Rapistrum rugosum has the potential to produce about 13,000 seeds plant−1 in fallow when it emerges at the end of autumn (April) (Mobli et al. Reference Mobli, Manalil, Khan, Jha and Chauhan2020). In another study, 47 plants m−2 of R. rugosum in competition with wheat produced 32,000 seeds m−2 (Manalil and Chauhan Reference Manalil and Chauhan2019).
The germination of R. rugosum was found to be >85% at temperatures ranging from 15/5 to 25/15 C (day/night temperature), suggesting the ability of this weed to germinate in winter environments (Manalil et al. Reference Manalil, Ali and Chauhan2018). With optimal germination temperatures coinciding with chickpea cultivation, long seed persistence (>3.5 yr) in the soil and the ability to emerge from near the soil surface may increase R. rugosum infestation in chickpea crops in Australia (Gill et al. Reference Gill, Borger and Chauhan2022; Manalil and Chauhan Reference Manalil and Chauhan2021).
In Australia, R. rugosum has evolved resistance to Group 2 herbicides (Adkins et al. Reference Adkins, Wills, Boersma, Walker, Robinson, McLeod and Einam1997). The plants of R. rugosum are tall and could shade a chickpea crop at the flowering stage and reduce its yield. It has been estimated that 18 to 24 plants m−2 of R. rugosum could cause a 50% reduction in wheat yield (Manalil and Chauhan Reference Manalil and Chauhan2019). Information about R. rugosum interference and chickpea yield loss in eastern Australia is limited.
Knowledge regarding weed interference in crops could provide valuable information in strengthening integrated weed management practices by understanding the weak and strong points of weeds (Lemerle et al. Reference Lemerle, Luckett, Lockley, Koetz and Wu2014; Mahajan and Chauhan Reference Mahajan and Chauhan2021, Reference Mahajan and Chauhan2022; Reiss et al. Reference Reiss, Fomsgaard, Mathiassen, Stuart and Kudsk2018). Crop–weed competition and its impact on crop yield could vary with different crops, weed species, and environmental conditions (Mwendwa et al. Reference Mwendwa, Brown, Wu, Weston, Weidenhamer, Quinn and Weston2018; Soltani et al. Reference Soltani, Dille, Robinson, Sprague, Morishita, Lawrence, Kniss, Jha, Felix, Nurse and Sikkema2018). There is a common understanding that different levels of weed infestations may have different impacts on crop yield. Information about the maturity time and dispersal behavior of weeds can be used in formulating strategies aimed at reducing weed seedbanks in the soil (Walsh and Powles Reference Walsh and Powles2014), and knowledge of the seed retention behavior of weeds in a crop helps in determining strategies for harvest weed seed control (HWSC) (Walsh et al. Reference Walsh, Broster, Schwartz-Lazaro, Norsworthy, Davis, Tidemann, Beckie, Lyon, Soni, Neve and Bagavathiannan2018).
Knowledge gaps exist for the interference and seed retention behavior of B. tournefortii, R. rugosum, and S. thellungii in chickpea. Therefore, field studies were conducted for 2 yr (winter 2020 and 2021) to evaluate the impact of B. tournefortii, R. rugosum, and S. thellungii on chickpea yield loss and weed seed retention behavior.
Materials and Methods
Field Trial, Description, and Data Collection
Three field experiments were conducted for 2 yr (2020 and 2021) at the research facility of the University of Queensland, Gatton (27.5514°S, 152.3428°E). The Gatton site is situated in the subtropical climate region of Australia and has an average annual rainfall of 728 mm (30-yr climatic normal; http://www.bom.gov.au). The soil type of the experimental site was medium clay with a pH of 6.9 and 1.4% organic matter. Each experiment was repeated in the same area of the field. For sowing, a fine seed bed was prepared with a disk harrow (twice) (Disc Plow 24 Plates, Jarrett Implements, Dural, NSW, Australia) followed by a rotovator (Celli B Series Rotary Hoes 2.1m-B 205, Farm Supplies, Coopers Plains, QLD, Australia) operation. The field remained fallow after the first chickpea crop.
Each experiment was conducted in a randomized complete block design with five densities as treatments, and each treatment was replicated three times. The weed densities varied for each species. Densities of S. thellungii were 0, 5, 15, 25, and 38 plants m−2. Brassica tournefortii densities were 0, 8, 11, 14, and 19 plants m−2, and densities for R. rugosum were 0, 8, 28, 40, and 64 plants m−2. In both years, chickpea (‘PBA Seamer’, Seednet, Horsham, VIC, Australia) was planted at 30 seeds m−2 with a row spacing of 35 cm using a cone planter. The study was conducted in an additive design (weed density varied, while the crop density was kept constant) for calculating thresholds. The size of each plot was 7 m2 (5.0 m by 1.4 m).
Seeds of B. tournefortii, R. rugosum, and S. thellungii, were mixed with sand and broadcast by hand before chickpea planting. Weed seeds for field infestation were collected from fields in the eastern region of Australia in October 2019. After collection, seeds were air-dried for 7 d in a screenhouse and then stored under dark conditions at room temperature until use.
Weed seeding rates for each plot were decided based on a germination test performed in the laboratory so that a high weed infestation level could emerge in each plot. Weed density in each plot was adjusted by thinning of weed seedlings after their emergence. In the second year, the same weed density as the first year was maintained for each infestation level. The crop was sprinkle-irrigated (immediately and 7 d after sowing) to ensure uniform crop and weed emergence. Manual weeding was done regularly (twice per week) for the initial 45 d to remove other weeds and to maintain the required weed densities. Weed densities were maintained by placing (and moving) a quadrat (1 m by 1 m) throughout each plot.
At crop harvest, weed density and biomass were recorded by placing a quadrat (50 cm by 50 cm) at two random places in each plot. For weed biomass, plants from the quadrat area were cut at the soil level, placed in paper bags, and dried in an oven at 70 C for 72 h.
Before weeds were removed from the quadrat area, the total number of weed seeds in the quadrat area was also counted and then converted into seeds per square meter.
To determine the seed numbers of B. tournefortii and S. thellungii, the total numbers of pods in the quadrat of each species was counted and then multiplied by the average seed number per pod of each species. The seeds from 10 intact pods each of B. tournefortii and S. thellungii were counted and then averaged to obtain seed numbers per pod. To quantify seeds from R. rugosum, each plant within a quadrat was threshed, and the total seed numbers were estimated on a weight basis.
Before crop harvesting,, five plants of chickpea were chosen randomly from each plot (at the center of each plot) to estimate the number of pods per plot. The number of pods was counted for each plant, and the average was calculated for the five plants. For calculating seeds per pod, the seeds were counted for 10 pods chosen from each plot and then averaged to get seeds per pod. Crop harvesting was done with a plot harvester (Zurn 160 Plot Harvester, Waldenburg, Germany), and seed yield was adjusted to 12% moisture content. The crop was harvested from a 5.6-m2 (4.0 m by 1.4 m) area.
Statistical Analyses
Data for both years in each experiment were subjected to an ANOVA using the software CPCS1, verified with GenStat 19th edition (VSN International, Hemel Hempstead, UK). Year by treatment interactions were nonsignificant for each parameter; therefore, data were pooled over the years (a total of six replications) for further analysis. Data were also validated to ensure that the assumptions of normality and the homogeneity of variance were met before analysis.
The relationship between chickpea yield loss as a percent of weed-free control and B. tournefortii was estimated with a sigmoidal model using SigmaPlot v. 14.5 (Systat Software, San Jose, CA, USA):

where y is the chickpea yield loss as a percent of weed-free control at weed density x, a is the maximum yield loss, x 0 is the weed density for a 50% reduction of the maximum yield loss, and b indicates the slope.
The relationship between chickpea yield loss as a percent of weed-free control and R. rugosum and S. thellungii density was estimated with a modified hyperbolic model using SigmaPlot v. 14.5 (Systat Software):

where a represents yield loss (y) per unit weed density as x (density) approaches zero, and b is the slope.
The relationship between weed seed production and weed density was estimated with a linear model using SigmaPlot v. 14.5 (Systat Software):

where y is the weed seed production at weed density x, a is the intercept, and b is the slope.
Results and Discussion
The crop received a total rainfall of 115 and 213 mm in 2020 and 2021, respectively. In 2020, rainfall was relatively higher in September and October compared with other months (May, June, July); while in 2021, crops received higher rainfall in May and June compared with other months (July, August, September, October) (Figure 1). The mean monthly maximum temperature during the crop season ranged from 21.7 to 30.9 C and 20.5 to 25.9 C in 2020 and 2021, respectively (Figure 1). In both years, the mean monthly maximum temperature was lowest in July and highest in October. The mean monthly minimum temperature during the crop season ranged from 6.8 to 11.6 C and 7.1 to 10.1 C in 2020 and 2021, respectively (Figure 1). The mean monthly minimum temperature in September 2020 (10.4 C) was relatively higher than in September 2021 (7.4 C). Similarly, the mean monthly maximum temperature in September 2020 (27.8 C) was relatively higher than in September 2021 (23.8 C).

Figure 1. Air temperature and rainfall during the crop seasons (May–October) of chickpea for 2020 and 2021 at the University of Queensland, Gatton, Australia.
Chickpea took 10 (GDD5 [growing degree days taking 5 C as base temperature] =141) and 11 (GDD5 =140) d for emergence in 2020 and 2021, respectively. The crop took 84 (GDD5 = 914) and 93 (GDD5 = 913) d for 50% flowering in 2020 and 2021, respectively. For harvesting, the crop took 155 (GDD5 = 1,802) and 157 (GDD5 = 1,604) d in 2020 and 2021, respectively. Brassica tournefortii, R. rugosum, and S. thellungii took 14, 10, and 13 d for emergence in 2020. However, in 2021, B. tournefortii, R. rugosum, and S. thellungii took 13, 10, and 12 d for emergence.
Brassica tournefortii and Chickpea Yield
The seed yield of chickpea in the weed-free environment in the B. tournefortii interference trial was 3,200 kg ha−1, and it was reduced by 23%, 44%, 61%, and 72% at weed infestation levels of 8, 11, 14, and 19 plants m−2, respectively (Table 1). A sigmoidal model estimated that the chickpea yield was reduced by 10%, 25%, and 50% at weed densities of 5, 7, and 11 plants m−2 (Figure 2A). About 24 pods plant−1 were produced by chickpea in the weed-free plot, and the pod number per plant decreased by 11%, 31%, 37%, and 49% at weed infestation levels of 8, 11, 14, and 19 plants m−2, respectively (Table 1). Chickpea seed numbers per pod were highest (1.6 seeds pod−1) in the weed-free environment and were reduced by 37% and 56% at the infestation levels of 14 and 19 plants m−2, respectively. The 100-seed weight of chickpea in the weed-free environment was 21 g, and it decreased by 7%, 21%, and 32% at infestation levels of 8, 14, and 19 plants m−2, respectively (Table 1).
Table 1. Effect of Brassica tournefortii density on weed parameters and chickpea yield attributes and seed yield. a

a Means followed by the same letter within a column are not significantly different according to the LSD test at a 5% level of significance.

Figure 2. Chickpea yield reduction as a percent of weed-free control in response to weed densities of (A) Brassica tournefortii, (B) Rapistrum rugosum, and (C) Sisymbrium thellungii. Relationships are described with a sigmoidal model for B. tournefortii and with modified rectangular hyperbolic models for R. rugosum and S. thellungii.
The biomass of B. tournefortii at the infestation level of 8 plants m−2 was 108 g m−2, and it continuously increased with the increased weed density up to 19 plants m−2. Seed production of B. tournefortii at the infestation level of 8 plants m−2 was 114,500 seeds m−2 (Table 1), which increased by 143% and 188% at infestation levels of 14 and 19 plants m−2, respectively. At chickpea maturity, seed retention of B. tournefortii at the infestation level of 5 plants m−2 was 51%, and it remained similar (47% to 51%) at each level of infestation (Table 1). At crop harvest, B. tournefortii plants attained a height of 74 cm at a weed density of 8 plants m−2, which increased to 85 cm at a weed density of 19 plants m−2 (Table 1).
Rapistrum rugosum and Chickpea Yield
The seed yield of chickpea in the weed-free environment in the R. rugosum interference trial was 3,310 kg ha−1, and was reduced by 79%, 90%, 94%, and 96% at weed densities of 18, 28, 40, and 64 plants m−2, respectively (Table 2). A modified hyperbolic model estimated that the chickpea yield was reduced by 10%, 25%, and 50% at weed densities of 1, 2, and 5 plants m−2 (Figure 2B). About 21 pods plant−1 were produced by chickpea in the weed-free plot, and the pod number per plant decreased by 50%, 67%, 76%, and 82% at weed infestation levels of 8, 28, 40, and 64 plants m−2, respectively (Table 2). Seed numbers per pod were highest (1.7 seeds pod−1) in the weed-free environment and were reduced by 53% and 71% at infestation levels of 8 and 64 plants m−2, respectively. The 100-seed weight of chickpea in the weed-free environment was 21 g and decreased by 39%, 49%, and 51%, respectively, at infestation levels of 8, 28, and 64 plants m−2 (Table 2).
Table 2. Effect of Rapistrum rugosum density on weed parameters, and chickpea yield attributes and seed yield. a
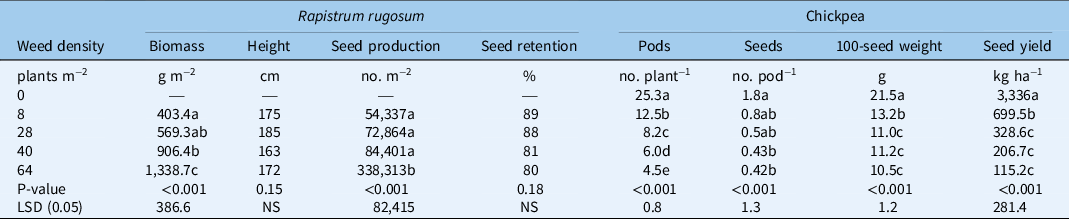
a Means followed by the same letter within a column are not significantly different according to the LSD test at a 5% level of significance.
The biomass of R. rugosum at the infestation level of 8 plants m−2 was 403 g m−2 and decreased by 55% and 70% at weed infestation levels of 40 and 64 plants m−2, respectively. Seed production of R. rugosum at the infestation level of 8 plants m−2 was 54,300 seeds m−2 (Table 2), and it increased by 522% at the infestation level of 64 plants m−2. At chickpea maturity, seed retention of R. rugosum at the infestation level of 8 plants m−2 was 89%, and it remained similar (80% to 89%) at each level of infestation (Table 2). At crop harvest, R. rugosum plants attained similar heights (163 to 185 cm) at each level of infestation (Table 2).
Sisymbrium thellungii and Chickpea Yield
The seed yield of chickpea in the weed-free environment in the S. thellungii interference trial was 3,670 kg ha−1 and was reduced by 18%, 29%, 51%, and 71% at weed infestation levels of 5, 15, 25, and 38 plants m−2, respectively (Table 3). A modified hyperbolic model estimated that the chickpea yield was reduced by 10%, 25%, and 50% at weed densities of 4, 11, and 25 plants m−2 (Figure 2C). About 26 pods plant−1 were produced by chickpea in the weed-free environment, and the pod number per plant decreased by 8%, 27%, 42%, and 52% at weed infestation levels of 5, 15, 25, and 38 plants m−2, respectively (Table 3). Seed numbers per pod were highest (1.7 seeds pod−1) in the weed-free environment and decreased significantly with increased levels of weed infestation (Table 3). The 100-seed weight of chickpea in the weed-free environment was 22 g, and it declined by 10%, 22%, and 30% at weed infestation levels of 5, 25, and 38 plants m−2, respectively. (Table 3).
Table 3. Effect of Sisymbrium thellungii density on weed parameters and chickpea yield attributes, and seed yield. a

a Means followed by the same letter within a column are not significantly different according to the LSD test at a 5% level of significance.
At the S. thellungii infestation level of 5 plants m−2, weed biomass was 31 g m−2, which further increased to 63, 82, and 102 g m−2 at infestation levels of 15, 25, and 38 plants m−2, respectively (Table 3). Sisymbrium thellungii produced 168,300 seeds m−2 at the infestation level of 5 plants m−2 (Table 3). Seed production of S. thellungii at infestation levels of 15, 25, and 38 plants m−2 increased by 80%, 245%, and 420%, respectively, compared with the infestation level of 5 plants m−2. At chickpea maturity, seed retention of S. thellungii was similar across infestation levels (45% to 50%) (Table 3). At crop harvest, S. thellungii plants attained a height of 94 cm at a weed density of 5 plants m−2, which increased to 108 cm at a weed density of 38 plants m−2 (Table 3).
This study reports the interference of B. tournefortii, R. rugosum, and S. thellungii in chickpea at various infestation levels. Based on the modified hyperbolic model, B. tournefortii, R. rugosum, and S. thellungii densities corresponding to the 50% yield reduction were 11, 5, and 25 plants m−2, respectively (Figure 2). The relationship between weed density and weed seed production for each weed species was found to be linear. The linear model estimated that B. tournefortii, R. rugosum, and S. thellungii could produce a maximum of 448,000, 206,700, and 869,400 seeds m−2, respectively (Figure 3).

Figure 3. Weed seed production in response to weed densities of (A) Brassica tournefortii, (B) Rapistrum rugosum, and (C) Sisymbrium thellungii. The lines represent a linear model fit to the data.
The yield reduction in chickpea due to the increased infestation of B. tournefortii, R. rugosum, and S. thellungii could be related to high weed–crop competition, resulting in a lower number of chickpea pods per plant and number of seeds per pod. This study also revealed that the seed retention of R. rugosum (80% to 89%) was higher compared with S. thellungii (45% to 50%) and B. tournefortii (47% to 51%), suggesting a greater opportunity for HWSC of R. rugosum.
The high level of seed production in these three brassica weeds indicates the potential of these species to become dominant weeds. The greater height of R. rugosum (∼160 cm) compared with chickpea (∼65 cm; data not provided) and high seed retention of R. rugosum at chickpea maturity make this weed an ideal candidate for HWSC. Plants of B. tournefortii and S. thellungii were found to be prone to shattering, and disturbance of plants during the harvesting operation may cause 100% seed shattering.
Chickpea is a slow-growing crop, and if weeds are allowed to grow, they compete with the crop and cause a yield loss (Bhan and Kukula Reference Bhan, Kukula, Saxena and Singh1987; Lyon and Wilson Reference Lyon and Wilson2005; Van Acker et al. Reference Van Acker, Weise and Swanton1993). As expected, we also observed chickpea yield losses due to interference of S. thellungii, B. tournefortii, and R. rugosum. This is the first study to demonstrate the chickpea yield losses due to the interference of S. thellungii. In this study, it was found that even the lowest level (5 to 8 plants m−2) of S. thellungii and B. tournefortii infestation could replenish the seedbank in the soil because of the high shattering ability of plants.
Seeds of S. thellungii (unpublished data) and B. tournefortii have greater persistence in a shallow soil layer compared with seeds buried at greater depths (Gill et al. Reference Gill, Borger and Chauhan2022; Mahajan et al. Reference Mahajan, Singh and Chauhan2020). Therefore, infestation by these weeds may increase in a no-till system if they are allowed to produce seeds in the field. Previous studies found that S. thellungii (unpublished data) and B. tournefortii emerged in multiple cohorts and produced a higher number of seeds in earlier rather than later cohorts (Gill et al. Reference Gill, Borger and Chauhan2022). These studies suggest that effort should be made to control early cohorts of S. thellungii and B. tournefortii, as they produced more seeds than late cohorts.
In our previous study, it was observed that B. tournefortii seed production declined by 33% and 78% when grown in competition with chickpea and wheat, respectively (Mahajan et al. Reference Mahajan, Singh and Chauhan2020). This information suggests that it is advisable to grow solid-drilled (narrow row spacing) crops, such as wheat and barley (Hordeum vulgare L.), if the field has a history of high infestations of B. tournefortii, to reduce its germination and establishment. A similar concept can be applied to managing S. thellungii, as wheat did not allow the plants of S. thellungii to flourish and produce seeds (unpublished data). It was observed in the previous study conducted in South Australia that B. tournefortii caused 10% wheat yield loss at a high weed infestation level of 80 plants m−2 (Gill et al. Reference Gill, Borger and Chauhan2022).
In the present study, chickpea yield was reduced by 80% at an R. rugosum density of 8 plants m−2. Previous work conducted in New South Wales, Australia, reported that R. rugosum density of 5 plants m−2 reduced chickpea yield by 40%; however, seed production and seed retention ability of this weed was not explored in that study (Whish et al. Reference Whish, Sindel, Jessop and Felton2002). The present study demonstrated that R. rugosum plants are prolific seed producers. Furthermore, this weed’s seed retention (i.e., non-shattering) and greater height relative to chickpea provide an opportunity for HWSC in chickpea. In Queensland, it was observed that 10 to 12 plants m−2 of R. rugosum in wheat fields could reduce yield by 33% to 44% (Manalil and Chauhan Reference Manalil and Chauhan2019). However, in South Australia, yield losses in wheat due to the interference of R. rugosum were found to be lower. The temperature difference between the two regions could be the reason for the variable competitive behavior of this weed in wheat. The plants of R. rugosum in South Australia were observed to be shorter in height than plants observed in Queensland (Chauhan et al. Reference Chauhan, Gill and Preston2006; Gill et al. Reference Gill, Borger and Chauhan2022). This suggests that the competitive behavior of this weed in chickpea could differ in southern Australia and would warrant further investigation.
Infestations of R. rugosum could lead to blockage of the threshing drum of the combine harvester during harvesting, as the plants are tall and stems are sturdy (Preston Reference Preston2019). Therefore, it is essential to control this weed at an early stage, otherwise, it could make mechanical harvesting of the crop difficult. A previous study reported that seeds of R. rugosum did not emerge from a 6-cm depth, and emergence was greater from 1 cm compared with other burial depths (Manalil et al. Reference Manalil, Ali and Chauhan2018). This suggests that the emergence of this weed could be restricted by employing strategic tillage in chickpea fields.
Brassica tournefortii, R. rugosum, and S. thellungii have evolved resistance to Group 2 herbicides. Therefore, relying completely on chemical control of these weeds cannot provide sustainable weed control due to the high selection pressure imposed by Group 2 herbicides (Duke and Heap Reference Duke and Heap2017). Strengthening integrated weed control strategies, such as improved crop competition, competitive cultivars, and HWSC tactics, could reduce the infestation of these weeds in chickpea fields.
In conclusion, Brassicaceae weeds, such as B. tournefortii, R. rugosum, and S. thellungii reduced chickpea yield. The percentage reduction of the crop yield was observed with weed infestation in each study. Brassica tournefortii and S. thellungii are prolific in seed production and their high shattering ability could increase the weed seedbank in the soil if these plants are allowed to produce seeds. Rapistrum rugosum is also prolific in seed production; however, the high seed retention ability of this weed makes it a good candidate for HWSC. Information generated from this study could help in the decision-making process for weed control, especially timely weed management and HWSC. The data generated from this study could also help in assessing yield and revenue losses due to the interference of these weeds in chickpea and other crops using modeling approaches.
Acknowledgments
This work was supported by funding from Grains Research and Development Corporation (GRDC), Australia. No conflicts of interest have been declared.