Introduction
In order to define the physical conditions under which drumlins form, we have studied published descriptions of drumlin fields and determined, wherever possible: (1) the nature of the large-scale bed topography on which the drumlins are found,: (2) the quality of drumlin development, (3) the spatial organization of individual drumlins in a field, (4) the characteristics of the material in the drumlins and substrate, (5) the time of drumlin formation, (6) the basal ice conditions at the time of drumlin formation, (7) the sense of strain in the ice (e.g. compressive or extending), and (8) the hydrologic conditions of the bed. We emphasize that we do not propose a model of drumlin formation, but rather attempt to define the range of physical conditions under which well-developed drumlins have formed.
Physical conditions that are always or nearly always observed in drumlin fields suggest factors that should be considered in any general theory of drumlin formation. In the same vein, physical conditions that differ appreciably between fields are presumably less important.
This study is an initial phase of an investigation of the hypothesis that there is an ice-flow instability capable of creating drumlins. Our suspicion is that when unstable preconditions are perturbed, forces are set up that cause a departure from the original state (Reference NayfehNayfeh, 1993). For example, a bump on the bed, represented by the drumlin cores that are often observed, might set up a pressure distribution or flow pattern that results in erosion adjacent to the bump or deposition over it, or both. For a drumlin to form, this perturbation not only must be present but must grow so that the resulting landform exceeds the height of the original obstacle.
Presentation of Data
Table 1 summarizes many of the characteristics investigated. Throughout the paper, drumlin fields are referred to by their numbers in this table. A lower-case superscript following a cited field number identifies a specific source. Some of the characteristics chosen for study were not usually described in the literature or were not easily measured. Others are based on necessarily indirect evidence; these characteristics are discussed but do not appear in the table. Space limitations required a selected reference list; a more complete list of drumlin references can be obtained (Reference MenziesMenzies, 1984; Reference PattersonPatterson, 1989).
Table 1. Summary of drumlin-field characteristics, Q is a subjective rating of quality of best drumlins in a field: 1 (well-formed and distinct) to 3 (poorly formed and indistinct). L is sense of longitudinal strain: ×, compressive; +, extensive. Τ is relative transverse strain using 1/r (see text). Material denotes drumlin-forming material and its structure, and Core is described by the same symbols: B, sand and gravel-bar deposits in streams; Cl, clay; Cone, concentric bedding parallel to surface; CT, clayey till: G, gravel; GM, ground moraine; IM, ice-marginal deposits; LT, lodgement till; RK, rock; S, stratified; Sd, sand; SMW, subglacial meltwater deposits; SL, sandy loam; ST, sandy till; T, till. Topo indicates topography over which drumlin-formiug ice advanced: APLAT, slightly ascending plateau (ice opposing); CSPLN, coastal plain; DESC, descending slope; DPLAT, slightly descending plateau; ISHGT, isolated height; LLND, lowland: OPPOS, opposing slope; PLAIN, plain; PLAT, plateau


Drumlin Quality
A subjective rating of quality was assigned to the drumlin fields to indicate the topographic expression and completeness of the best landforms in a given field. The rating ranges from 1 to 3; a rating of 1 means that the landforms are well-formed and distinct and 3 means that they are faint and indistinct.
Drumlins vary in length and width between fields and also within a field. However, a single form invariably dominates each area of the field, and the change to another shape is gradual. The shape has been said to vary according to topography, position with respect to the boundaries of the field, the amount of drift available, ice velocity, strain in the ice, and the drumlin-forming and substrate materials. Such situations are not documented frequently enough, however, to show that a causal relationship exists.
Drumlin elongation often varies systematically within a field, with flutes being end members. Longer streamlined features are most common down-ice from a drumlin field (9, 52, 54, 55, 74, 80b), but were also found up-ice from drumlins (48, 57) and in both locations (2).
Consistent with the pattern of flutes forming nearest the ice margin is the observation that, during a glacier retreat, flutes tend to form later than drumlins and therefore under thinner ice (11, 82–84).
Topography
Regional large-scale topography, because of its effect on ice dynamics, should be a significant variable in drumlin formation. However, our data suggest that this is not the case. Topographic descriptions were taken from the literature where available, or deduced from topographic maps. Drumlins are almost equally common on plains, on highlands, such as plateaus and isolated flat hilltops, and in confined lowlands (Table 1). Slopes do not appear to be particularly important unless they are exceptionally-steep.
Patterns of drumlin spacing and spatial organization
Distinct spatial patterns are observed in drumlin fields, and variations in drumlin density are common. One frequently mentioned spatial pattern consists of alternating concentric bands of high and low drumlin density that are perpendicular to the ice-flow direction (10, 25, 28, 52, 85, 94, 95). Typically the drumlin shapes and sizes are similar within a band Reference FairchildFairchild, 1905; Reference MullerMuller, 1963; Reference Shaw and KvillShaw and Kvill, 1984). The spacing of the bands may decrease up-glacier (25). Each band may represent a separate period of drumlin formation associated with a still stand of the ice margin during a general retreat (46b, 94b).
Other fields or areas of fields have a radial banding parallel to the ice-flow direction (4, 83, 85, 95). The outer parts of one field (94c) are characterized by radial bands, but transverse bands are present in the central part.
Often mentioned in theories of drumlin formation is the “en echelon” pattern of drumlin spacing (4, 5, 8, 9, 18, 19, 24). This is not the most commonly seen pattern, and no field is entirely “en echelon”. Other patterns of drumlin grouping arc parallel (side by side) (8, 9, 18, 85–87, 90–92), in succession down-ice (9, 85, 87, 91, 92) and overlapping or fused (4, 9, 11, 55, 87, 91, 92).
Drumlin-Forming Material
Most drumlins comprise a composite of materials of local derivation. These materials are typically glacial, but sometimes even weak bedrock may be molded into drumlin forms (52). In general, tills in drumlins tend to be rich in sand and gravel (Reference KarrowKarrow, 1981). The suggestion that drumlins are composed only of clay-rich material (Reference FairchildFairchild, 1907) has been disproved, not only in general (Reference KarrowKarrow, 1981), but also with respect to the specific area (52) studied by Fairchild (Reference MullerMuller, 1963). Three recent regional investigations note a correspondence of drumlin fields with an up-ice source of easily erodible, fine-grained, sedimentary rock (Reference Aylsworth and ShiltsAylsworth and Shilts, 1989; Reference BouchardBouchard. 1989; Reference CoudéCoudé, 1989).
Almost half of the drumlins having exposed interiors have distinct cores. The core may be almost anything: an older, denser till: stratified sand and gravel, or gravel and till: stratified clay; or solid rock. These observations demonstrate that some drumlins are partly erosional in origin. However, others clearly have a depositional component. Any general theory of drumlin formation must accommodate both possibilities.
In some drumlinized areas, small topographic perturbations that might have formed cores did not become drumlins (Reference FairchildFairchild, 1907; Reference AronowAronow, 1959; Gluckert, 1973; Reference GillbergGillberg, 1976). Thus the presence of a perturbation alone, even within a drumlin field, appears not to be sufficient to cause drumlin formation.
Substrate Material
The substrate is defined as the bedrock or sediment on which drumlins were deposited. Surrounding material that was deposited at or after the time of drumlin formation is not considered to be part of the substrate, but material in a drumlin core that is laterally continuous is considered to be part of the substrate.
Unconsolidated sediments make up 34% of the substrates, 18% being till and 16% being stratified sediments, typically of outwash origin. The remaining 66% are rock. One-third of the rock substrates are shales and slates, one-third crystalline rocks, and one-quarter carbonates, with sandstones, conglomerates and basalts making up the remainder. In short, drumlin development is not obviously linked to lithology of the substrate.
In many fields the density of drumlins decreases with decreasing depth of glacial sediment (40, 41, 52, 58, 64, 69, 72). In addition, well-formed drumlins tend to occur on thicker sediment, while imperfect, poorly shaped drumlins of lower relief occur in areas of thin sediment (40, 72a, 81b). In other fields, however, the amount of loose debris overlying the bedrock does not appear to be an important variable (68, 72b, 85–92).
Time of Formation
While it is often possible to determine the relative ages of various features within a drumlin field, the lack of absolute ages for the original deposition of drumlin-forming material and the difficulty of determining whether the drumlin material is of the same age as the drumlin formation make interpretations of timing difficult. In addition, drumlins in different parts of a field may have formed at different times.
The most likely time for drumlins to form (or to form and then be preserved) appears to be during the final stages of advance and the early stages of retreat of the ice mass. Where ice-movement directions are inferred to have been different during advance and retreat, drumlins are aligned with the retreat direction Reference FairchildFairchild, 1907; Reference LundqvistLundqvist, 1970).
Drumlin fields in North America are no older than late-Wisconsinan. and all preserved drumlin fields of significant size appear to have formed during this time period in the late Pleistocene. If drumlins formed during earlier glacial time, they probably were eroded or masked by subsequent glaciations. Only three fields, consisting of a few very small drumlins. are described from the Holocene (1, 3, 18).
Basal Conditions
Drumlins apparently form in a restricted zone somewhat up-glacier from, and separated from, the associated ice margin. Normally there is a drumlin-free zone immediately up-glacier from the moraine. Drumlin belts occur 10–80 km (95c), 2–16 km (57c), 5–50 km (96), 2–10 km (Reference Dardis, McCabe and MitchellDardis and others, 1984), 20–30 km (48), 15–20 km (5),. and at least 25 km (46) behind the associated moraine. The drumlin-free zone may be due to some minimum ice thickness being required for drumlin formation. The Warwick-Tokio field (57) may be an exception to this rule if the drumlins and moraine are contemporaneous.
Temperature modeling suggests that steady-state basal temperatures in such areas were probably at the melting point (Reference Moran, Clayton, Hooke, Fenton and AndriashrkMoran and others, 1980). This follows from the fact that temperatures in polar glaciers increase rapidly with depth in the ablation zone (e.g. Reference HookeHooke, 1977). Ice can, of course, advance over a zone of permafrost, leading to non-steady-state, sub-freezing, basal temperatures that can persist for a period of some decades or perhaps even centuries. But the observation (previous sub-section) that drumlins seem to form during late phases of an ice advance suggests that thermal conditions may have had time to reach the steady state.
Drumlins are found as far south as 4° Ν in North America and 46° Ν in Europe, and as far north as 53° S in the Southern Hemisphere (Fig. 1). Although thawed-bed conditions existed beneath much of the ice sheet, these latitudes suggest that frozen margins may have been present. Such frozen margins can be up to 2–3 km wide (Reference Moran, Clayton, Hooke, Fenton and AndriashrkMoran and others, 1980).
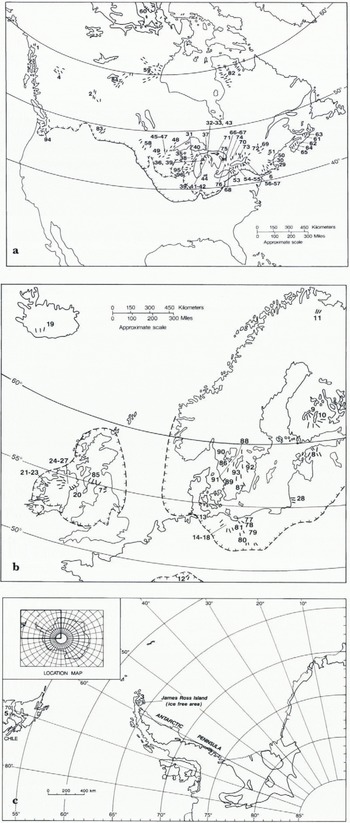
Fig. 1. Locations of the drumlin fields considered in this study in (a) North America, (b) Europe, and (c) Antarctica and Chile. Numbers keyed to Table 1. ‴ General trend of drumlins. ⊥ ⊥ ⊥ Limit of late-Wisconsinan or correlative ice advance. ⊥⊥⊥⊥⊥ Maximum Pleistocene ice advance in North America.
Glacial-thrust features are additional evidence of frozen margins (Reference Moran, Clayton, Hooke, Fenton and AndriashrkMoran and others, 1980). These features apparently form when the bed becomes frozen to the glacier sole near the margin, while melting conditions exist somewhat farther up-glacier. In North America, late-Wisconsinan thrust features are common in North Dakota (Reference Moran, Clayton, Hooke, Fenton and AndriashrkMoran and others, 1980), western Canada from southern Saskatchewan to east-central Alberta (Reference KupschKupsch, 1961) central to northern Minnesota (Reference MooersMooers, 1990) and Ontario (Reference CrozierCrozier, 1975; Reference Gray and LauriolGray and Lauriol, 1985), all of which are major drumlin areas.
Secondly, in the ablation zones of glaciers and ice sheets with frozen margins there is generally a significant concentration of surficial debris (Reference Goldthwait, Goldthwait and GoldthwaitGoldthwait and others, 1951; Reference BoultonBoulton, 1968, Reference Boulton and Goldthwait1971; Reference Moran and GoldthwaitMoran, 1971; Reference HookeHooke, 1973; Reference MenziesMenzies, 1979). Hummocky moraine and dead-ice topography form as a result of this debris. Such topography is characteristic of moraines down-ice from drumlin fields.
Thirdly, paleoclimatic reconstructions provide an approach to assessing the condition of the ice margin, since a mean annual temperature of less than −5°C but not much lower than −10°C is probably needed to form a frozen margin of significant (2 km) width, (Reference Moran, Clayton, Hooke, Fenton and AndriashrkMoran and others, 1980). For example, a yearly average temperature of below ×2°C is needed for permafrost, and as a rule permafrost is present everywhere north of the −6° to −8°C isotherms (Reference Williams, Wright and MoseleyWilliams, 1975). In areas of drumlin formation in Europe, the mean annual temperature was appreciably below −2°C and possibly as cold as −12 °C (Reference FrenzelFrenzel, 1973). In the U.S.A., the upper Midwest (North Dakota; Minnesota) probably had a mean annual temperature between −7 ° and −10°C with low accumulation, favoring permafrost development (Reference Barry, Wright and PorterBarry, 1983). Thus permafrost is likely to have bordered the ice sheet in areas where drumlins formed. Continuous permafrost existed in Wisconsin from 25000 to 13000 BP (Reference Clayton and AttigClayton and Attig, 1989).
A frozen margin in and of itself cannot be a requirement for drumlin formation, however, because many lobes that formed drumlins were floating at the terminus (1, 19, 22–26, 28, 32, 36, 38, 60–65, 70, 81, 84). One key characteristic that marine-based ice lobes probably shared with those that had frozen margins is high pore-water pressure. In the case of the marine-based lobes, this would have been provided simply by the height of the water surface against a calving face.
Ice Thickness and Basal Shear Stress
Since drumlins formed relatively near ice margins, the ice under which they developed cannot have been very thick. The lobate shape of many ice margins associated with drumlin fields also indicates that the ice was thin and controlled by topography. Precise estimates of ice thickness at the time of drumlin formation are few.
In the case of the Holocene drumlins of southern Iceland (18), the ice was 100–150 m thick over the drumlin field during the maximum historical extension. Drumlins are absent from the terminal 500 m of the glacier forefield where the maximum ice thickness was less than 100 m (Kruger and Thomsen, 1984).
Nunataks provide a record of ice thicknesses over drumlins. In one case (82) a maximum thickness of 400 m was found, and in another (94b) the thickness ranged between 140 and 440 m. A reconstruction of the Puget lobe (93) yielded a maximum ice thickness of 200–300 m at the terminus and 1100–1200 m at 250 km from the margin. Drumlins formed throughout this zone.
Assuming a parabolic profile and constant basal shear stress, the shear stresses in the above examples are 0.15 bar (82), 0.11–0.35 bar (94) and 0.14–0.22 bar (93). In the last case, the calculated shear stress depends on whether the drumlins formed all at one time or successively as the ice retreated. These basal shear stresses suggest that ice flow may have been relatively sluggish at the time of drumlin formation. However, it is also possible that the low shear stresses implied by thin ice reflect the weakness of the bed over which the ice was moving.
Sense of Strain in the Glacier
Longitudinal compression is characteristic of ablation areas of glaciers. On present-day ice caps and ice sheets the ablation area comprises approximately the outer 10% of the flowline. Most drumlin fields arc located sufficiently near a contemporaneous ice margin to ensure that they formed beneath the ablation area.
Although drumlin patterns are not always simple and symmetric (Reference SlaterSlater, 1929; Reference GillbergGillberg, 1976), the sense of transverse strain can usually be determined by the pattern of drumlins within a field. Fan-shaped fields result from transverse extension and funnel-shaped fields from transverse compression.
In a cylindrical flow field, the circumferential (or transverse) strain rate, εθ is u/r (Reference NyeNye, 1953) where u is the ice velocity in the down-glacier direction and r is the distance from the axis of the cylinder. However, the velocity of the ice under which the drumlins formed is unknown, and r varies. Thus, to obtain a more quantitative measure of relative transverse strain rates, we chose a point where two typical drumlins were 1 km apart, drew radii parallel to the axes of these drumlins and measured the distance from either of the drumlins to the point of intersection of the radii. Lines converging in an up-ice direction define a positive value for r, and diverging lines define a negative value. The reciprocal of this distance, 1/r, is then equal to εq/u for that particular location, and to the extent that u does not vary excessively among the various drumlin-forming situations, the ratio εθ/u is a measure of the relative value of εθ . This measurement was made wherever fields were adequately mapped and drumlin orientations sufficiently consistent to make a meaningful measurement.
In the drumlin fields studied. 1/r ranges from −0.22 to 0.08, with more than half the values falling between 0.01 and 0.02 (Table 1; Fig. 2). Thus, the majority of drumlin fields apparently formed under conditions of transverse extension. This may be simply because they are located in the ablation area, where transverse extension is common, but the cluster of 1/r values between 0 and 0.02 may be significant. Only three fields considered here (2, 6, 59) have negative values. In one of these (59) the ice involved may have been an ice stream.

Fig. 2. Histogram of 1/r values for the drumlin fields measured. The ratio 1/r is a measure of relative transverse strain rate. Positive values indicate transverse extension. See text for explanation.
The Role of Water
Evidence for the presence of subglacial water at the time of drumlin formation is theoretical and/or indirect. As noted earlier, temperature models suggest that the beds of land-based lobes, in the region of drumlin formation somewhat up-glacier from the margins, were probably at the pressure-melting temperature. In the case of marine-based lobes, subglacial temperatures were certainly at the pressure-melting point.
In both cases, pore-water pressures were probably high. In the case of land-based glaciers, as noted, drumlin areas border margins that were probably in the permafrost zone. Frozen margins would have blocked the release of meltwater, thus increasing subglacial water pressures. Reference Moran, Clayton, Hooke, Fenton and AndriashrkMoran and others (1980) attribute the formation of glacial-thrust terrane, often found down-glacier from drumlin fields, to high pore-water pressures. Pore-water pressures were probably also high in the case of marine-based margins, particularly if the water was deep.
The thin-ice profiles reconstructed above imply low basal shear stresses. A weak bed, resulting from high pore-water pressures would be consistent with these observations. There are a few documented cases of drumlins containing deformation structures such as diapirs or dikes (e.g. 95). Such features can be created by the flow of saturated sediment as a result of weakening of the sediment by high pore-water pressure.
The presence of fluvial sediment beneath drumlins does not necessarily mean that the fluvial deposition was contemporaneous with drumlinization. Stratified deposits lapping onto a drumlin in its lee (Reference Chapman and PutnamChapman and Putnam, 1966; Reference Dardis, McCabe and MitchellDardis and others, 1984) require the drumlin for their accumulation, but there is no evidence that the fluvial activity and drumlin formation were related. However, concentrically bedded, fluvially deposited, upper layers that conform to the drumlin profile, as in the Livingstone Lake field (83), suggest that fluvial accumulation may have been an integral part of the later phases of the formation of some drumlins. Likewise, the common association of eskers (8, 9, 24, 26, 29, 30, 37, 46, 72, 81–83, 88) and, less commonly, tunnel valleys (46, 83, 94; Reference Grube and EhlersGrube, 1983), with drumlins is not conclusive evidence for the presence of water at the time of drumlin formation, because some drumlins are known to pre-date these features. However, eskers and tunnel valleys are indicative of subglacial drainage shortly after drumlin formation. Although these observations do not relate conclusively to the subglacial hydrology during drumlin formation, they suggest that water was present soon after formation.
In summary, high pore-water pressures likely existed in all of the drumlin fields studied, because at least one of the following is true: (1) a frozen toe was probably present; (2) the glacier terminated in water; (3) subglacial drainage followed drumlin formation; (4) fluvial deposits are an integral part of the drumlin form; or (5) the till is deformed.
Conclusions
Parameters studied that were significantly variable were (1) regional topography, (2) material in drumlins, (3) substrate lithology, and (4) substrate thickness. These, therefore, are not central to drumlin formation.
Shared characteristics are linked to ice physics. Drumlins were formed within the ablation area relatively-near ice margins. As a result, the longitudinal strain rate was normally compressive and the transverse strain rate extending. The ice must have been relatively thin. The drumlin-free zone between the moraine and the drumlin field suggests, however, that there is a limit to how thin the ice can be and still form drumlins. This drumlin-free zone varies from 2 to 25 km in width.
In many drumlin fields a frozen toe was probably present. Evidence for this includes paleoclimatic reconstructions, ice-thrust features and areas of stagnation moraine. Such a frozen toe would be likely to cause high pore-water pressures in the area of drumlin formation. Frozen toes could not have been present where ice sheets terminated in water, but the presence of an appreciable depth of water at the terminus would also have led to high pore-water pressures farther up-glacier. No drumlin fields were found in areas where elevated pore-water pressures would have been unlikely.
Within fields, two common patterns of drumlin spacing can be distinguished: transverse and radial banding. Transverse banding is the more common and may be a result of time-transgressive formation of drumlins during retreat of an ice margin. One drumlin form typically dominates an area within a field, and the change to another form is gradual. Drumlins normally become elongated down-ice.
Acknowledgements
In the evolution of our ideas on drumlins, we have benefited from discussions with D. Mickelson, H. Mooers, J. Shaw and R. Shreve. The critical comments of D. MacAyeal significantly improved the presentation. This research was supported in part by the University of Minnesota Graduate School.