Introduction
Avian schistosomes of the genus Trichobilharzia are digenean parasites occurring worldwide, with an increasing presence at northern latitudes – for example, Denmark, Norway and Sweden (Thors & Linder, Reference Soleng and Mehl2001; Larsen et al., Reference Kumar, Stecher, Li, Knyaz and Tamura2004; Soleng & Mehl, Reference Soldánová, Selbach, Kalbe, Kostadinova and Sures2011; Tracz et al., Reference Thors and Linder2019; Al-Jubury et al., Reference Al-Jubury, Kania, Bygum and Buchmann2020). Several species within the genus are of medical importance as their cercariae cause cercarial dermatitis (swimmer's itch) in humans (Horák et al., Reference Horák, Mikeš, Lichtenbergová, Skála, Soldánová and Brant2015; Christiansen et al., Reference Christiansen, Olsen, Buchmann, Kania, Nejsum and Vennervald2016; Zbikowska & Marszewska, Reference Wesenberg-Lund2018; Tracz et al., Reference Thors and Linder2019; Al-Jubury et al., Reference Al-Jubury, Kania, Bygum and Buchmann2020). The first connection between bird schistosomes and swimmer's itch was described from Michigan, USA, almost a century ago (Cort, Reference Cort1928). In contrast, Denmark's first case was reported three decades later (Berg & Reiter, Reference Berg and Reiter1960).
The adult bird schistosomes occupy the visceral veins (visceral species) or the nasal mucosa (nasal species) of birds, where they release eggs that subsequently hatch and release ciliated larvae (miracidia) to the aquatic environment. They penetrate the intermediate pulmonate snail host and transform to mother sporocysts, which subsequently produce daughter sporocysts releasing furcocercariae able to penetrate the skin of the definitive host (waterfowl) for completion of the life cycle (Horák et al., Reference Horák, Kolářová and Adema2002). Bird schistosomes are zoonotic trematodes as their cercariae may not only seek birds but also penetrate the skin of humans in the freshwater lake due to the similarity in skin lipid composition between aquatic birds and humans (Haas & Van de Roemer, Reference Haas and Van de Roemer1998). The resulting disease is termed cercarial dermatitis due to marked skin reactions with an itchy maculopapular eruption induced by the dying or dead parasite larvae (Haas & Pietsch, Reference Haas and Pietsch1991; Horák & Kolářová, Reference Horák and Kolářová2000, Reference Horák and Kolářová2001; Bayssade-Dufour et al., Reference Bayssade-Dufour, Vuong, René, Martin-Loehr and Martins2002). Re-infections of humans are generally more pronounced, possibly due to sensitization and allergic responses, whereby swelling of larger skin areas, fever and nausea can be seen (Kolárová et al., Reference Kolářová, Horák and Fajfrlík2013; Tracz et al., Reference Thors and Linder2019).
Recently, the occurrence of bird schistosomes and swimmer's itch has become a more frequent problem in recreational areas (Buchmann et al., Reference Buchmann, Larsen and Bresciani2004; Soldánová et al., Reference Skírnisson, Aldhoun and Kolářová2013; Jouet et al., Reference Jouet, Skírnisson, Kolářová and Ferté2015; Marszewska et al., Reference Marcogliese2016; Al-Jubury et al., Reference Al-Jubury, Kania, Bygum and Buchmann2020). Of special concern is the finding that larvae of certain visceral avian schistosome species have been detected in the lungs of experimentally infected murine hosts (Horák et al., Reference Horák, Kolářová and Adema2002), and feet paralysis has been seen in mice infected with the neurotropic species Trichobilharzia regenti (Horák et al., Reference Horák, Kolářová and Dvořák1998; Hrádková & Horák, Reference Hrádková and Horák2002). Therefore, it is important to survey the occurrence of parasites at the species level in lakes attended by the public for recreational purposes. For further risk analysis, it is relevant to correlate the parasite occurrence with physicochemical environmental factors in the lakes, as the parameters such as pH, alkalinity, nitrogen, phosphorous, chlorophyll and conductivity were previously claimed to affect the survival of the intermediate host snails and cercariae (Hunter & Wigington, Reference Hunter1972; Madsen et al., Reference Loy and Haas1988; Marcogliese, Reference Madsen, Daffalla, Karoum and Frandsen2001). The present study presents the results from an extensive survey, covering a representative part of the Danish lake territory, elucidating the occurrence of avian schistosome species in the lakes with different physicochemical conditions.
Materials and methods
Lake sampling and snails examined
From August 2018 to October 2020, a total of 10,225 pulmonate freshwater snails (Lymnaea stagnalis, Radix (syn. Ampullaceana) balthica, Radix auricularia, Stagnicola palustris, Omphiscola glabra, Planobarius corneus, Planorbis planorbis, Anisus vortex, Bithynia tentaculata, Bithynia leachii) were collected from 39 (natural and artificial) freshwater lakes distributed all over Denmark (table 1).
Table 1. Lake names, locations in Danish main regions (Zealand, Jutland, Funen and Lolland), coordinates and surface area of freshwater lakes examined.
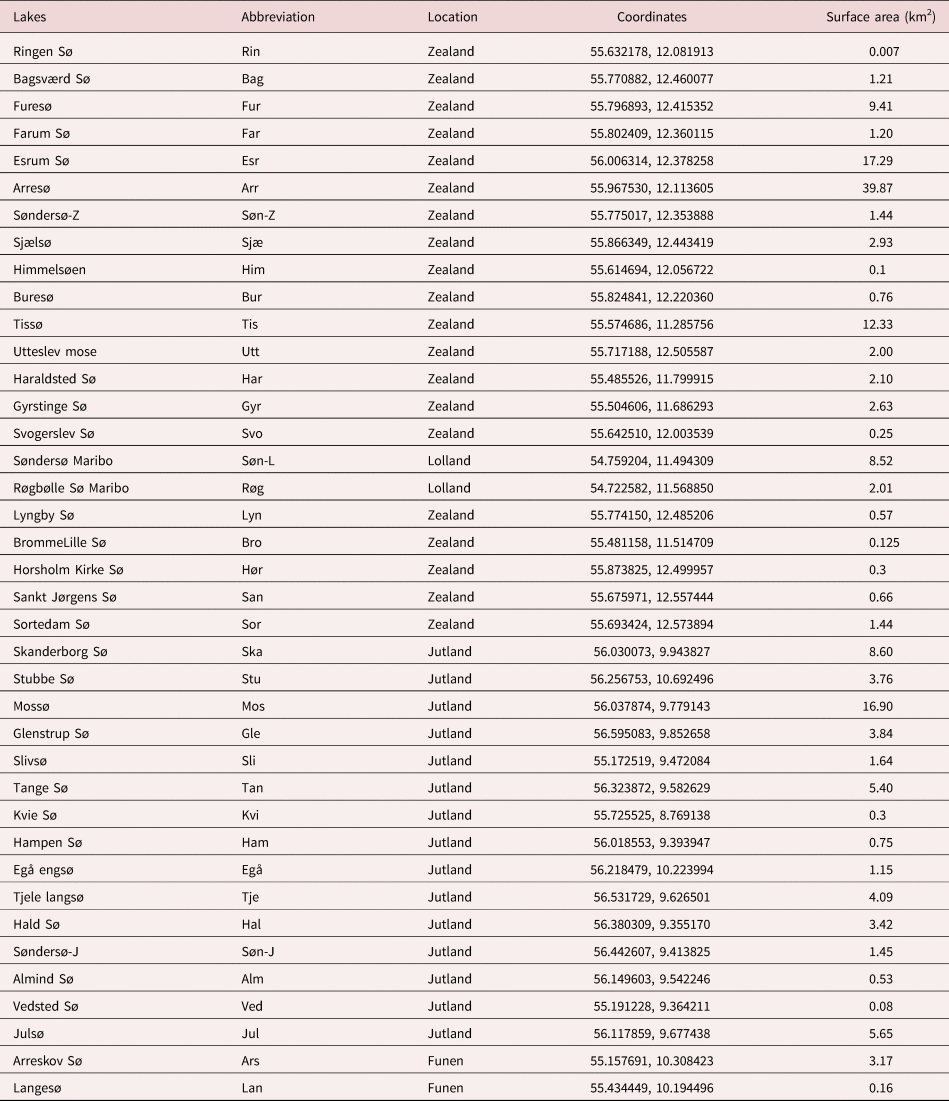
Snails were collected randomly by hand or forceps from stones and aquatic plants along several parts of the shore in shallow water (minimum three localities of each individual lake). Snails were subsequently transported to the Laboratory of Aquatic Pathobiology, University of Copenhagen, for further examination. The sampled snails were rinsed by dechlorinated water for removal of surface adhering organisms (preventing interference of false positive results), after which each snail was identified to species level based on morphometric features (Glöer, Reference Glöer2002), and subsequently placed separately in a 200 ml plastic beaker containing 100 ml dechlorinated water. All beakers with snails were placed in natural light and room temperature overnight. The following morning all Trichobilharzia-positive snails were identified by examining the water in the beaker using a dissecting microscope (Leica CLS 150X, Leica Microsystems, Wetzlar, Germany, magnification ×4–40).
Cercariae
The cercarial type and genus were determined morphometrically based on standard morphometric characters (Wesenberg-Lund, Reference Van Donk, Gulati and Grimm1934; Schell, Reference Rudolfová, Littlewood, Sitko and Horák1970; Horák et al., Reference Horák, Kolářová and Adema2002; Marszewska et al., Reference Marcogliese2016). Specimens identified to genus level as Trichobilharzia sp. were isolated and preserved in 70–96% ethanol and kept at 4°C for subsequent molecular species identification.
Bird collection
A total of 12 specimens of waterfowl were subjected to examination for the presence of bird schistosomes. Nine mallards (Anas platyrhynchos, three males and six females, mean body weight 1200 g) were shot by a hunter in Roskilde municipality, Denmark, in 2019 and were within one day transported to the Laboratory of Aquatic Pathobiology at the University of Copenhagen for parasitological examination. In addition, three specimens of mute swan Cygnus olor were found dead at three different localities (Vanløse and Roskilde in Zealand and Norre Alslev in Lolland). They were collected and treated likewise. All birds were frozen at –18°C until autopsy and parasitological examination.
Parasitological examination of birds
The dissection was performed according to Skírnisson & Kolářová (Reference Sengupta, Hellstrom and Kariuki2008) with some modifications: the gizzard was cut open, muscles and content were checked by the naked eye, while heart, liver, lung and pancreas were pressed carefully and individually placed in a sieve and washed with tap water. The samples were transferred to 1000 ml beakers filled with approximately 700 ml tap water. After 60 min, the water and the suspension was then examined under the dissecting microscope (Leica CLS 150X) for recovery of fragments or complete adult worm. The spinal cord and cerebral membranes were also carefully removed and placed in a petri dish with phosphate-buffered saline and examined. Direct faecal and nasal mucosal tissue examination were performed by placing the stool or mucosa sample on a slide covered with a coverslip and subsequently checked under a compound microscope (Leica DM5000B, Leica Microsystems, Wetzlar, Germany). Digital images was obtained using a Leica MC170 HD camera.
DNA extraction and polymerase chain reaction (PCR)
DNA extraction from cercariae
DNA was extracted from fresh or alcohol-preserved single furcocercaria with a QIAamp® DNA Mini Kit (QIAGEN GmbH, GIAGEN strasse, Hilden, Germany) following the manufacturer's instruction protocol, with a minor modification (DNA was eluted in 50 μl of elution buffer instead of 200 μl).
DNA extraction from waterfowl positive faecal and mucous samples
DNA was extracted and purified from the individual positive faecal samples using the QIAamp® Fast DNA Stool Mini Kit (QIAGEN), while the QIAamp® DNA Mini Kit was used for DNA extraction from the positive mucus samples (one mallard was positive for parasite eggs in the nasal mucosa), as described above.
Molecular identification
By PCR, internal transcribed spacer 1 (ITS1)-5.8S-ITS2 regions of the ribosomal DNA (rDNA) was amplified using the forward primer BD1 (5′AGG AAT TCC TGG TAA GTG CAAG′3) and the reverse primer BD2 (5′TAT GCT TAA ATT CAG CGG GT′3) (Galazzo et al., Reference Galazzo, Dayanandan, Marcogliese and McLaughlin2002). Reactions were run in a T100™ Thermal Cycler (BioRad, Copenhagen, Denmark) in a total reaction volume of 60 μl containing: 1 μM of each primer (TAG Copenhagen, Denmark), 1 mm of dNTP Mix (Life Technologies, Copenhagen, Denmark), 1.25 units of BIOTAQ DNA polymerase (Saveen Werner Aps, Copenhagen, Denmark), 1.5 mm magnesium chloride, and 6 μl 10 × PCR buffer and 2 μl DNA template. Finally, DNase- and RNase-free water (Invitrogen™, Life Technologies, Copenhagen, Denmark) was added to a final reaction volume of 60 μl. Sterile water was used as negative control. The following PCR protocol was used: initial denaturation at 94°C for 5 min, followed by 45 cycles of denaturation at 94°C for 30 s, annealing at 57°C for 30 s and elongation at 72°C for 75 s. After cycling, a final elongation step at 72°C for 7 min was performed. The PCR products were visualized by gel electrophoresis and subsequently purified using Illustra™ GFX™ PCR and Gel Band Purification Kit (VWR, Copenhagen, Denmark). DNA concentration and purity were measured using a Nanodrop 2000 spectrophotometer (Saveen & Werner ApS, Copenhagen, Denmark). Fragments were sequenced by Macrogen Inc. (Amsterdam, the Netherlands) using the PCR primers used for the amplification process.
In order to identify species with high molecular identities, the obtained sequences were subjected to BLAST analysis at the National Center for Biotechnology Information (NCBI). Further, the annotations were established by a combination of using the two web-based tools Rfam at http://rfam.xfam.org/search – tabview = tab1 at Nucleic Acids Research web site (2020) and the ITS Database at http://its2.bioapps.biozentrum.uni-wuerzburg.de/ (Ankenbrand et al., Reference Ankenbrand, Keller, Wolf, Schultz and Forster2015). In cases where 18S and 28S ribosomal sequences were too short, alignment to closely related and highly identical sequences with sufficient parts of 18S and 28S supplemented the web-based tools.
Phylogenetic and molecular evolutionary analyses were conducted using the software MEGA version X (Kumar et al., Reference Krist, Kay, Larkin and Neiman2018). Initially, an alignment was constructed using Muscle and including three non-Trichobilharzia trematodes species (Tylodelphys clavata (MW135143), Diplostomum paracaudum (MW135073) and Posthodiplostomum cuticola (MW135111)) as an outgroup. Muscle was chosen because Clustal W was not capable of aligning the included 5.8S ribosomal RNA (rRNA) properly. Due to the presence of a variable number of repeats in the ITS1 of Trichobilharzia anseri and Trichobilharzia szidati, some obvious editing of the alignment was necessary. Subsequently, the best fitting model of the 24 models tested by MEGA version X was, when using the Akaike criterion, General Time Reversible + G. The evolutionary history was inferred by using the maximum likelihood method and General Time Reversible model (Nei & Kumar, Reference Marszewska, Cichy, Heese and Zbikowska2020). The tree with the highest log likelihood (−8272.16) was chosen. Initial tree for the heuristic search were obtained automatically by applying Neighbour-Join and BioNJ algorithms to a matrix of pairwise distances estimated using the maximum composite likelihood approach, and then selecting the topology with superior log likelihood value. A discrete Gamma distribution was used to model evolutionary rate differences among sites (five categories (+G, parameter = 1.4441)). The tree is drawn to scale, with branch lengths measured in the number of substitutions per site.
Lake physicochemical parameters
Online data (ODA) at https://odaforalle.au.dk, delivered by the municipalities for the lakes investigated, were accessed and six parameters (temperature, pH, alkalinity, nitrogen, phosphorus, chlorophyll and conductivity) were extracted. Data in ODA are collected as part of the National Monitoring Program for the Aquatic Environment and Nature (NOVANA), the Danish Environmental Protection Agency. The data have been through quality assurance before being made available online. We then performed a Spearman correlation coefficient test between the parameters and the occurrence of infections (infected prevalence of snails and number of human cases).
Results
Lakes and snails
A total of 10,225 freshwater snails from 39 different freshwater localities distributed in Zealand (22), Jutland (15) and Funen (2) were collected (fig. 1). The freshwater snails collected and examined in this study were belonging to three families and ten different species: Lymnaeidae (L. stagnalis (2524), R. balthica (3175), R. auricularia (72), S. palustris (1078) and O. glabra (10)), Planorbidae (P. corneus (899), P. planorbis (27), A. vortex (1851)) and Bithyniidae (B. leachii (66), B. tentaculata (523)). The samples were dominated by R. balthica, followed by L. stagnalis and S. palustris. Eight snail species were sampled in Furesø, seven species in Farum Sø, six species in Bagsværd Sø and Esrum Sø, five species from Søndersø Zealand, Buresø, Haraldsted Sø, Hørsholm kirke Sø and Skanderborg Sø. Other lakes, such as the artificial lakes Ringen Sø and Svogerslev Sø, merely contained one snail species, L. stagnalis and R. balthica, respectively.

Fig. 1. Sampling locations (number) in Danish (natural and artificial) freshwater lakes. Lake name and sampling size (x) reported as follows (lakes written in bold are negative): 1. Ringen (359), 2. Bagsværd Sø (495), 3. Furesø (1270), 4. Farum Sø (766), 5. Esrum Sø (1600), 6. Arresø (92), 7. Søndersø-Z (197), 8.Sjælsø (203), 9.Himmelsøen (30), 10.Buresø (88), 11.Tissø (296), 12.Utterslev mose (81), 13.Haraldsted Sø (234), 14.Gyrstinge Sø (30), 15.Svogerslev Sø (8), 16.Søndersø Maribo (201), 17.Røgbølle Maribo (217), 18.Lyngby Sø (250), 19.BrommeLille Sø (135), 20.Horsholm kirke Sø (182), 21.Sankt Jørgens Sø (255), 22.Sortedam Sø (169), 23.Skanderborg Sø (496), 24.Stubbe sø (116), 25.Mossø (100), 26.Glenstrup Sø (490), 27.Slivsø (137), 28.Tange Sø (209), 29.Kvie Sø (162), 30.Hampen Sø (140), 31.Egå Engsø (183), 32.Tjele langsø (287), 33.Hald Sø (181), 34.Søndersø-J (151), 35.Almind Sø (18), 36.Vedsted Sø (36), 37.Julsø (22), 38.Arreskov Sø (183), 39.Langesø (156).
Prevalence of Trichobilharzia infection in snails in the investigated lakes
Thirteen lakes were positive with regard to Trichobilharzia spp. (cercarial shedding), seven of them located in Zealand and six in Jutland. The remaining 26 locations were negative for Trichobilharzia spp. – as judged from cercarial shedding from snails. Lakes (written in bold in fig. 1) were positive for Trichobilhariza cercarial shedding. Of the 10,225 snails, 37 released Trichobilharzia cercariae, resulting in an overall prevalence of 0.4%. Lymnaea stagnalis and Radix balthica were the only snail species found infected with Trichobilharzia spp. (overall prevalences 1 and 0.4%, respectively). The highest total prevalence (6%) was recorded in Ringen Sø, followed by Arresø (4.9%). Cercariae released from snails in the positive lakes were identified both morphologically and molecularly.
Furesø and Hørsholm Kirke Sø were the only lakes in which two Trichobilharzia species were found. In those lakes, T. franki and T. regenti were isolated from four individual snails (prevalence 0.4%, 0.4% and 0.75% and 0.75%, respectively). The remaining positive lakes hosted only one species of Trichobilharzia (table 2). Cercariae released from snails in Ringen Sø, Mossø and Tjele langsø were identified as T. szidati, while cercariae from Bagsværd Sø, Furesø, Hørsholm kirke Sø, Himmelsø, Glenstrup Sø, Kvie Sø and Egå Engsø were T. franki.
Table 2. Overall prevalence of 13 Trichobilharzia spp.-positive lakes (snails shedding) with species identification.

Consult table 1 for abbreviations of lake names.
Prevalence of infection in birds
Four of the 12 birds (33.3%) were positive for avian schistosome eggs. Three A. platyrhynchos (one male and two females) were positive for Trichobilharzia spp. (egg screening). Subsequent molecular sequencing of DNA recovered from faeces showed that one female bird and one male bird carried T. szidati, while the other female carried eggs identified as T. regenti in the nasal mucosa (table 3) . One swan (C. olor) was positive for Trichobilharzia sp. by faecal examination. Identification to the genus level was done based on the egg morphology and molecular characteristics (Skírnisson & Kolářová, Reference Sengupta, Hellstrom and Kariuki2008), as the PCR was not conclusive at the species level.
Table 3. Overview of isolated specimens of Trichobilharzia spp. identification, lake and GenBank accession number.

Molecular identification of cercariae
Cercariae released from 15 infected snails in the positive lakes and three samples from birds were identified molecularly and have been assigned GenBank accession numbers from MW482435 to MW482450, MW538530 and MW538531. At NCBI, the BLAST tool was used to reveal similarity/identity to sequences submitted to GenBank prior to this study (supplementary table S1). In addition, phylogenetic analysis was performed (supplementary fig. S2). Seven cercariae were identified as T. franki in Furesø, Bagsværd Sø, Hørsholm Kirke Sø, Himmelsøen, Kvie Sø, Egå Engsø and Glenstrup Sø. Five sequences identified the samples as T. szidati. Two of these were from cercariae in Ringen Sø, and one in Mossø. Two T. szidati sequences were from birds samples in Roskilde municipality. Three sequences identified the samples as T. regenti in Furesø, Hørsholm Kirke Sø and Roskilde municipality (bird sample). Three cercariae were identified as T. anseri in Arresø, Hampen Sø and Farum Sø (for more details, see table 3). The sequences from the T. anseri specimens were difficult to align to each other. First of all, sequences designated T. anseri covering either ITS1 or ITS2 – but not both – were available at GenBank. However, three sequences (FJ469784, FJ469785 and FJ469791), designated as Trichobilharzia sp., contained parts that were identical to ITS1 and ITS2 sequences of T. anseri at GenBank. In addition, these sequences included a part of 18S, the complete 5.8S and a part of 28S. Secondly, a repeated sequence (105 bp long) in ITS1 occurred in one, three, five or seven copies. Thirdly, MW482445 of this study included a 151-bp-long insertion in ITS1. Finally, another sample (MW538530) from Farum Sø identified as T. anseri (MW538530) had a stretch of 503 bp double sequence across the insertion mentioned above, thus resulting in a gap. The obtained parts of ITS1 and ITS2 of MW538530 were identical to MW482435 from Arresø, including two heterozygote bases in ITS1. In the case of MW482435 (recovered from Arresø), we obtained parts of 18s and 28S; these rRNAs were identical to the 18S and 28S parts of FJ469784, FJ469785 and FJ469791 from GenBank. Therefore, based on the molecular analysis of this study, the three sequences FJ469784, FJ469785 and FJ469791 previously deposited at GenBank having the designation Trichobilharzia sp. in GenBank may be designated T. anseri.
Abiotic parameters
We found a clear association between lake temperature and occurrence of infected snails. During the first months of the year the water temperature was low, and no shedding occurred from sampled snails. When temperature increased in spring the infection prevalence increased and peaked in high and late summer (August) (fig. 2). However, other abiotic parameters were not clearly associated with infection. Table 4 lists the physicochemical parameters measured from May to September, including pH, alkalinity, nitrogen, phosphorus, chlorophyll and conductivity from 18 different freshwater lakes from where snails were collected and from which data were available online (ten lakes from Zealand, five from Jutland, two from Funen and one from Lolland). We found no significant correlation between physicochemical parameters and the prevalence of infection in snails or number of cercarial dermatitis cases reported (table 5). The range of pH was from 5.8 in Kvie Sø to 10.5 in Sortedam Sø. The range of alkalinity was from 50 μM in Kvie Sø to 4.4 mM in Haraldsted Sø. The range of nitrogen measure recorded was from 0.49 mg/l in Esrum Sø to 4.8 mg/l in Tissø. The range of phosphorus was from 0.01 mg/l in Tissø to 0.36 mg/l in Søndersø Jutland. The range of chlorophyll was from 1.6 μg/l in Tange Sø to 210 μg/l in Slivsø. The range of the conductivity measurements was from 10 mS/m in Kvie Sø to 90 mS/m in Sortedam Sø.

Fig. 2. Water temperature recorded during the period of investigation (August 2018–September 2020). Months with positive and negative snails are indicated (dark and grey, respectively), together with months where snails could not be recovered (white).
Table 4. Abiotic parameters recorded during summertime (May–September 2018–2020) in 18 lakes, with records of previous and current cercarial dermatitis clinical cases.

The first nine lakes listed contained Trichobilharzia spp.-positive snails revealed by cercarial shedding, and the subsequent nine lakes did not contain snails with Trichobilharzia spp.-positive snails.
a Larsen et al. (Reference Kumar, Stecher, Li, Knyaz and Tamura2004); bChristiansen et al. (Reference Christiansen, Olsen, Buchmann, Kania, Nejsum and Vennervald2016); cunpublished data (Jørgensen & Buchmann). GSD, geometric standard deviation; SD, standard deviation.
Table 5. Correlation between physicochemical parameters and occurrence of parasite infection (snails and human cases). r and P indicate Spearman correlation factors and P-values obtained, respectively.

Probability level applied: P < 0.01.
Biotic parameters in lakes
During snail collection, the occurrence of waterfowl (mallards and swans) was recorded in all of the freshwater habitats visited – both infected and non-infected. The only biotic parameter associated with infection in lakes was the presence of intermediate hosts (snails) and final hosts (waterfowl).
Discussion
During the warm summer of 2018, Danish dermatologists reported an unusually high number of clinical cases of cercarial dermatitis (swimmer's itch) (Tracz et al., Reference Thors and Linder2019). Children and adults became infected due to contact with water in several freshwater lakes. Although the disease is considered benign and the invading cercariae are supposed to die in the skin of the patient, it cannot be ruled out that some cercariae of at least two species may migrate further in a mammalian host (Horák & Kolářová, Reference Horák and Kolářová2000; Horák et al., Reference Horák, Dvořák, Koiářová and Trefil2009). We have, therefore, performed an extensive survey of the occurrence of different species of Trichobilharzia in freshwater lakes. The low prevalence corresponds well with several extensive studies from other freshwater bodies in Europe (Kolářová et al., Reference Kock1992; Loy & Haas, Reference Larsen, Bresciani and Buchmann2001; Ferte et al., Reference Ferte, Depaquit, Carre, Villena and Leger2005; Skírnisson et al., Reference Skírnisson and Kolářová2009) and the infection level previously found in Danish lakes (Larsen et al., Reference Kumar, Stecher, Li, Knyaz and Tamura2004). However, since the snails were sampled in different seasons (from spring until autumn) (fig. 2), and infection is highly temperature-dependent, precaution is required with respect to interpretation of data as the infection level may vary considerably over a season (Loy & Haas, Reference Larsen, Bresciani and Buchmann2001; Zbikowska & Nowak, Reference Zbikowska and Marszewska2009; Brown et al., Reference Brown, Soldánová, Barrett and Kostadinova2011; Al-Jubury et al., Reference Al-Jubury, Kania, Bygum and Buchmann2020). The species identified were T. franki, T. szidati, T. regenti and T. anseri. The first three species have been previously reported from several countries in Europe, including Denmark (Horák et al., Reference Horák, Dvořák, Koiářová and Trefil2009, Reference Horák, Mikeš, Lichtenbergová, Skála, Soldánová and Brant2015; Cichy et al., Reference Cichy, Żbikowska and Faltýnková2011; Soldánová et al., Reference Skírnisson, Aldhoun and Kolářová2013; Christiansen et al., Reference Christiansen, Olsen, Buchmann, Kania, Nejsum and Vennervald2016; De Liberato et al., Reference De Liberato, Berrilli, Bossu, Magliano, Montalbano Di Filippo, Di Cave, Sigismondi, Cannavacciuolo and Scaramozzino2019). However, this is the first report on the identification of T. anseri from Danish lakes, which links this work to a few previous findings in Europe (Jouet et al., Reference Jouet, Skírnisson, Kolářová and Ferté2015).
We showed that three schistosome species may use the same snail host, R. balthica, corresponding to previous reports showing this species as the most important snail host in the life cycle of avian schistosomes (Jouet et al., Reference Hunter2008, Reference Jouet, Ferte, Depaquit, Rudolfova, Latour, Zanella, Kaltenbach and Leger2010, Reference Jouet, Skírnisson, Kolářová and Ferté2015; Skírnisson & Kolářová, Reference Sengupta, Hellstrom and Kariuki2008). In the present work, T. szidati appeared only in L. stagnalis, supporting previous Danish reports (Al-Jubury et al., Reference Al-Jubury, Kania, Bygum and Buchmann2020). However, T. szidati and T. regenti may infect and, under certain circumstances, establish in other related snail species, at least under experimental settings (Kock, Reference Jouet, Kolářová, Patrelle, Ferté and Skírnisson2001; Korsunenko et al., Reference Kolárová, Horák, Skírnisson, Marečková and Doenhoff2010; Hunova et al., Reference Hunova, Kasny, Hampl, Leontovyc, Kubena, Mikes and Horak2012). This suggests that expanded field investigations may be able to expand the list of intermediate hosts for this species. Further systematic analyses of the snail genus Radix, including R. balthica, would also enlighten the problem of some cryptic species possibly covered under the same name. A molecular approach would be suitable in this regard.
It is noteworthy that several cercarial dermatitis cases have been reported from the lake Esrum Sø (Tracz et al., Reference Thors and Linder2019). Although in this particular lake we sampled the highest number of snails from one location, no Trichobilharzia-positive snails were detected. This may be explained by the fact that Esrum Sø is the second largest lake in Denmark, whereby the precise habitat with infected snails may be difficult to locate. It may also illustrate that snail sampling is not always sufficient for mapping the occurrence of the parasite.
We selected a number of freshwater lakes for our investigations, seeking to cover the main areas of Denmark (Zealand, Lolland, Funen and Jutland). Trichobilharzia regenti was distributed only in Zealand, while T. szidati, T. franki and T. anseri were noted in lakes also from Jutland and Zealand. However, as mentioned above, this does not exclude that all species may occur nationwide. In some cases, only a low number of snails were collected at some locations (Almind Sø, Julsø, Vedsted Sø, Svogerslev Sø and Gyrsting Sø) due to limited access to private property, physical inaccessibility, elevated water levels and inferior weather conditions (Duan et al., Reference Duan, Al-Jubury, Kania and Buchmann2021).
In Europe, a number of waterbird species, including mallard and mute swan, serve as definitive hosts and have been reported to host both visceral and nasal species of Trichobilharzia (Horák et al., Reference Horák, Kolářová and Adema2002; Rudolfová et al., Reference Pietrock and Marcogliese2002; Skírnisson & Kolářová, Reference Sengupta, Hellstrom and Kariuki2008; Soldánová et al., Reference Skírnisson, Aldhoun and Kolářová2013). This study identified two final hosts of bird schistosomes. We observed, by light microscopic examination, crescentic eggs in three A. platyrhynchos (two in stool and one in nasal mucosa), giving a prevalence of 33%. This finding corresponds to previous records in wild freshwater birds from Poland and Czechia, with prevalences of 29% and 23%, respectively (Rudolfová et al., Reference Rudolfová, Sitko and Horák2007). In addition, Skírnisson & Kolářová (Reference Sengupta, Hellstrom and Kariuki2008) reported 35.5% prevalence of avian schistosomes in anseriform birds in Iceland. Two schistosome species were found in birds in our study. Their identification was based on sequencing of the ITS region, which showed that eggs recovered from the stool were T. szidati, while the parasite from the nasal region was T. regenti. This connects the final hosts for T. regenti and T. szidati to infections of snails in the Danish freshwater bodies.
The presence of intermediate snail hosts and final bird hosts is the main biotic factor crucial for the life cycle of the parasites, and in all lakes examined both types of hosts were observed. However, snails and released infective cercariae are directly exposed to and may be affected by environmental biotic and other abiotic factors (Pietrock & Marcogliese, Reference Okland1992; Al-Jubury et al., Reference Al-Jubury, Kania, Bygum and Buchmann2020). Temperature was clearly associated with infection, as documented previously (Al-Jubury et al., Reference Al-Jubury, Kania, Bygum and Buchmann2020). However, we also included data on other physicochemical parameters and evaluated any connection between the occurrence of infective parasites and pH, alkalinity, nitrogen, phosphorus, chlorophyll and conductivity. A low pH was suggested to affect the presence of host snails (Hunter, Reference Hunter and Wigington1988, Reference Hunter1990; Okland, Reference Ntonifor and Ajayi2007), whereas Cañete et al. (Reference Cañete, Yong, Sánchez, Wong and Gutiérrez2004) claimed that pH is rarely a factor affecting the distributions of aquatic snails. The pH range (6.9–10) presented in this study did not seem to affect the occurrence of snails. The values recorded in this study correspond to values presented by other reports (Ntonifor & Ajayi, Reference Njoku-Tony2007; Njoku-Tony, Reference Nei and Kumar2011; Usman et al., Reference Tracz, Al-Jubury, Buchmann and Bygum2017). Another factor analysed for an effect on the development and existence of snail infection was chlorophyll. The mean concentration recorded in the summer period from May to September was between 3.5 and 67 μg/l, which complies with the finding that snails and cases of cercarial dermatitis can occur under 5 μg/l (Van Donk et al., Reference Utzinger, Mayombana, Mez and Tanner1989), but otherwise no clear association was noted. This study showed that snails could live and release cercariae in water lakes with a conductivity of 10–80 mS/m, which supplements the range of 56–344 mS/m reported by Dida et al. (Reference Dida, Gelder and Anyona2014). The contents of nitrogen and phosphorus, in this and other studies, did not impact snail distribution in the water bodies (Krist et al., Reference Korsunenko, Chrisanfova, Ryskov, Movsessian, Vasilyev and Semyenova2014; Hill & Griffiths, Reference Hill and Griffiths2017). We cannot exclude that some species of snails, as well as parasites, have different tolerance levels for abiotic factors, but the physicochemical parameters investigated in this study did not exclude snail and parasite occurrence. The distribution of freshwater snails may be a result of more complex interactions of different factors (Utzinger et al., Reference Usman, Babeker and Malann2017), and with regard to the occurrence of parasites, it was clear that the presence of intermediate snail hosts and the final hosts determine the infection risk. As long as the abiotic conditions allow the presence of snails and birds, there is a risk for bird schistosome infections. With climate change, there is a risk of an increased occurrence of the parasites because of temperature elevation, which leads to increased shedding of cercariae in water bodies (Al-Jubury et al., Reference Al-Jubury, Kania, Bygum and Buchmann2020). In addition, cercariae may be predated upon by aquatic invertebrates such as copepods (Courmes et al., Reference Courmes, Eauran, Benex and Deschiens1964; Holliman & Mecham, Reference Holliman and Mecham1971; Bulaev, Reference Bulaev1982). This was supported by recent studies showing that ambient communities can lower disease risk for hosts, via predation on free-living stages of parasites (predation effect). This suggests that a higher biodiversity may influence the occurrence of infective cercariae in water bodies (Goedknegt et al., Reference Goedknegt, Welsh, Drent and Thieltges2015; Al-Jubury et al., Reference Al-Jubury, Kania, Bygum and Buchmann2020). Future studies should, therefore, focus on the importance of predatory plankton organisms and their influence on infection risk.
In conclusion, recreational freshwater lakes in Denmark are known to have highly favourable natural conditions for avian schistosomes. We documented the occurrence of at least four different avian schistosome species in Danish freshwater lakes. Cercariae belonging to the species T. franki, T. szidati, T. regenti and T. anseri were detected from east to west in Danish lakes commonly used for recreational activities such as bathing, swimming and fishing. The wide distribution is of public health concern due to the skin pathologies that may accompany bathing in infested water. It cannot be excluded that at least some Trichobilharzia species may perform extra-dermal migrations in the human host. Particularly noteworthy in this context is the finding of the neurotropic species, T. regenti. The parasite occurrence was associated with presence of final bird hosts (mallards and swans) and first intermediate lymnaeid host snails, but a nationwide bird survey is recommended to elucidate the role of other avian host species. The measurement of the physicochemical parameters under natural conditions of the Danish freshwater lakes documented an association between temperature and infections, whereas other abiotic parameters were of low or no importance. It was clear that the presence of intermediate snail hosts and the final hosts determines the infection risk. As long as the abiotic conditions allow the presence of snails and birds, there is a risk for bird schistosome infections. The possible neurotropic pathology of T. regenti and other avian schistosomes in humans (Lichtenbergová & Horák, Reference Lichtenbergova and Horak2012) calls for further controlled studies on the fate of cercariae in the human host. Routine surveillance of lakes used for recreational activities should be implemented and sensitive and species-specific molecular methods for detection of infective agents should be developed (Bass et al., Reference Bass, Stentiford, Littlewood and Hartikainen2015; Sengupta et al., Reference Schell1970).
Supplementary material
To view supplementary material for this article, please visit https://doi.org/10.1017/S0022149X21000122
Acknowledgements
The authors would like to thank the staff of the Aquatic Pathobiology Laboratory for their patience and help during the snail investigations. We are grateful to staff members in the Laboratory of Helminthology, Department of Parasitology, Faculty of Science, Charles University, for providing training in bird dissection. The authors would also like to acknowledge Anne Sofie Vedsted Hammer, Section of Pathology at Faculty of Health and Medical Sciences, University of Copenhagen, for her help in delivering dead swans.
Financial support
This study was funded by Odense Universitetshospital and the University of Copenhagen.
Conflicts of interest
None.
Ethical standards
This study did not require approval from authorities, organizations or ethics committees. All animal procedures were performed observing the general ethical guidelines of the University of Copenhagen. All snail samples were euthanized after the shedding procedures.