Assessment of emerging CVD risk factors may improve risk prediction and treatment strategies as many individuals continue to experience residual CVD risk despite meeting current LDL-cholesterol and blood pressure targets. For instance, HDL functionality (i.e. cholesterol efflux) has recently been proposed as a more informative marker of CVD risk than HDL-cholesterol( Reference Khera, Cuchel and de la Llera-Moya 1 , Reference deGoma, deGoma and Rader 2 ) due to the lack of benefit on hard endpoints in trials of pharmacological HDL-cholesterol raising agents( Reference Boden, Probstfield and Anderson 3 – Reference Barter, Caulfield and Eriksson 5 ). Similarly, central blood pressure and related indices of arterial stiffness have been shown to better predict cardiovascular events( Reference Roman, Devereux and Kizer 6 ) and are more sensitive predictors of disease severity( Reference Waddell, Dart and Medley 7 ) compared with brachial pressures. Plant-based whole foods are recommended for reducing CVD risk( 8 ) but it has yet to be conclusively established whether cholesterol efflux and/or macrovascular function can be modified by polyphenol-rich plant-based foods/beverages.
Previous studies have demonstrated promising results for these outcomes with certain interventions (e.g. berries, red wine and cocoa), but evidence is limited. For instance, ex vivo cholesterol efflux has been shown to increase following supplementation with extra virgin olive oil( Reference Helal, Berrougui and Loued 9 ) and coffee( Reference Uto-Kondo, Ayaori and Ogura 10 ), as well as resveratrol( Reference Berrougui, Grenier and Loued 11 ) and anthocyanins( Reference Xia, Hou and Zhu 12 ), which are found in red wine and berries. However, other studies have produced mixed results( Reference Fuhrman, Volkova and Aviram 13 ) and other polyphenol-rich interventions have yet to be investigated. Improvements in central blood pressure and other measures of macrovascular function have also been found following supplementation with polyphenol-rich berries( Reference McAnulty, Collier and Landram 14 , Reference Dohadwala, Holbrook and Hamburg 15 ), grapefruit( Reference Habauzit, Verny and Milenkovic 16 ), watermelon( Reference Figueroa, Wong and Hooshmand 17 , Reference Figueroa, Sanchez-Gonzalez and Perkins-Veazie 18 ) and isoflavone-enriched chocolate( Reference Curtis, Potter and Kroon 19 ), but results have been inconsistent for other interventions( Reference Jovanovski, Bosco and Khan 20 – Reference Lynn, Hamadeh and Leung 23 ). In addition, many of these findings were specific to postmenopausal women( Reference Habauzit, Verny and Milenkovic 16 , Reference Figueroa, Wong and Hooshmand 17 , Reference Curtis, Potter and Kroon 19 ) or normotensive individuals( Reference McAnulty, Collier and Landram 14 , Reference Jovanovski, Bosco and Khan 20 – Reference Lynn, Hamadeh and Leung 23 ).
Soyabeans provide plant protein as well as numerous polyphenols and other bioactive compounds, including isoflavones( Reference Sacks, Lichtenstein and Van Horn 24 , Reference Kris-Etherton, Hecker and Bonanome 25 ). Consuming 25 g/d soya protein (as part of a diet low in saturated fat and cholesterol) may reduce the risk of heart disease by lowering blood cholesterol( 26 ), and epidemiological evidence suggests that greater soya protein intake may be related to reduced CVD risk( Reference Zhang, Shu and Gao 27 , Reference Sasazuki 28 ), particularly in postmenopausal women( Reference Kokubo, Iso and Ishihara 29 ). However, much less is known about the effects of soya on cholesterol efflux and macrovasculature function. Notably, many previous studies have been limited to isolated soya isoflavones, despite pre-clinical evidence that cardiovascular benefits may only be achieved when isoflavones are administered in conjunction with soya protein( Reference Wagner, Schwenke and Greaves 30 – Reference Anthony, Clarkson and Bullock 32 ). With regard to HDL function, to our knowledge no studies have been conducted using a soya protein isolate with naturally occurring isoflavones. Supplementation with isolated soya isoflavones did not elicit any change in ex vivo cholesterol efflux in postmenopausal women following 3 months of supplementation( Reference Badeau, Jauhiainen and Metso 33 , Reference Tormala, Nikander and Tiitinen 34 ). Initial evidence for the effect of soya isoflavones on macrovascular function has been promising( Reference Teede, McGrath and DeSilva 35 , Reference Pase, Grima and Sarris 36 ); however, few studies have investigated soya protein with endogenous isoflavones and results have been inconsistent( Reference Nestel, Fujii and Zhang 37 – Reference Teede, Dalais and Kotsopoulos 39 ). Furthermore, no studies have evaluated potential dose–response effects of an isoflavone-containing soya protein isolate on these risk factors.
The present study was designed to investigate the dose–response effects of a soya protein isolate (delivering 25 and 50 g/d soya protein) containing endogenous isoflavones on HDL function (i.e. ex vivo cholesterol efflux), central blood pressure, indices of arterial stiffness and other blood markers of CVD risk in adults with moderately elevated resting blood pressure. Our primary hypothesis was that supplementation with an isoflavone-containing soya protein isolate would increase ex vivo cholesterol efflux compared with the placebo control. In addition, we hypothesised that soya supplementation would improve vascular function, indices of arterial stiffness, and blood markers of CVD risk in a dose-dependent manner relative to the control.
Methods
Study population
Men and women who were 35–60 years of age, had moderately elevated resting blood pressure (systolic blood pressure≥120 mmHg and/or diastolic blood pressure≥80 mmHg and <160/100 mmHg), and were otherwise free of any serious illness were recruited for the study. Other inclusion criteria consisted of: following a typical American diet (i.e. not vegetarian, not engaged in a structured diet plan or attempting to lose weight, and not frequently consuming soya products), BMI of 18–39 kg/m2, fasting TAG<3·95 mmol/l, and fasting LDL-cholesterol<4·14 mmol/l. Exclusion criteria included: smoking and/or use of other tobacco products; a history of diabetes, autoimmune disorders, and heart, liver, kidney or thyroid disease; pregnancy, lactation or a desire to become pregnant during the study; chronic use of non-steroidal anti-inflammatories or medications/supplements for elevated lipids, blood pressure or glucose; lactose intolerance; and excessive alcohol consumption (>14 standard drinks per week).
Participant recruitment
Participants were recruited from March 2014 to June 2015 via fliers in the community, campus email lists and a university research website. Potential subjects emailed or called to indicate interest in participating in the study and were then given additional information about the study. If interested, they were asked a series of medical history and lifestyle questions to screen for eligibility. A schematic of participant recruitment for the study is provided in Fig. 1. Of the 170 initial respondents who provided contact information, 149 elected to complete the initial screening questions by telephone. In all, forty-eight of these volunteers met study criteria and completed a screening appointment at the Pennsylvania State University Clinical Research Center (CRC) to verify eligibility. After written informed consent was obtained, a urine pregnancy test was performed for women of child-bearing potential, and blood pressure was measured according to JNC 7 guidelines( Reference Chobanian, Bakris and Black 40 ). In brief, after a 5-min seated rest, three readings were taken by nurses in a controlled environment using a calibrated mercury sphygmomanometer. The average of the last two readings was used to determine eligibility. If an individual’s blood pressure met the study inclusion criteria, body weight and height were measured (without shoes and in light clothing) to calculate BMI. A blood sample was then drawn for a complete blood count and standard chemistry profile (lipid panel, glucose, liver and kidney function) to rule out the presence of illness (autoimmune disease, cancer and immunodeficiency). Of the forty-eight individuals who were screened, twenty-three met eligibility criteria and were enrolled in the study. Three participants withdrew during the study. Thus, data are reported for twenty participants (nine men, eleven women). The screening characteristics of the twenty participants who completed the study are available in the online Supplementary Table S1.

Fig. 1 Flow diagram of participant recruitment.
A balanced randomisation scheme with a block size of six was developed in advance (by A. C. S.-R.) using an online randomisation generator, and subjects were assigned to a treatment sequence at enrolment (by C. J. L.). Sample size was determined based on a power calculation, with ex vivo cholesterol efflux as the primary outcome. In all, twenty participants were estimated to provide 80 % power to detect a 2 percentage point change in cholesterol efflux with a significance level of 0·05, based on the variability of cholesterol efflux values in our previous work( Reference Berryman, Grieger and West 41 ). This study was conducted according to the guidelines laid down in the Declaration of Helsinki and all procedures involving human subjects were approved by the Institutional Review Board of the Pennsylvania State University. Written informed consent was obtained from all participants. The study was registered at clinicaltrials.gov as NCT02180841.
Study design and intervention
This was a randomised, placebo-controlled, three-period cross-over study with 6-week treatment periods separated by an approximate 2-week compliance break. Treatment periods were extended by up to 2 weeks (8 weeks total) in the case of illness, injury or scheduling difficulties. During the treatment periods, participants received 50, 25 or 0 g/d (control) soya protein delivered as an unfractionated soya protein isolate (isolate contained 87·4 % protein by total weight) that contained naturally occurring isoflavones, in random order. Treatments consisted of dry blended beverage powders provided as two identical sachets per day during all three periods. Treatments were matched to coded alphanumeric identifiers so that the researchers and participants were blinded to treatment assignment. The soya and control powders were formulated to be matched for carbohydrate and mineral (Na+, K+, Ca2+ and Mg2+) content, and differed mainly in the amount of soya protein provided (Table 1). The control beverage powders contained maltodextrin in place of the protein, and also contained approximately 1 % (w/w) total hydrocolloids (xanthan and carageenan; DuPont™ Danisco® Grinsted®) to provide a similar mouthfeel as the protein-containing beverages and maintain blinding. The soya protein isolate was prepared from defatted soyabean flakes by standard isoelectric precipitation, followed by partial protein hydrolysis with food enzymes and agglomeration for quicker dispersion into liquid. The soya protein isolate was analysed for isoflavone content by Nestlé Purina Analytical Laboratories and was determined to contain 1·7 mg of isoflavones (expressed as aglycone units) per g of protein, consisting of genistein, daidzein and glycitein (0·55:1·09:0·06 mg/g protein, respectively). All treatment powders were provided in individual sachets that were identical in appearance. Powders were also matched for taste and appearance to maintain the blinding of participants and researchers to treatment sequence. All study powders were formulated and provided by DuPont Nutrition and Health and analysed by Nestlé Purina Analytical Laboratories.
Table 1 Nutrient profile of the control and isoflavone-containing soya protein supplementsFootnote *
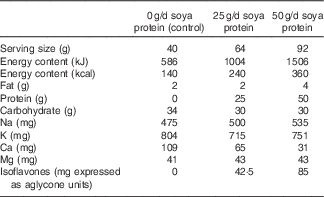
* Values are based on analyses conducted on the final products by Nestlé Purina Analytical Laboratories and represent the averages of the vanilla and chocolate flavours that were provided for each treatment period.
Participants were instructed to consume two sachets per day during each treatment period by mixing the study powders with milk, water or food. They were provided with 1 week’s worth of treatment powders at a time. Participants were asked to incorporate the study powders into their habitual diet and maintain their normal dietary intake and physical activity. In addition, participants were instructed to avoid all other soya products for the duration of the study. A 3-d food record (2 weekdays and 1 weekend day) was completed at baseline and during the final week of each treatment period to confirm that dietary habits remained stable throughout the study and that soya-containing foods were avoided. Participants reported to the Metabolic Diet Center on a weekly basis. At this time, a member of the research team who was not involved in the statistical analyses provided participants with additional study powder and reviewed their body weight and treatment powder consumption logs.
Blood sampling and vascular testing was performed at baseline and following each 6-week supplementation period. All study procedures were conducted at the Pennsylvania State University CRC according to standardised protocols. During the 48 h before testing visits, participants were instructed to: avoid high antioxidant foods (berries, cocoa/chocolate), strenuous exercise and alcohol; refrain from taking pain relievers, vitamins or minerals; and limit their intake of coffee and tea to no more than one cup per day. Testing visits were conducted following an overnight fast (no food or drink other than water for 12 h). At each visit, vascular function testing was performed before blood sampling. All study procedures were completed by December 2015.
Vascular function measures
Vascular function, in terms of central blood pressure and arterial stiffness indices, was assessed using the SphygmoCor System pulse waveform analysis (AtCor Medical). All measurements were performed in a temperature-controlled, quiet, dimly lit room.
Pulse wave analysis: central (aortic) blood pressure and augmentation index
Following a 5 min seated rest, central pressures and wave reflection characteristics (i.e. augmentation pressure and the augmentation index (AI)) were derived from brachial pressure waveforms using a generalised transfer function that is considered by the US Food and Drug Administration to be substantially equivalent to generalised transfer functions for radial tonometry that have been validated against an indwelling catheter( Reference Chen, Nevo and Fetics 42 – Reference Sharman, Lim and Qasem 44 ). At each visit, three pulse wave analysis (PWA) measurements were taken, following JNC 7 blood pressure guidelines( Reference Chobanian, Bakris and Black 40 ), with 1 min between each reading. The last two PWA results were averaged and used for analysis. The AI was standardised to a heart rate of 75 beats per min (AI@75) to correct for the independent inverse effect of heart rate on augmentation of the pulse wave form( Reference Wilkinson, MacCallum and Flint 45 ).
Pulse wave velocity
Aortic stiffness was assessed by carotid-femoral pulse wave velocity (PWV). Carotid and femoral arterial pressure waveforms were measured simultaneously via an applanation tonometry sensor manually held in place above the right common carotid artery and a blood pressure cuff placed on the right femoral artery. Distance measurements were taken from the sternal notch to the carotid artery, from the sternal notch to the top of the femoral cuff, and from the femoral artery to the top of the femoral cuff. Based on these measurements, the SphygmoCor System automatically calculates the distance travelled by the pulse wave from the carotid artery to the femoral artery. Transit time between the carotid and femoral pressure waves is determined by the SphygmoCor System using the foot-to-foot method( Reference Laurent, Cockcroft and Van Bortel 46 ). PWV is then calculated as distance over transit time. At each visit, three PWV measurements were obtained in the supine position, with 1 min between readings. The last two PWV results were averaged for analysis.
Blood sample collection and assay methods
Blood drawn into anticoagulant-coated tubes containing lithium heparin or EDTA was immediately centrifuged for 15 min at 1500 g . Blood drawn into serum separator tubes was allowed to clot for 30 min before centrifugation. Total cholesterol and TAG were measured by enzymatic procedures (Quest Diagnostics; CV<2 % for both). HDL-cholesterol was estimated according to the modified heparin-manganese procedure (Quest Diagnostics; CV<2 %). LDL-cholesterol was calculated using the Friedewald equation (LDL-cholesterol=total cholesterol−(HDL-cholesterol+TAG/5)). Glucose was determined by Spectrophotometry procedures (Quest Diagnostics). Insulin was measured by RIA using 125I-labelled human insulin and a human insulin antiserum (Quest Diagnostics). Serum high-sensitivity C-reactive protein (CRP) was measured by latex-enhanced immunonephelometry (Quest Diagnostics; assay CV<8 %). For other endpoints, aliquots of serum and plasma were immediately stored at −80°C for batch analysis.
Cholesterol efflux
Cholesterol efflux capacity was quantified in serum samples using a validated ex vivo assay (Vascular Strategies LLC). J774 mouse macrophage cells were grown and maintained in Roswell Park Memorial Institute (RPMI) 1640 medium containing 10 % fetal bovine serum (FBS) and 0·5 % gentamycin and incubated at 37°C in a humidified incubator (95 % air and 5 % CO2). Cells were then plated in growth medium in 24-well tissue culture plates at a density of 150 000 cells/well for 24 h and grown to 80–90 % confluence. Subsequently, cells were washed three times with HEPES-buffered minimum essential medium (MEM) and labelled with 2 µCi/ml [1,2-3H]cholesterol for 24 h in RPMI medium containing 5 % FBS. After labelling, cells were washed with MEM–HEPES twice and RPMI 1640 medium containing 0·2 % bovine serum albumin plus 0·3 mm-8-(4-cholorophenyl-thio)-cyclic AMP was added to the cells for 16 h to up-regulate ATP-binding cassette transporter 1 (ABCA1). After ABCA1 up-regulation, cells were washed with MEM–HEPES, and efflux of [3H]free cholesterol (FC) was initiated by incubating the cells for 4 h with 2·5 % apoB-depleted serum from the study subjects. After the media was collected and filtered, 0·2 ml was used to calculate efflux by measuring the release of [3H]FC into the medium. Experiments were performed with the presence of acetyl-CoA acetyltransferase (ACAT) inhibitor, Sandoz 58-035 to ensure that essentially all of the labelled cholesterol and cholesterol mass was present as FC. Cholesterol efflux was quantified as the percentage of radiolabel in the media compared with that present in the cells before incubation with cholesterol acceptors. The amount of radiolabel present in the cells was determined by extracting cell lipids with isopropanol and measuring the [3H] cholesterol in the lipid extraction by liquid scintillation counting. Samples were analysed in triplicate, with a mean CV<5 %.
Statistical analyses
All statistical analyses were performed using SAS (version 9.4; SAS Institute). Only participants who completed all three supplementation periods were included in analyses. Differences between male and female participants at baseline were assessed by an independent two-sample t test (PROC TTEST). Change scores for end of treatment values were calculated by subtracting study-entry baseline values from each post-supplementation measure. Outcome variables were checked for normality (PROC UNIVARIATE) and positively skewed variables (skew >1; TAG, CRP and insulin) were logarithmically transformed. Participants with acutely elevated CRP (i.e. CRP≥10·5 mg/l) at the end of a treatment period (n 2 for 50 g/d treatment period), which is indicative of acute inflammation, were excluded from all analyses for that treatment period. This was done to ensure that the analysis was performed on the target population of healthy adults with moderately elevated blood pressure. For end of treatment values, the mixed models procedure (PROC MIXED) in SAS was used to test the effects of treatment, period and treatment by period interactions for each outcome. Baseline values were included as covariates. Because there was a trend for a significant period effect for the change in weight over the course of the study (P=0·07; −0·2, +0·3 and +0·9 kg for periods 1, 2 and 3, respectively), change in weight was included as a covariate in all models. Subject was treated as a random effect and the remaining factors were fixed effects. When period and treatment by period interactions were non-significant, they were removed from the model. For all outcomes, no treatment by period effect was found. When period effects were significant, they were retained in the final model of treatment effects. Tukey–Kramer-adjusted P values were used for post hoc comparisons between the three groups. Values that were measured in duplicate on separate days (i.e. body weight and lipids) were averaged before analysis. Means are reported as least squares means with their standard errors unless otherwise specified. For all tests, α was set at 0·05.
Results
Of the twenty participants that completed the study, all were middle-aged, seven were normal weight (BMI 18·5 to <25 kg/m2), nine were overweight (BMI >25 and <30 kg/m2), and four were obese (BMI>30 kg/m2) at baseline (Table 2). All had moderately elevated resting systolic and diastolic blood pressure (Table 2). At baseline, LDL-cholesterol was optimal (<2·6 mmol/l) in two subjects, above optimal (2·6–3·3 mmol/l) in fourteen subjects, and borderline high (3·4–4·1 mmol/l) in four subjects. At baseline, male participants had significantly lower total cholesterol (TC), HDL-cholesterol, LDL-cholesterol, non-HDL-cholesterol, augmentation pressure, AI and heart rate compared with female participants (Table 2); otherwise, male and female participants who completed the study were not different from one another at baseline. No sex differences were found for any outcome variables in exploratory subgroup analyses (data not shown). Participants were compliant with the study intervention based on completion of self-reported daily consumption logs.
Table 2 Baseline characteristics of participants who completed the study (Mean values with their standard errors, and ranges; n 20)

TC, total cholesterol; CRP, C-reactive protein; SBP, systolic blood pressure; DBP, diastolic blood pressure; PP, pulse pressure; AP, augmentation pressure; AI@75, augmentation index normalised to 75 beats per min; HR, heart rate; PWV, pulse wave velocity.
* Significant difference between male and female participants (P<0·05).
Effect of soya supplementation on ex vivo cholesterol efflux
There were no significant treatment effects for any measures of ex vivo cholesterol efflux (Table 3). ABCA1-specific efflux was significantly reduced from baseline following supplementation with the control (−12·7 %; P=0·02), but this change was not significant compared with ABCA1 efflux following 50 g/d of soya protein (P=0·4).
Table 3 Measures of ex vivo cholesterol efflux following supplementation with 50 g/d isoflavone-containing soya protein compared with the controlFootnote † (Mean values with their standard errors; n 20)

ABCA1, ATP-binding cassette transporter 1; cAMP, cyclic AMP; CRP, C-reactive protein.
Significant change from baseline: * P=0·02.
† ABCA1-specific efflux was calculated as the difference between cAMP-stimulated and non-stimulated (−cAMP) efflux. Values were compared using the MIXED procedure (SAS version 9.4; SAS Institute Inc.). Period effects were universally non-significant (P>0·05).
‡ Data represent eighteen participants; two were excluded from analyses for this treatment period due to acutely elevated CRP concentrations (i.e. CRP≥10·5 mg/l).
Effect of soya supplementation on vascular function
Post-supplementation values for blood pressure (brachial and central), AI and PWV are presented in Table 4. There was a significant treatment effect for brachial diastolic blood pressure (P=0·04); however, in post hoc comparisons, the 2·3 mmHg reduction in brachial diastolic blood pressure following supplementation with 50 g/d soya protein was only significant compared with 25 g/d supplementation (Tukey-adjusted P=0·03) (Fig. 2). There was a trend towards a significant treatment effect for central systolic blood pressure (P=0·12); however, the only post hoc comparison that neared significance was 50 g/d soya protein v. 25 g/d (1·6 % decrease v. 0·8 % increase, respectively; unadjusted P=0·05). There were no significant treatment effects for any other outcomes. There was a trend for a 3·1 and 2·8 point increase in AI@75 from baseline following supplementation with 50 g/d soya protein and 0 g/d (P=0·07 and P=0·09, respectively), but these changes were not significantly different from one another.

Fig. 2 Changes in brachial and central blood pressures from baseline following 6 weeks of control and soya protein supplementation. Values are means (n 20), with their standard errors represented by vertical bars. SBP systolic blood pressure; DBP, diastolic blood pressure; , 0 g/d;
, 25 g/d;
, 50 g/d. Change scores were calculated by subtracting the baseline pre-supplementation value from post-supplementation values, and were compared using the MIXED procedure (SAS version 9.4; SAS Institute Inc.). a,b Mean values with unlike letters were significantly different. * Trend towards a significant difference from baseline (P=0·07).
Table 4 Measures of vascular function and indices of arterial stiffness following each 6-week treatment periodFootnote * (Mean values with their standard errors; n 20)
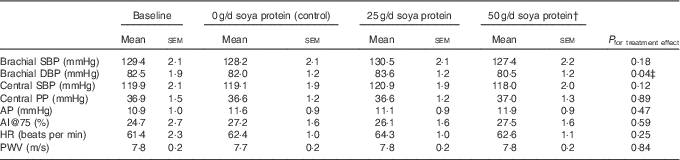
SBP, systolic blood pressure; DBP, diastolic blood pressure; PP, pulse pressure; AP, augmentation pressure; AI@75, augmentation index normalised to 75 beats per min; HR, heart rate; PWV, pulse wave velocity; CRP, C-reactive protein.
* Values were compared using the MIXED procedure (SAS version 9.4; SAS Institute Inc.). Period effects were universally non-significant (P>0·05).
† Data represent eighteen participants; two were excluded from analyses for this treatment period due to acutely elevated CRP concentrations (i.e. CRP≥10·5 mg/l).
‡ Only 25 g/d soya protein v. 50 g/d was significant in post hoc comparisons (Tukey-adjusted P=0·03).
Effect of soya supplementation on lipids/lipoproteins and other blood markers of CVD
Post-supplementation values for lipids/lipoproteins, glucose metabolism and inflammatory markers are presented in Table 5. There were no significant treatment effects for any outcomes. Significant period effects were found for TAG, non-HDL-cholesterol and CRP. In post hoc comparisons, period 1 values were significantly lower than period 3 values for TAG (geometric means: 1·09 mmol/l (95 % CI 0·89, 1·320) v. 1·32 mmol/l (95 % CI 1·11, 1·57)) and non-HDL-cholesterol (means: 3·40 (sem 0·09) v. 3·68 (sem 0·09) mmol/l), whereas period 1 CRP values (geometric mean: 1·8 mg/l (95 % CI 1·1–2·9)) were significantly higher than period 2 (geometric mean: 1·2 mg/l (95 % CI 0·7–2·0)) and period 3 values (geometric means: 1·2 mg/l (95 % CI 0·7–2·1)). Significant changes from baseline were found following soya supplementation for glucose and total cholesterol but no post hoc comparisons between treatment groups were significant.
Table 5 Blood markers of CVD risk following each 6-week treatment periodFootnote * (Mean values with their standard errors; n 20)

TC, total cholesterol; CRP, C-reactive protein.
a Period 1 values significantly lower than period 3 values in post hoc comparisons (Tukey-adjusted P=0·002); b period 1 values significantly lower than period 3 values in post hoc comparisons (Tukey-adjusted P=0·02); c period 1 values significantly higher than period 2 and period 3 values in post hoc comparisons (Tukey-adjusted P=0·03 and 0·05, respectively).
* Values were compared using the MIXED procedure (SAS version 9·4; SAS Institute Inc.).
† Data represent eighteen participants; two were excluded from analyses for this treatment period due to acutely elevated CRP concentrations (i.e. CRP≥10·5 mg/l).
Discussion
This study investigated the effects of a soya protein isolate containing endogenous isoflavones on HDL function and vascular function in adults with moderately elevated resting blood pressure. We found no significant treatment effects of soya supplementation for ex vivo cholesterol efflux or other blood markers of CVD risk. Brachial diastolic blood pressure was significantly reduced following consumption of 50 g/d of soya protein, but this 2·3 mmHg decrease was significant only when compared with the non-significant increase following supplementation with 25 g/d soya protein. No other measures of vascular function were significantly altered by the study treatments.
Previous studies have shown that HDL function, in terms of ex vivo cholesterol efflux, can be improved by polyphenol-rich interventions such as extra virgin olive oil( Reference Helal, Berrougui and Loued 9 ) and coffee( Reference Uto-Kondo, Ayaori and Ogura 10 ), but little is known about soya. To our knowledge, the effect of an isoflavone-containing soya protein isolate on HDL function – rather than isolated soya isoflavones – has not previously been studied. The two previous studies of isolated soya isoflavones found that 3 months of supplementation had no effect on ex vivo cholesterol efflux in postmenopausal women( Reference Badeau, Jauhiainen and Metso 33 , Reference Tormala, Nikander and Tiitinen 34 ). Pre-clinical evidence suggests that the provision of isoflavones in combination with soya protein may be necessary to elicit significant changes( Reference Wagner, Schwenke and Greaves 30 – Reference Anthony, Clarkson and Bullock 32 ). The lack of effect on HDL efflux in the current study, using a soya protein isolate with isoflavones, may be due to the relatively short plasma half-life of soya isoflavones( Reference Hazim, Curtis and Schar 47 – Reference Zubik and Meydani 49 ), as soya isoflavones may not have been at peak concentrations during the time of the fasting blood sample. Alternatively, a longer supplementation period (e.g. 3 months) may be needed for other components of soya (i.e. soya protein) to have a sustained effect on ex vivo cholesterol efflux. Further research is needed to determine whether soya supplementation, either acute or longer-term, has the potential to improve HDL function. In addition, other tests of HDL function are also available and may provide further insight as a single assay may not be an ideal surrogate measure of HDL function.
Polyphenol-rich interventions have also been shown to improve central blood pressure and other measures of macrovascular function, but results have been inconsistent. For instance, significant benefits have been achieved with watermelon( Reference Figueroa, Wong and Hooshmand 17 , Reference Figueroa, Sanchez-Gonzalez and Perkins-Veazie 18 ), cranberry juice( Reference Dohadwala, Holbrook and Hamburg 15 ) and isoflavones( Reference Curtis, Potter and Kroon 19 , Reference Teede, McGrath and DeSilva 35 ), whereas no changes were found with spinach( Reference Jovanovski, Bosco and Khan 20 ), cherry juice( Reference Lynn, Mathew and Moore 21 ) or blueberries( Reference Johnson, Figueroa and Navaei 50 ). These disparate results may be due in part to differences in study design (e.g. duration of supplementation) or the relative health of participants (e.g. individuals with elevated blood pressure v. normotensive individuals), but are also likely dependent on the unique profile of bioactive compounds provided by each intervention. Current understanding of the effects of soya on macrovascular function are similarly inconsistent and limited to a relatively small number of studies( Reference Nestel, Fujii and Zhang 37 – Reference Teede, Dalais and Kotsopoulos 39 , Reference Hazim, Curtis and Schar 47 , Reference Tormala, Appt and Clarkson 51 ). Although significant reductions in PWV have been found using a synthetic isoflavone metabolite( Reference Nestel, Fujii and Zhang 37 ) and isolated red clover isoflavones( Reference Teede, McGrath and DeSilva 35 ), no significant changes in central PWV or AI were found following supplementation with a soya cereal (40 g soya protein)( Reference Teede, Giannopoulos and Dalais 38 ) or soya protein isolates (40 and 52 g soya protein)( Reference Teede, Dalais and Kotsopoulos 39 , Reference Tormala, Appt and Clarkson 51 ). Thus, our results are consistent with previous findings of no effect on PWV and/or AI. The cause of this incongruity between the vascular effects of isolated isoflavones v. soya protein is unknown as the studies were conducted in similar populations and the interventions provided similar amounts of isoflavones( Reference Teede, McGrath and DeSilva 35 , Reference Nestel, Fujii and Zhang 37 – Reference Teede, Dalais and Kotsopoulos 39 , Reference Tormala, Appt and Clarkson 51 ); however, this may be related to differences in the bioavailability of isoflavones from different food sources( Reference Cassidy, Brown and Hawdon 52 ).
LDL-cholesterol and blood pressure are the primary modifiable CVD risk factors, and the potential hypocholesterolaemic effects of soya have been extensively investigated. Based largely on a 1995 meta-analysis( Reference Anderson, Johnstone and Cook-Newell 53 ) in which soya protein consumption (47 g/d) reduced LDL-cholesterol by 13 %, the US Food and Drug Administration issued a health claim stating that 25 g/d of soya protein (as part of a diet low in saturated fat and cholesterol) may reduce the risk of heart disease( 26 ). However, more recent studies of soya protein have found much smaller LDL-cholesterol reductions (3–6 %)( Reference Sacks, Lichtenstein and Van Horn 24 , Reference Zhan and Ho 54 – Reference Harland and Haffner 56 ). LDL-cholesterol reduction may also be greater in individuals with hypercholesterolaemia and/or elevated LDL-cholesterol at baseline( Reference Anderson, Johnstone and Cook-Newell 53 , Reference Zhan and Ho 54 , Reference Tokede, Onabanjo and Yansane 57 ); thus, the lack of LDL-cholesterol reduction in our study may have been due to the relatively normal TC (mean TC=5·12 mmol/l) and LDL-cholesterol (mean LDL-cholesterol=3·10 mmol/l) of our participants at baseline, and is not unprecedented. Studies investigating the effects of soya on brachial blood pressure are more limited, but evidence suggests that soya supplementation may improve systolic and diastolic blood pressure; however the magnitude of effects may depend on the baseline blood pressure of participants and the type of control( Reference Dong, Tong and Wu 58 ). The 2·3 mmHg reduction in diastolic blood pressure that we observed with the 50 g/d dose is consistent with previous findings for individuals with moderately elevated resting blood pressure; however, it should be noted that this change was only significant compared with the change following the 25 g/d dose.
In addition to the duration of supplementation, our null results may also be explained by the capacity of our participants to produce equol. In approximately 25–30 % of individuals in Western countries, the parent isoflavone compound daidzein undergoes intestinal bacterial metabolism to form equol( Reference Setchell and Clerici 59 ). Because equol and other isoflavone metabolites have more potent oestrogenic and antioxidant properties, it has been suggested that the beneficial effects of soya are dependent upon this conversion, and thus may occur only in equol producers( Reference Setchell, Brown and Lydeking-Olsen 60 ). For instance, in older men with moderate 10-year CVD risk, only equol producers experienced acute improvements in arterial stiffness following the consumption of isolated isoflavones( Reference Hazim, Curtis and Schar 47 ). However, the role of equol in eliciting the cardiovascular benefits of soya remains unclear since not all studies have shown differential effects in equol producers and non-equol producers( Reference Badeau, Jauhiainen and Metso 33 , Reference Tormala, Appt and Clarkson 51 , Reference Kreijkamp-Kaspers, Kok and Bots 61 ). In addition, in most cases these were exploratory analyses and the studies were not designed to analyse differences between equol producers and non-equol producers. We did not assess equol production in our participants. However, given the prevalence of equol producers in the general US population, it is likely that many of our participants were non-equol producers and this may have influenced our results. Future soya protein supplementation studies should prospectively examine potential differences in effects between equol producers and non-equol producers to clarify the role of equol in achieving any physiological changes.
We further speculate that any beneficial effects of soya may depend on additional concomitant dietary changes. In the current study, the soya intervention, which provided both protein and isoflavones, was incorporated into the background diet without otherwise altering dietary habits. Participants significantly increased their protein intake during the 25 and 50 g/d soya protein supplementation periods (+17 and +44 g/d, respectively; P<0·0001), but the intake of energy content and other macronutrients was unchanged throughout the study and soya protein supplementation did not result in significant weight gain. In many of the studies demonstrating the LDL-lowering ability of soya, soya protein was compared with casein, milk or mixed animal proteins( Reference Sacks, Lichtenstein and Van Horn 24 ), whereas a carbohydrate-matched control was used in the current study. We noted that the carbohydrate control led to similar non-significant reductions in total and LDL-cholesterol compared with the soya protein treatments. It is possible that the cholesterol lowering effect from the control was due to a substitution effect of reducing fat energy content with energy content from carbohydrates, and/or the prebiotic effect of maltodextrin with the added hydrocolloids. Therefore, improvements in cholesterol efflux and macrovascular function following consumption of a soya protein isolate may similarly depend on the type of control and/or whether it replaces another component of the diet (e.g. animal protein, refined carbohydrates, added sugars, etc.). In addition, it is possible that the processing techniques used to produce the soya protein isolate and make it more dispersible in beverages (agglomeration) may have altered essential attributes of the protein or isoflavones that are necessary for their physiological actions. Future studies are needed to investigate whether processing techniques can alter the physiological effects of soya protein isolates. If future research confirms that soya products effectively modify CVD risk factors, incorporating them into an overall healthy dietary pattern is likely to achieve the greatest benefit.
Strengths and limitations
The cross-over design of this study allowed participants to act as their own controls and decreased potential sources of error by minimising the influence of between-subject variability when analysing treatment effects. Our participants had moderately elevated resting blood pressure but were otherwise generally healthy and representative of the general population. Our study population was also well-balanced with regard to male and female participants; however, our sample size was relatively small and additional studies with larger participant populations are needed. Moreover, we did not determine the equol producing capability of our participants, which may influence whether soya supplementation elicits significant health benefits( Reference Setchell and Clerici 59 ). The soya protein isolate intervention was incorporated into the normal dietary habits of participants, making it more reflective of ‘real world’ implementation. The carbohydrate content of the soya and control powders was closely matched, and the amount of soya protein and isoflavones provided by the interventions was consistent previous studies( Reference Badeau, Jauhiainen and Metso 33 , Reference Tormala, Nikander and Tiitinen 34 , Reference Nestel, Fujii and Zhang 37 , Reference Teede, Dalais and Kotsopoulos 39 , Reference Tormala, Appt and Clarkson 51 ). The consumption logs and 3-d food recalls indicate that participants consumed the study powders and followed the instruction to avoid other soya-containing foods; thus, it is unlikely that these factors are responsible for our null findings. Multiple CVD risk factors, including both traditional and emerging risk factors, were also assessed. Although few of our female participants were premenopausal, vascular function may have been assessed in these women during different phases of their menstrual cycle. Future studies should test premenopausal female participants during the 1st week of their menstrual cycle to minimise potential hormonal effects on vascular function. Furthermore, due to the number of treatments, the duration of individual supplementation periods was constrained in order to maximise participant retention for the duration of the study. Longer periods of supplementation may be required to alter cholesterol efflux and/or macrovascular function. Additional research is also needed to directly compare the effects of soya protein, soya isoflavones and their combination.
Conclusions
Supplementation with an isoflavone-containing soya protein isolate (delivering 25 and 50 g/d soya protein) for 6 weeks did not alter ex vivo cholesterol efflux or measures of macrovascular function in adults with moderately elevated brachial blood pressure. Additional research is needed to determine whether consumption of a soya protein isolate with naturally occurring isoflavones reduces CVD risk by modifying ex vivo cholesterol efflux and macrovascular function. Future studies should employ longer periods of supplementation, and should be powered to analyse differences between equol producers and non-equol producers.
Acknowledgements
The authors would like to thank our research participants for their dedication to the project, as well as the nursing and clinical staff of the Clinical Research Center of the Pennsylvania State University. The authors would also like to acknowledge Cameron Lusk and Glenna Hughes for formulating and producing the soya and control powders.
DuPont Nutrition and Health provided financial support for the study. This project was also supported by the Penn State Clinical & Translational Research Institute, Pennsylvania State University Clinical and Translational Science Award (CTSA), National Institutes of Health/National Center for Advancing Translational Science (NIH/NCATS) grant no. UL1 TR000127. The contents are solely the responsibility of the authors and do not necessarily represent the official views of the NIH or NCATS. P. M. K.-E. received funding from DuPont Nutrition and Health for the current study. Financial supporters had no role in the design and conduct of the study; in the collection, analysis and interpretation of the data; or in the preparation, review or approval of the manuscript.
The authors’ responsibilities were as follows: C. K. R., A. C. S.-R., J. A. F., R. M., E. S. K. and P. M. K.-E. designed the research; A. C. S.-R. and C. K. R. provided assistance with the implementation of study procedures and data collection; C. J. L. conducted the research; C. K. R. performed the statistical analyses; and C. K. R., A. C. S.-R., J. A. F. and P. M. K.-E. wrote the manuscript. All authors take responsibility for the manuscript’s final content.
E. S. K. was employed by DuPont Nutrition and Health while this study was conducted, analysed and prepared for publication. R. M. is employed by DuPont Nutrition and Health. C. K. R., A. C. S.-R., J. A. F. and C. J. L. have no conflicts of interest to declare.
Supplementary material
For supplementary material/s referred to in this article, please visit https://doi.org/10.1017/S000711451700143X