INTRODUCTION
Humans have been transporting vertebrates to islands worldwide since prehistoric times. This has more often than not impacted native flora and fauna (Russell et al. Reference Russell, Meyer, Holmes and Pagad2017), and Darwin (Reference Darwin1859) remarked in this context, ‘Let it be remembered how powerful the influence of a single introduced tree or mammal has shown to be.’ The successful introduction of such exotics has often led to the extinction of native species (Atkinson Reference Atkinson, Western and Pearl1989; Groombridge et al. Reference Groombridge1992; Courchamp et al. Reference Courchamp, Chapuis and Pascal2003; Long, Reference Long2003), especially on small, isolated islands. Invasive rats (Rattus rattus, Rattus norvegicus, Rattus exulans) are likely responsible for the greatest number of extinctions on islands (Towns et al. Reference Towns, Atkinson and Daugherty2006). Not only do they compete ecologically with native species, they also evolve within short historical periods (Rowe-Rowe & Crafford Reference Rowe-Rowe and Crafford1992; Michaux et al. Reference Michaux, Cucchi, Renaud, Garcia-Talavera and Hutterer2007; Renaud et al. Reference Renaud, Hardouin, Pisanu and Chapuis2013; Lister & Hall Reference Lister and Hall2014). Additionally, invasive species may also alter the evolutionary pathway of native species by mechanisms such as niche displacement, hybridization, introgression and predation (e.g. Mooney & Cleland Reference Mooney and Cleland2001; Stuart Reference Stuart2014; van der Geer et al. Reference van der Geer, Lyras, Lomolino, Palombo and Sax2013). The timescale and direction of evolution of invasive rodents are, however, insufficiently known. Data on how they evolve in interaction with native species are urgently needed with the increasing pace of introductions today due to globalized transport: invasive rodent species are estimated to have already colonized more than 80% of the world's island groups (Atkinson Reference Atkinson and Moors1985).
Here, I present data from a ubiquitous invasive species, the Polynesian rat (R. exulans), which provides an excellent proxy to measure and model evolutionary changes for several reasons. First, it vastly extended its geographic range through human-mediated transport (Roberts Reference Roberts1991) (Fig. 1) and is currently a commensal in islands of the Lesser Sunda Archipelago, the Moluccas, Sulawesi, the Philippines, New Guinea, New Zealand and practically all inhabited Pacific islands (Corbet & Hill Reference Corbet and Hill1992; Musser & Carleton Reference Musser, Carleton, Wilson and Reeder2005), and has recently also been recorded in Taiwan and the southern Ryukyu Islands (Motokawa et al. Reference Motokawa, Lu, Harada and Lin2001). Second, the introduction of this species has a large temporal range, starting c. 3500 years ago (Roberts Reference Roberts1991; Wilmshurst et al. Reference Wilmshurst, Anderson, Higham and Worthy2008). Third, it is not limited to urban and agricultural areas, but also occurs in disturbed forests (e.g. Rabor Reference Rabor1977; Danielsen et al. Reference Danielsen, Balete, Christensen, Hoegaard and Jakobsen1994) and primary forests without native rodents (Heaney et al. Reference Heaney, Heideman, Rickart, Utzurrum and Klompen1989), as well as in dry deciduous forests, mangrove forests and lowland coastal forests (Molur et al. Reference Molur, Srinivasulu, Srinivasulu, Walker, Nameer and Ravikumar2005). It is an adept climber and builds nests in small trees and hedges (Thomson Reference Thomson1922), and especially the canopy of coconut palms provide nesting and feeding opportunities (Wegmann et al. Reference Wegmann, Buckelew, Howald, Helm, Swinnerton, Veitch, Clou and Towns2011). Its native range is the tropics and subtropics, but its colonization success elsewhere shows that it thrives equally well in temperate maritime climates. Lastly, rats in general have the potential to show evolutionary changes within a relatively short time span due to their high reproduction rate.

Figure 1 Diagrammatic map showing simplified routs of human migration (after Roberts Reference Roberts1991; Austin Reference Austin and Ota1999). The dispersal of the Polynesian rat (Rattus exulans) has been facilitated by humans since prehistoric times. Populations in Oceania were introduced about 3000–3500 years ago (Roberts Reference Roberts1991; Austin Reference Austin and Ota1999), in Hawaii about 1200–1700 years ago (Kirch Reference Kirch2001) and in New Zealand about 800 years ago (Matisoo-Smith et al. Reference Matisoo-Smith, Allen, Roberts, Irwin, Lambert, Galipaud and Lilley1999). Light grey (orange in online version): native and introduced range of the Polynesian rat. White: areas without Polynesian rats.
The combination of large geographic and temporal scales thus makes the Polynesian rat an ideal species to test potential evolutionary pathways in invasive species. For most islands, there was only a single colonization event (Barnes et al. Reference Barnes, Matisoo-Smith and Hunt2006). The colonization history can be traced using rat haplogroup mtDNA (Matisoo-Smith & Robins Reference Matisoo-Smith and Robins2004), archaeological and genetic data and linguistic evidence of the Polynesians (Lipson et al. Reference Lipson, Loh, Patterson, Moorjani and Ko2014). Populations in Oceania were first established some 3000–3500 years ago (Roberts Reference Roberts1991), whereas Hawaii, New Zealand and Rapa Nui (Easter Island) were reached much later (c. 1190–1290 CE; Wilmshurst et al. Reference Wilmshurst, Hunt, Lipo and Anderson2011). The rats’ differing morphologies among the various islands were long noted (e.g. Motokawa et al. Reference Motokawa, Lin and Lu2004) and are reflected in the long list of junior synonyms (e.g. Mus maorium) and subspecies status such as Rattus exulans negrinus for the Negros small field rat.
My aim is to test whether the body size of insular populations of the Polynesian rat varies in correlation with biotic and abiotic variables. Here, I take samples from the entire introduced and native range. This vast geographic range ensures the presence of a large variety of islands with various geographic features, with and without mammalian competitors and predators.
The hypothesis is that body size evolution of this introduced mammal on the various islands complies with predictions based on the island rule, a graded trend according to which populations of small-sized species evolve larger body sizes on islands (gigantism) (sensu Foster Reference Foster1964; Van Valen Reference Van Valen1973). The island rule, or island syndrome, was originally formulated for native species and populations, but appears to apply to populations of introduced commensal rats as well (e.g. Russell et al. Reference Russell, Ringler, Trombini and Le Corre2011; van der Geer et al. Reference van der Geer, Lomolino and Lyras2018). According to an ecological interpretation of the trend (Lomolino et al. Reference Lomolino, Sax, Palombo and van der Geer2012, Reference Lomolino, van der Geer, Lyras, Palombo, Sax and Rozzi2013; van der Geer et al. Reference van der Geer, Lyras, Lomolino, Palombo and Sax2013), the direction and degree of size change depends on a combination of biotic and abiotic factors, with an especially important role for co-occurring mammalian species. In species-rich systems, competition and predation are expected to result in diversification and ecological displacement among resident species (native as well as long-term introduced species). In species-poor systems such as remote islands, release from these ecological interactions is expected to reverse these trends and murids are expected to become larger (Lomolino Reference Lomolino2009). This character release enables the colonizer to expand its range, habitat or resource usage, opening up the possibility to evolve in a new direction (Gillespie Reference Gillespie, Gillespie and Clague2009).
The null hypothesis is that body size of introduced populations of the Polynesian rat cannot be predicted based on the following variables: island size (a proxy for species and habitat diversity); latitude (a proxy for mean environmental temperatures and a weak correlate of primary productivity); number of mammalian species (a measure of ecological interactions); and maximum elevation (a measure of topographic relief, habitat diversity and the influence of anthropogenic activities, which tend to dominate lower elevations).
MATERIALS AND METHODS
Dataset
Extensive collections of the Polynesian rat are available, representing the entire introduced range. The dataset includes 105 populations (n = 587) of this species introduced onto islands and six mainland populations (n = 16) from Southeast Asia (Vietnam, Laos, peninsular Malaysia, Thailand, Myanmar and Sumatra) for comparison from the following institutes: AMNH (American Museum of Natural History, New York), AMS (Australian Museum, Sydney), AWMM (Auckland War Memorial Museum), LRNZ (Landcare Research, Lincoln), FMNH (Field Museum of Natural History, Chicago), MNZ (Museum of New Zealand Te Papa Tongarewa, Wellington), RMNH.MAM (Naturalis Biodiversity Center, Leiden; formerly Rijks Museum voor Natuurlijke Historie), NHM (Natural History Museum, London), QM (Queensland Museum, Brisbane), USNM (National Museum of Natural History, Washington, DC) and ZMB (Museum für Naturkunde, Berlin).
The data consist of skull size (condylobasal length (CBL); see figure in Supplementary Table S1, available online), relative body mass, island area, latitude, maximum elevation and the number of mammalian species (total as well as number of competitors and predators). As far as possible, only specimens with advanced dental wear (stages IV or V; King Reference King2006) were measured, as rodents grow slightly after they reach maturity (Roach et al. Reference Roach, Mehta, Oreffo, Clarke and Cooper2003). Polynesian rats do not show significant sexual dimorphism, and data from males and females were pooled. Relative body mass (S i) is the size index relative to the size of the averaged mainland body size, calculated with the cubic law, based on the CBL, where S i = 1 indicates mainland body size, whereas S i = 2 stands for double the mainland body size. Island area was log10 transformed prior to analyses in order to meet assumptions of normality and homogeneity of variance. Data on the number of co-occurring mammalian terrestrial species for each focal island were taken from various published sources (see Supplementary Table S2). Geographical data (surface area, latitude, longitude and maximum elevation) for the focal islands were taken from the islands website of the United Nations Environment Programme (islands.unep.ch) or, for islands not included in this database, from alternative sources (see Supplementary Table S2). Isolation was not included as an independent variable because this was considered irrelevant to species introductions, except in terms of the effects of isolation on the diversity of co-occurring species, which were recorded as independent variables in our analyses.
Analyses
Regressions, correlations and multivariate regressions were performed using Past 3.16 (Hammer et al. Reference Hammer and Harper2001) with a significance level p = 0.05. Normality of the dataset was tested with the Shapiro–Wilk test (Shapiro & Wilk Reference Shapiro and Wilk1965).
RESULTS
The 95 islands vary in size (0.1–785 753 km2; mean 15 592.1 km2), absolute latitude (0.25–47.21°; mean 16.5°), maximum elevation (1–5030 m; mean 872 m), numbers of ecologically relevant native predators (n = 0–13; mean n = 0.2) and native competitors (n = 0–73; mean n = 2.3) and total number of mammalian species (n = 1–164; mean n = 8.4) (Supplementary Table S1). The extraordinarily high maximum value for competitors/mammalian species is caused by the very high number of native murids in New Guinea. All variables are normally distributed (p < 0.05).
Mean CBL of the insular populations varied between 27.8 mm for Normanby Island (Papua New Guinea) and 35.2 mm for Nuku Hiva (Marquesas), with a mean of 31.2 mm, whereas that of the mainland sample is 27.6 mm (Southeast Asia) (Supplementary Table S2). A still larger value (36.3 mm) might be presented by the population of Tulaghi (Solomon Islands), but because this value is based on a single specimen, I exclude Tulaghi from further discussion. S i varied accordingly between 1.02 and 2.07 (mean 1.46) for introduced populations (Table 1). All introduced insular populations evolved larger average skulls than mainland populations (Fig. 2), except for the populations from Normanby, Sulawesi and Fergusson (<0.05% size difference compared with the mainland population). The largest specimen is from Guadalcanal, Solomon Islands (CBL = 39.1 mm; S i = 2.94), but this specimen is an outlier compared to other specimens from Guadalcanal and is excluded here as it may represent a black rat instead. The largest specimens are from Pitcairn (S i = 2.44) and Rapa Iti (S i = 2.41). Island populations with a mean body mass twice that of their mainland conspecifics or heavier are found in remote Eastern Polynesia (Henderson, S i = 1.92; Pitcairn, S i = 2.04; Nuku Hiva, S i = 2.07) and New Zealand (Stanley, S i = 1.94; Red Mercury, S i = 1.95).
Table 1 List of islands with mean relative body sizes of introduced populations of Polynesian rats (Rattus exulans). The symbol S i stands for the size index relative to the size of the averaged mainland body size, using the cubic law of relative skull length (condylobasal length; CBL), where mainland skull length is 27.6 mm: S i = (CBL (island)/CBL (mainland))3. Populations showing a considerable degree of gigantism, reflected in an S i that varies between 1.50 and 1.90, are highlighted in bold. Populations that doubled their body size (S i >1.90) are highlighted in italics. For ranges and numbers of specimens per island, see Supplementary Table S2.


Figure 2 An example of insular gigantism in the introduced Polynesian rat: adult female specimens from (a) Rose Atoll, Samoa (USNM 532898) and (b) Quảng Trị, Vietnam (RMNH.MAM.22559).
The data support the influence of absolute latitude on body size evolution of the Polynesian rat. Introduced populations evolved to larger body sizes on islands located in the higher latitudes (Fig. 3(a); Pearson r = 0.4586, p < 0.01). Introduced populations further appear to exhibit the graded trend of progressively increasing skull length with decreasing island area (Fig. 3(b); Pearson r = –0.4472, p < 0.01), with, however, much variation. Area is merely a proxy for habitat diversity and a measure for biodiversity: island area is indeed positively and significantly correlated with the total number of species (Supplementary Table S1; Pearson r = 0.5368, p < 0.01). In addition, there is a strong positive correlation between island area and maximum elevation (Supplementary Table S1; Pearson r = 0.7631, p < 0.01). Accordingly, body size of the Polynesian rat is negatively correlated with maximum elevation (Supplementary Table S1; Pearson r = –0.3033, p < 0.01), implying that the largest sizes are found on low-lying islands.
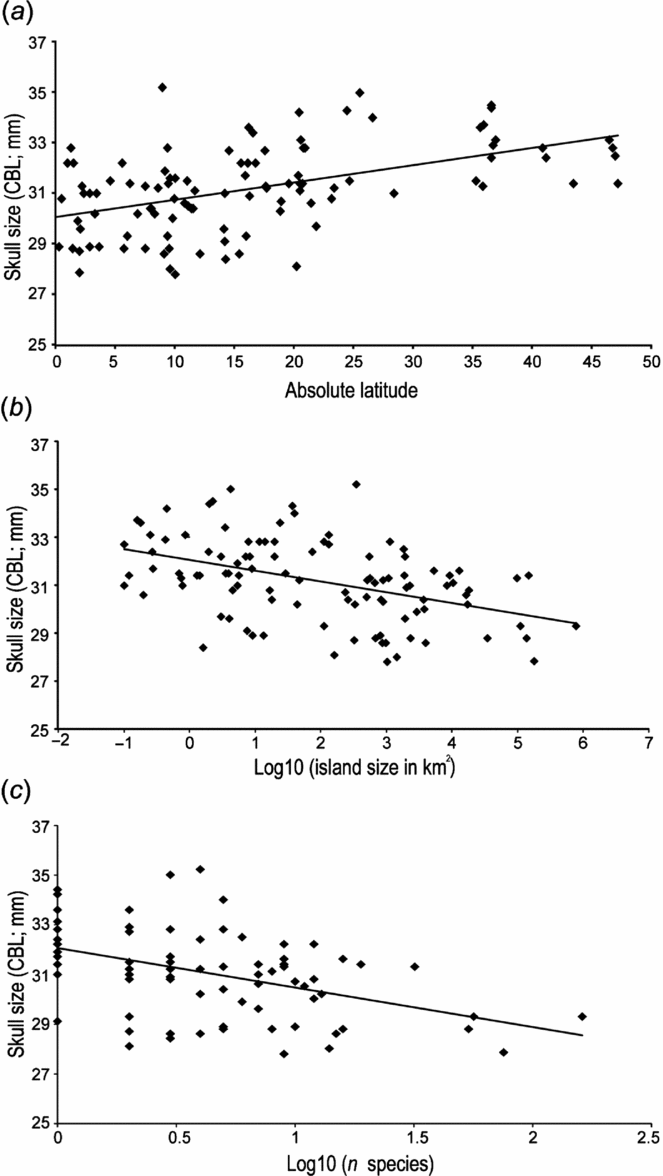
Figure 3 Relationships between skull size of introduced island populations of the Polynesian rat (a) with latitude (Pearson r = 0.4586, p < 0.01, r 2 = 0.2475), (b) island area (log-transformed; Pearson r = –0.4472, p < 0.01, r 2 = 0.1949) and (c) the total number of mammalian species (log-transformed; Pearson r = –0.4411, p < 0.01, r 2 = 0.1911). CBL = condylobasal length.
The ecology of evolution, with introduced species exhibiting ecological character release and displacement – evolving to larger size on islands with fewer mammalian predators and competitors – is confirmed by the data, with a negative correlation between skull size and total number of mammal species (Supplementary Table S1; Pearson r = –0.2912, p < 0.01). In a log-transformed analysis, in order to level the effect of New Guinea, Luzon, Mindanao, Java and Sulawesi, which are outliers regarding their extraordinarily high biodiversity levels compared to the majority of the islands, but excluding mammal-free islands, the negative correlation still holds (Fig. 3(c); Pearson r = –0.4411, p < 0.01). Furthermore, skull size is negatively correlated with native as well as alien competitors (Pearson r = –0.27 and –0.21, p = 0.01 and p = 0.04, respectively). The strong effect of total biodiversity and habitat diversity as shown by the significant correlations with island area (see above) might be a bias due to missing data on the number of nesting seabird species and other birds, which tend to be higher on small and low islands without mammals. Additional analysis incorporating prey types as additional factor is, however, beyond the scope of the present study, and will be part of a future follow-up.
DISCUSSION
Compared to their conspecifics from the native, continental range, introduced insular populations of Polynesian rats have larger skull sizes, with the largest sizes found on the smaller islands without mammalian species (mainly Polynesia) and on islands with a temperate climate (New Zealand and surrounding islands). The invasive success of insular populations of the Polynesian rat is likely greatly enhanced by its highly opportunistic foraging nature, enabling it to survive on a great variety of plant and animal matter. Its diet includes rice, coconuts, maize, palms, sweet potatoes, white potatoes, cassava, sugarcane (Wood Reference Wood, Buckle and Smith1994), pineapples (Twibell Reference Twibell1973), mangrove propagules (Steele et al. Reference Steele, Ewel and Goldstein1999), arthropods and, to a lesser degree, seabirds, forest birds, land snails and lizards (Atkinson & Towns Reference Atkinson, Towns and King2005; Shiels & Pitt Reference Shiels, Pitt, Timm and O'Brien2014). Generally, its diet is dominated by plant material (Shiels et al. Reference Shiels, Flores, Khamsingh, Krushelnycky, Mosher and Drake2013), sometimes of a single source (e.g. 87.6% of its diet on coral atolls in remote Oceania consists of coconuts found on the ground) (Moseby et al. Reference Moseby, Wodzicki and Thompson1973). Grass seed is an especially important component, and its abundance coincides with population peaks on temperate islands (Hitchmough Reference Hitchmough1980; Bunn & Craig Reference Bunn and Craig1989). These versatile eating habits and its wide fundamental niches enable it to occupy all available habitats on the islands at varying densities depending on food availability, habitat suitability and number of ecologically relevant competitors and predators (Harper & Bunbury Reference Harper and Bunbury2015).
Latitude is positively correlated with skull size of introduced Polynesian rats, in accordance with the spatial and temporal trends associated with Bergmann's rule (Bergmann Reference Bergmann1847; Smith et al. Reference Smith, Betancourt and Brown1995), whereby the body size of homoeothermic animals tends to increase with latitude. Explanations for this include those based on thermoregulation, physiological endurance during harsher winter conditions and ecological interactions. Heavier individuals have more insulation and greater reserves relative to the energy needs for thermoregulation and survival under winter conditions (McNab Reference McNab2002). Larger mammals can also exploit a broader dietary range (McNab Reference McNab1971). The results presented here on skull size and latitude confirm those of other studies on introduced Polynesian rats (Yom-Tov et al. Reference Yom-Tov, Yom-Tov and Moller1999; Atkinson & Towns Reference Atkinson and Towns2001).
The negative correlation between island area and skull size is the result of a combination of ecologically important factors that appear to be significantly correlated with island size: total number of species and maximum elevation (see Russell et al. Reference Russell, Clout and McArdle2004 and below). The data presented here confirm the positive relationship between island area and total number of terrestrial mammal species for the islands in the Pacific Ocean. As observed by Gillespie et al. (Reference Gillespie, Claridge and Roderick2008), the impact of introduced species appears to be highly dependent on the properties of the native communities and how these communities were originally assembled. Skull size of the Polynesian rat is indeed negatively correlated with the total number of native species: the more resident species (described by the island species–area relationship; e.g. see van der Geer et al. Reference van der Geer, Lomolino and Lyras2017), the smaller the skull size of the rats. The data here presented confirm earlier observations (Yom-Tov et al. Reference Yom-Tov, Yom-Tov and Moller1999) on the negative correlation of Polynesian rat skull size with the number of other rodents on the same island. Competition with another invasive rat, the black rat, seems especially important: the Polynesian rat tends to be outcompeted or displaced to more remote areas by the black rat (Atkinson & Towns Reference Atkinson, Towns and King2005; Shiels et al. Reference Shiels, Flores, Khamsingh, Krushelnycky, Mosher and Drake2013; Russell et al. Reference Russell, Sataruddin and Heard2014, Reference Russell, Caut, Anderson and Lee2015). The mechanism of this competition is incompletely understood (Taylor Reference Taylor1975). However, a change in habitat use occurs, with Polynesian rats in primary forests being more arboreal when black rats are present (McCartney Reference McCartney1970; Twibell Reference Twibell1973), whereas they are found more often on the forest floor when the larger congenerics are absent (Wegmann et al. Reference Wegmann, Buckelew, Howald, Helm, Swinnerton, Veitch, Clou and Towns2011), indicating ecological displacement.
Potentially, the larger skull sizes found at higher latitudes (New Zealand) as well as on small islands without other mammals (Micronesia, Polynesia) may alternatively be explained by a founder effect or a positive selection for larger individuals by the Polynesians on their way to the more distant archipelagos. If valid, one would expect the largest sizes in Micronesia, Hawaii and New Zealand and intermediate sizes en route. However, the distribution of relative body size values over the Pacific does not confirm such an explanation. Low values are also found in Remote Oceania (e.g. Line Islands, Austral Islands, Tuamotus), while vice versa, high values are also found in Near Oceania (e.g. Solomon Islands). The relative body size values of Hawaii do not differ from those found on the Maluku Islands, Mindanao and the Solomon Islands. The morphological data thus do not show a clear founder effect. A study on population genetics to address this issue in detail is currently in progress.
Two factors may explain larger skull size on topographically simpler islands. Low-lying islands support, on average, a higher human population and a higher degree of habitat disturbance, factors that promote the success of invasive rodents (van der Geer et al. Reference van der Geer, Lomolino and Lyras2018). Many of the smallest islands are flat atolls with coconut plantations, which provide excellent shelter and food (see above). In addition, uninhabited or sparsely inhabited small islands often harbour breeding colonies of seabirds. In house mice, a strong positive effect has been observed on a few islands of such breeding colonies on body size. The world's largest feral house mice (almost twice the ancestral size; S i = 1.89) are found on Gough Island in the South Atlantic (Rowe-Rowe & Crafford Reference Rowe-Rowe and Crafford1992). This impressive body size increase is entirely due to a switch to a higher degree of carnivory, whereby the mice eat live seabird chicks (Gray et al. Reference Gray, Wegmann, Haasl, White and Gabriel2014; Cuthbert et al. Reference Cuthbert, Wanless, Angel, Burle and Milton2016). The body size increase of the Polynesian rat is, however, even more impressive: slightly more than twice the ancestral size. Sea birds generally form at most a minor part of the diet of Polynesian rats; for example, at most 8% on Kure Atoll (Wirtz Reference Wirtz1972), yet the Kure rats reached 1.42-times the mainland body size, suggesting a potentially more important role of birds as prey. On Henderson Island (Pitcairn Islands, South Pacific), where it almost doubled its size (S i = 1.92), the Polynesian rat is held potentially responsible for the local extinction of the sandpiper (Prosobonia sp.) (Wragg & Weisler Reference Wragg and Weisler1994). The uninhabited island is a World Heritage site and one of the world's last two raised coral atolls with a relatively unaltered ecosystem, with a remarkable avian and floral biodiversity for its size (c. 37.3 km2), to which this invasive rat constitutes a serious threat. Chicks of two of its petrel species (Pterodroma ultima and Pterodroma neglecta) have been observed being eaten by the rat (Brooke Reference Brooke1995). The Henderson Island rats may thus represent the rat parallel to the Gough Island mice. This invasive species has a substantial impact on the breeding success of several seabirds on other islands as well by predation on eggs and chicks (overview in Harper & Bunbury Reference Harper and Bunbury2015) as much as the impact of black and brown rats (R. rattus and R. norvegicus) (Jones et al. Reference Jones, Tershy, Zavaleta, Croll, Keitt, Finkelstein and Howald2008). Polynesian rats even fatally attack adult Laysan albatrosses (Phoebastria immutabilis), as in the Leeward Islands of Hawai‘i, likely associated with reduced fruiting of food plants after storms (Atkinson & Towns Reference Atkinson and Towns2001). Carnivory has also been observed, for example, on Adele Island, a small, uninhabited island off the coast of northern Australia and a major breeding site for several seabirds, where the rats have fed on chicks and birds since their arrival c. 130 years ago (Boyle et al. Reference Boyle, Swann, Willing, Gale and Collins2004). Most avian species losses in the Pacific are unknown as they often occurred during human dispersal in south-eastern Polynesia and likely included flightless rails, pigeons, lorikeets and reed-warblers from nearly every larger vegetated island (Graves Reference Graves1992; Steadman Reference Steadman2006; Tennyson & Martinson Reference Tennyson and Martinso2006).
CONCLUSION
Introduced insular populations of Polynesian rats have larger skull sizes, with the largest sizes found on islets and atolls in the lower latitudes. The smallest sizes are found on islands rich in native mammals. The skull sizes of these introduced rats are positively correlated with latitude, likely associated with Bergmann's rule, but are negatively correlated with number of species, likely through ecologically important drivers such as competition and predation. Introduced insular populations of the Polynesian rat confirm the ecological explanation of the island rule.
Polynesian rats have a larger skull size on topographically simpler islands. Several factors may play a role in this, in combination or independently: (1) low-lying islands support, on average, a higher human population and a higher degree of habitat disturbance; (2) many of the smallest islands are flat atolls with coconut plantations, which provide shelter, nesting opportunities and abundant food; and (3) uninhabited or sparsely inhabited small islands often harbour breeding colonies of seabirds, potentially resulting in increased predation on eggs and chicks. The combination of seabird predation with increasing body size up to almost twice the size of mainland rats suggests that seabird populations presently coexisting with these rodents may become increasingly vulnerable in the future.
ACKNOWLEDGMENTS
I am much indebted to colleagues from numerous museums and institutes for providing data and other information. I thank Ross MacPhee, Neil Duncan, Marisa Surovy and Eleanor Hoeger (American Museum of Natural History), Steve Van Dyck and Heather Janetzki (Queensland Museum), Sandy Ingleby (Australian Museum), Steven van der Mije, Pepijn Kamminga and Wendy van Bohemen (Naturalis Biodiversity Center), Darrin Lunde and Nicole Edmison (United States National Museum of Natural History) and Lawrence Heaney and the late William Stanley (Field Museum of Natural History) for permission to access the collections under their care. I further thank George Lyras for making the figures and part of the data collecting and Bartholomeus van der Geer for his assistance in the collections. I thank James Russell and two anonymous reviewers for their comments and suggestions that improved the manuscript.
FINANCIAL SUPPORT
This work was supported the Netherlands Organisation for Scientific Research (NWO grant 016.veni.181.041).
CONFLICT OF INTEREST
None.
ETHICAL STANDARDS
None.
Supplementary material
To view supplementary material for this article, please visit https://doi.org/10.1017/S0376892918000085