In the last decade, there has been intense interest in the potential health benefits of dietary-derived plant polyphenols. An ever-increasing number of studies have described their antioxidant properties and linked this to their proposed role in the prevention of human disease. Plant polyphenols are abundant in the human diet, particularly in fruit, vegetables and pulses which have been consistently associated with a decreased risk of cancerReference Steinmetz and Potter1–Reference Le Marchand3, CVDReference Gardner4–Reference Jenkins, Kendall, Marchie, Jenkins, Connelly, Jones and Vuksan6 and a range of other chronic disorders. The likely active components of fruits, vegetables and pulses are a group of phytochemicals, known as polyphenols. However, it has proved extremely difficult to quantitatively establish the benefit afforded by polyphenols for a number of reasons: (1) there is a great diversity of polyphenol content between foods; (2) there is limited data regarding the polyphenol content of specific foods within the commonly-used food composition databases; (3) there are challenges in characterising and quantifying habitual food intake; and (4) there is a limited understanding regarding the extent of absorption and metabolic fate of individual polyphenols from particular foods.
Biological markers of nutrient exposure, as an alternative to the more traditional dietary assessment tools, have been used for many years. In this approach one or more biochemical moieties are measured in an accessible fluid or tissue to provide a semi-quantitative index of the exposure to individual food constituents. For polyphenols, this may appear to be an attractive approach. However, the relationship between dietary intake and resulting concentrations of biomarkers in body fluids is highly complex. Before a particular dietary component, or its metabolite, can be used as a sensitive and accurate biomarker of exposure to a specific polyphenol, a number of factors must be realised. Firstly, a full understanding of the metabolism of polyphenols in human subjects is required in order to select credible biomarkers. Secondly, it is important to understand the time–response relationship between polyphenol intake and the appearance of the biomarker in biological fluids. Thirdly, the precise dose–response relationship between the intake of a specific polyphenol and the appearance of its biomarker is essential. Finally, one must also have an understanding of the extent to which certain physiological and environmental factors affect the rate of polyphenol metabolism in human subjects. The current review will highlight the criteria that should be given consideration during the process of developing such biomarkers, and summarises the strengths and limitations of a number of potential biomarkers of polyphenol intake currently available.
Classification and structure of major dietary polyphenols
Polyphenols are naturally occurring non-nutritive plant compounds, which can be classified into several groups based on their structures. The main classes include flavonoids, phenolic acids, stilbenes and lignans. Fig. 1 illustrates the different groups of polyphenols with a representative compound from each group showing the characteristic chemical structure.
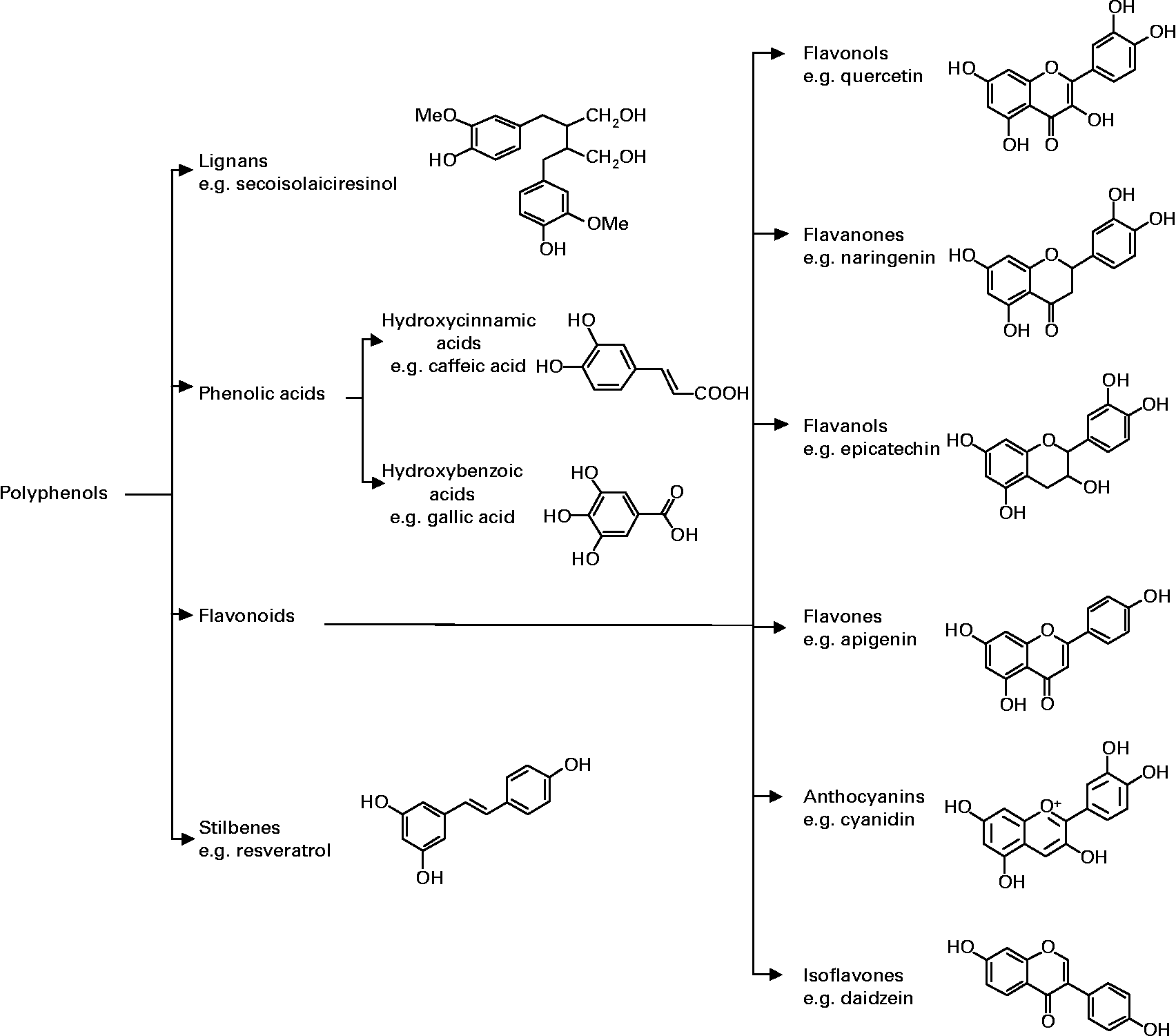
Fig. 1 Classification and chemical structure of major classes of dietary polyphenols.
One of the major classes of polyphenols is flavonoids which are identified by having a common structure consisting of two aromatic rings, which are bound together by three carbon atoms that form an oxygenated heterocycle (Fig. 1). Based on the variation in the type of heterocycle involved, flavonoids may be divided into six subclasses: flavonols, flavones, flavanones, flavanols, anthocyanins, and isoflavones. Individual differences within each group arise from the variation in number and arrangement of the hydroxyl groups and their extent of alkylation and/or glycosylation. Phenolic acids are abundant in food and can be classified into derivatives of benzoic acid and derivatives of cinnamic acid. Polyphenolic compounds also include stilbenes, which are compounds that possess 1,2-diarylethene structures and lignans characterised by a 1,4-diarylbutane structure.
Limitations in current methods used for estimation of dietary intake: need for biomarkers
In nutrition studies, the intake of nutrients and non-nutrient dietary components are usually determined using dietary assessment methods such as diet histories, FFQ or diet diaries. This food intake data is then translated into quantitative information regarding the specific food components of interest using food composition databases. Such methods rely on self-reporting of dietary patterns by the study participants, and consequently their accuracy is often uncertain. In particular, foods perceived as being unhealthy such as high-fat and -sugar products or processed foods are typically under-reported, with fruit and vegetable intakes, which represent the main dietary sources of polyphenols, often being over-reported.
Dietary assessment methods
The translation of food composition into intakes of specific dietary nutrients or non-nutrients is usually achieved using food composition databases. For polyphenols this approach is problematic, as currently available databases have only limited information on the diversity and concentration of polyphenols present in plant foods. These limitations arise because, unlike most nutrients, there has been only a limited systematic approach to comprehensively characterise and quantify polyphenols in plant foods using standardised analytical methods. In addition, much of the available information has been assembled from heterogeneous sources, in which the original food sampling and description is uncertain. For example, plant variety is a major determinant of the polyphenol composition, with up to a 4-fold variation in flavonoid and phenolic acid content being reported in applesReference Hammerstone, Lazarus and Schmitz7 and in yellow and red onionsReference Tsushida and Svzuki8.
Many other factors are also important. These include the ripeness of the plant food at time of harvest, environmental factors, such as UV exposure, and conditions of storage and processing. Studies investigating the effect of ripeness on the concentration of polyphenols in plants have shown that, in general, phenolic acid concentrations decrease during ripening, whereas the levels of anthocyanins increaseReference Manach, Scalbert, Morand, Remesy and Jimenez9. In addition, as many polyphenolic compounds possess anti-microbial activity in plants, their concentration in plant foods would be expected to be higher if plants had experienced bacterial or viral infection during growthReference Parr and Bolwell10, Reference Tomas-Barberan and Clifford11. This is observed in plants grown under organic conditions, where the polyphenol content has been reported to be higher than in non-organically grown varietiesReference Caris-Veyrat, Amiot, Tyssandier, Grasselly, Buret, Mikolajczak, Guilland, Bouteloup-Demange and Borel12. This is presumably due to the fact that organic crops are grown in the absence of exogenous herbicides and pesticides which encourages the synthesis of endogenous polyphenols in response to plant stress. Storage of plant-derived materials has also been shown to affect polyphenols content as many are easily oxidised. For example, storage of apple juice at room temperature results in a 60 % loss of quercetin and total loss of procyanidinsReference Spanos, Wrolstad and Heatherbell13, Reference Miller, Diplock and Riceevans14 whereas cold storage results in much smaller decreases in polyphenol contentReference van der Sluis, Dekker, de Jager and Jongen15.
Further complexity arises due to the uneven distribution of polyphenols in plant tissues and/or the loss or enrichment of specific polyphenols during food processing. For example, in apple, quercetin is found in the peel (1 mg/g fresh weight) with peeled fruit containing no flavonolsReference Burda, Oleszek and Lee16. Similarly, polyphenols in wheat grain are located predominantly in outer layers and are usually lost during refining of the flourReference Shahidi and Naczk17. In contrast, the processing of fruits into juices often results in the solubilisation or extraction of phenolic compounds from the skin, resulting in higher than expected concentrations in the juice. This is illustrated by the presence of significant levels of phloridzin in apple juice even though it is primarily confined to the pips of applesReference Spanos and Wrolstad18. Cooking may also have a major effect with both onions and tomatoes losing between 75 % and 80 % of their initial quercetin content following boiling, 65 % following micro-waving and 30 % after fryingReference Crozier, Lean, McDonald and Black19.
Impact of analytical methodology on published values for food polyphenol content
Along with the inherent variation in the polyphenol content of foods, the difficulty in accurately quantifying polyphenols has further contributed to the limitations of food composition databases. Indeed, standardised analytical methods for quantifying the polyphenol content of foods are lacking. Two main approaches have been used to quantify the polyphenol content of fruits, vegetables and beverages: (1) chromatography techniques, usually HPLC linked with various detection systems and (2) assays which measure total phenols, most notably those which quantify the reduction of the Folin-Ciocalteu reagentReference Scalbert, Hemingway and Laks20. These different techniques result in another source of variation in the measurement of polyphenol content. A measurement of polyphenol content by HPLC usually yields lower values than those that quantify on the basis of total polyphenol content. One reason for this is that some polyphenols may escape determination during HPLC analysis either because they are uncharacterised or they are not resolved by the chromatography. On the other hand, an overestimation may be generated by the Folin-Ciocalteu assay, as foods may contain components other than polyphenols, which act as reducing agents. A good example of such a component is ascorbic acid, which accounts for about 40 % of the estimated total phenols in potato and tomato but only about 5 % of that in onions and appleReference Scalbert and Williamson21. In spite of this problem, most authors still use data published >25 years ago which utilised the phenol quantification methodReference Kuhnau22.
The impact of all of these variables on food composition databases means that often they will provide an inaccurate representation of the true polyphenol content of the food consumed. Indeed, no accurate food-composition databases have been produced successfullyReference Manach, Scalbert, Morand, Remesy and Jimenez9, as they have for micronutrients such as vitamins. The considerable difficulties in assessing polyphenol intakes using traditional dietary assessment techniques highlight the need for rigorous biomarkers of intake which may circumvent these limitations.
Factors affecting choice of potential biomarkers of polyphenols intake
In order to serve as a useful biomarker of intake in nutrition studies, the criteria listed below need to be satisfied:
1. Quantification of the biomarker of interest should be qualitatively and quantitatively robust. Therefore, sensitive and specific techniques should be available to accurately quantify the biomarker of interest in tissue/biofluid, which has been appropriately collected and stored to ensure minimum biomarker degradation.
2. Concentrations of the biomarker in the tissue/biofluid of interest should be sensitive to changes in intake of the dietary component of interest.
3. The biomarker should be specific to the dietary component of interest, i.e. variation in its concentration should be due to changes in the intake of the dietary component of interest only.
4. The interpretation of dietary intake–biomarker relationships will require a clear understanding of the impact of physiological factors and whole diet composition on kinetics of absorption, metabolism and excretion of the putative biomarker.
The remainder of this review will focus on these points in relation to biomarkers of polyphenol intake.
Metabolic fate of polyphenols
Although the identity of many of the major polyphenols in plant foods is known, the identification of circulating and tissue metabolites, which could serve as potential biomarkers of intake has been undertaken for a small number of polyphenols only. In general, native polyphenols are subjected to extensive metabolism following ingestion. In the upper gastrointestinal tract, dietary polyphenols act as substrates for an array of enzymes leading to large differences in the proportion of polyphenols that are absorbed. During transfer across the jejunum and ileum, polyphenols are subject to extensive metabolism by phase I hydrolysing and oxidizing enzymes, including cytochrome P450 and glucosidase enzymes, and by phase II conjugating and detoxifying enzymesReference Spencer, Chowrimootoo, Choudhury, Debnam, Srai and Rice-Evans23–Reference Spencer25. The majority of aglycones, which escape metabolism in the small intestine, are subject to phase I and II metabolism in the liver following transfer via the portal bloodReference Spencer, Schroeter, Rechner and Rice-Evans24, Reference Day and Williamson26. Such enzymes include uridine diphosphate (UDP)-glucuronosyl transferases, which lead to the formation of O-glucuronides, sulpho-transferases, which generate O-sulphates and catechol-O-methyl transferases, which produce O-methylated metabolites. In addition, a large proportion of ingested dietary polyphenols escape absorption in the stomach and small intestine, including those re-excreted into the gut lumen via the bile, and pass to the large intestine where they are subjected to actions of the gut microfloraReference Spencer, Schroeter, Rechner and Rice-Evans24, Reference Rechner, Smith, Kuhnle, Gibson, Debnam, Srai, Moore and Rice-Evans27. The colon contains approximately 1012 microorganisms/cm3 which have enormous catalytic and hydrolytic potential and produce a wide range of metabolites which are subsequently absorbed into the systemic circulation from the large bowel. Knowledge of the extensive metabolism of flavonoids and polyphenols has challenged the concept that flavonoids are responsible for the large increases in plasma total antioxidant capacity observed after the consumption of flavonoid-rich foods. Many studies, reviewed nicely by Lotito and FreiReference Lotito and Frei28, have indicated that increases in plasma antioxidant capacity do not result due to plasma flavonoids, but are more likely the consequence of increased uric acid levels.
One of the most important factors thought to determine the subsequent metabolic fate of polyphenols in human subjects is the quantity ingested. There is a significant difference in the metabolite profile following the administration of polyphenols in pharmacological doses compared with that observed following consumption of normal dietary quantities. For example, after the intake of a large dose of pure catechin, free catechin is detected in the plasmaReference Hackett, Griffiths, Broillet and Wermeille29. In contrast, consumption of a single serving of red wine, containing 35 mg catechin, results in all the circulating catechin being detected in the conjugated formReference Bell, Donovan, Wong, Waterhouse, German, Walzem and Kasim-Karakas30. The appearance of free flavanol in the plasma is likely to be due to the saturation of phase I and II conjugating enzymes during the absorption of large amounts of free flavanol. In addition, the type of conjugate formed also appears to vary according to the dose ingested. Sulphation is generally a higher affinity, lower-capacity pathway relative to glucuronidation, so that as the ingested dose increases, a shift from sulphation toward glucuronidation occursReference Koster, Halsema, Scholtens, Knippers and Mulder31.
An essential requirement for the characterisation of a polyphenol metabolite as a potential biomarker of intake is the specificity of this metabolite. Such a metabolite should only appear in human plasma following the intake of a specific polyphenol and should not be formed as a product of the metabolism of any other compound either consumed or produced endogenously. Unfortunately, the ingestion of a number of polyphenols often leads to the formation of common metabolites in the circulation. For example, metabolism of polyphenols by the intestinal bacteria in the large intestine leads to the formation of common small-cleavage productsReference Spencer25. This has been observed with caffeic acid intake from coffee, where the colonic-derived metabolites, hippuric acid and 3-hydroxyhippuric acid, may also derive from the metabolism of: (1) quinic acid, (2) the aromatic amino acids tryptophan, tyrosine and phenylalanine and (3) other polyphenols which possess a hydroxyl group in the 3-position of the aromatic ringReference Rechner, Spencer, Kuhnle, Hahn and Rice-Evans32. Consequently, such metabolites are not useful biomarkers for the intake of caffeic acid. However, isoferulic acid may represent such a biomarker for caffeic acid intake as it has not been described as a major constituent of food and is only derived from 4-methoxylation of caffeic acid prior to or after its absorptionReference Rechner, Spencer, Kuhnle, Hahn and Rice-Evans32. Further research is required in order to expand our current knowledge regarding the biotransformation of the different classes of polyphenols and to assess the usefulness of such metabolites as biomarkers of intake.
Analytical techniques used to identify and quantify biomarkers
If specific metabolites are to be used as biomarkers for the intake of polyphenols, it is essential that analytical techniques for their measurement in biological samples are both sensitive and reliable. The circulating concentrations of both native and metabolic forms of polyphenols are in the nanomolar to low micromolar range and normally represent only a very small percentage of the amount consumed. Furthermore, the metabolic forms that predominate are difficult to characterise and/or quantify due to a lack of suitable standards. Consequently, the most common approach is to quantify the aglycones using HPLC coupled with UV spectra, fluorescence or electrochemical detection following the hydrolysis of polyphenol conjugates with the enzymes β-glucuronidase and/or sulphatase. However, accurate quantification may still be hampered due to the similar retention times of polyphenol conjugates, leading to misidentification and an inability to accurately quantify the metabolite of interestReference Aziz, Edwards, Lean and Crozier33, Reference Day, Mellon, Barron, Sarrazin, Morgan and Williamson34. The relatively recent application of HPLC–tandem mass spectrometry technology has provided a useful tool for the positive identification of compounds in biological fluids. This technique is likely to aid the identification and quantification of polyphenols and their metabolites in biological fluids such as plasma and urine. Finally, consideration should also be given to the stability of polyphenol metabolites during sample storage and during sample extraction. For example, anthocyanins are highly sensitive to changes in pH and may undergo ring opening or oxidation during sample extraction to yield forms that escape detectionReference Felgines, Talavera, Gonthier, Texier, Scalbert, Lamaison and Remesy35.
Response to the dose of polyphenol ingested
A prerequisite for a biomarker is that differences in consumption must be able to be discriminated i.e. the biomarker is quantitatively associated with intake. Bioavailability and/or pharmacokinetic studies have shown that plasma metabolite concentrations achieved following consumption of polyphenols vary greatly according to the nature of the polyphenol, and in particular the nature of the food source. For example, consumption of 80–100 mg quercetin glycosides results in plasma concentrations of quercetin metabolites between 0·3 and 0·75 μmol/l, whether the flavonol was consumed in the form of apples, onions, or meals rich in flavonolsReference Hollman, vanTrijp, Buysman, VanderGaag, Mengelers, Devries and Katan36–Reference Manach, Morand, Crespy, Demigne, Texier, Regerat and Remesy38. Similar plasma concentrations of catechin and epicatechin are observed following consumption of green tea (0·1–0·7 μmol/l for an intake of 90–150 mg)Reference Unno, Kondo, Itakura and Takeo39, cocoa (0·25–0·7 μmol/l for an intake of 70–165 mg)Reference Wang, Schramm, Holt, Ensunsa, Fraga, Schmitz and Keen40 or red wine (0·09 μmol/l for an intake of 35 mg)Reference Donovan, Bell, Kasim-Karakas, German, Walzem, Hansen and Waterhouse41. However, the plasma concentrations achieved following the administration of similar doses of different flavanols has been shown to vary greatly, with levels of catechin peaking at 0·1 μm and epicatechin peaking at 2·6 μm following the intake of 160 mg catechin and epicatechin, respectivelyReference Holt, Lazarus, Sullards, Zhu, Schramm, Hammerstone, Fraga, Schmitz and Keen42. If plasma concentrations are to be used as a biomarker of intake, more quantitative information regarding the relationship between dose administered and plasma concentrations is required.
A more complex picture emerges when one considers the form (i.e. the type of food) in which the polyphenol is ingested. The food source is likely to have a large impact on the dose–response relationship between intake and appearance of a specific metabolite. This is in part due to the fact that the food matrix may greatly influence absorption of polyphenols from the gastrointestinal tract and partly because polyphenols exist in a variety of chemical forms depending on the food source. The best-described example of the latter is the impact of glycosylation on flavonoid absorption. Many studies have indicated that it is polyphenol aglycones that are transferred across the enterocyte, with glucosides needing to be enzymatically hydrolysed, by β-glucosidases located in the gut mucosa or by bacterial enzymes, prior to absorption. Therefore, with the exception of glucosides, the efficiency of absorption of a particular polyphenol will be markedly reduced if the dietary form exists as glycosides. For example, the absorption of quercetin is greater following the ingestion of onions, which are rich in glucosides, compared with the ingestion of apples, which contain both glycosides and glucosidesReference Hollman, vanTrijp, Buysman, VanderGaag, Mengelers, Devries and Katan36. However, in some cases, polyphenol glucosides have been shown to be more efficiently absorbed than the free aglyconeReference Hollman, Devries, Vanleeuwen, Mengelers and Katan43, Reference Setchell, Brown, Desai, Zimmer-Nechemias, Wolfe, Brashear, Kirschner, Cassidy and Heubi44. This may relate to their transport into enterocytes by the Na-dependent glucose transporter, SGLT1, where they are hydrolysed by cytosolic β-glucosidase. Although the same metabolic pathways apply to phenolic acids, there are additional complications in that they are often esterified in plants and their absorption is influenced by the extent to which they are bound to complex polysaccharides in plant cell walls. The best-studied example is ferulic acid, which is often etherified to arabinoxylans in the outer husks of cereals. Whilst free ferulic acid is absorbed efficiently from tomatoes, leading to relatively high plasma concentrations, much lower plasma levels of ferulic acid are obtained following the ingestion of cerealsReference Bourne and Rice-Evans45.
Although not well studied, evidence suggests that variations in polyphenol absorption also occur due to interactions between polyphenols and other food components. For example, there are contradictory reports regarding the influence of milk on the absorption of flavonoids. Most studies indicate that milk does not affect the absorption of catechin, quercetin or kaempferol when added to green or black teaReference van het Hof, Kivits, Weststrate and Tijburg46, Reference Hollman, van het Hof, Tijburgh and Katan47, or the absorption of flavanols from chocolateReference Schroeter, Holt, Orozco, Schmitz and Keen48. However, another study indicated that plasma epicatechin levels were reduced by almost 2-fold when dark chocolate was accompanied by milk or when the chocolate itself contained milkReference Serafini, Bugianesi, Maiani, Valtuena, De Santis and Crozier49. Furthermore, bread- and sugar-containing meals have been shown to enhance the absorption of catechin and epicatechin from chocolate, with the authors suggesting that the carbohydrate composition of the meal may impact on flavonoid absorption through an effect on gastric secretions, motility and hepatic blood flowReference Schramm, Karim, Schrader, Holt, Kirkpatrick, Polagruto, Ensunsa, Schmitz and Keen50. Consequently, the dose–response relationship between polyphenol intake and the concentration of potential biomarker in plasma or urine may be influenced by both the chemical form of the polyphenol present in foods and the general food composition.
Lastly, there appears to be considerable inter-individual variation in the metabolic response to a given dose of a particular polyphenol-rich food. This is observed particularly in the case of metabolites produced by colonic microflora. For example, the formation of equol from daidzein in the gut is thought to be a clinically relevant step in the therapeutic potential of soya isoflavones, as the estrogenic potency of equol is 10-fold higher than its precursor daidzein. However, it is well recognised that adults differ in their ability to produce equol, with only 30–40 % of individuals producing equol following daidzein ingestionReference Lampe, Karr, Hutchins and Slavin51. This has been attributed to an absence of a specific enzyme-producing bacterial species in the large bowel. However, even for polyphenols for which absorption is not dependent on bacterial metabolism, there is considerable inter-individual variation in the dose–response relationshipReference Vitaglione, Sforza, Galaverna, Ghidini, Caporaso, Vescovi, Fogliano and Marchelli52. Such variability in absorption or post-absorptive metabolism may be attributed in part to variability in the expression of metabolising enzymes and transporters due to genetic variability within the population. Therefore, the dietary polyphenol–biomarker relationship may be dependent on inter-individual genetic variability as well as on the food source.
Kinetics of metabolite/biomarker following exposure
Another vital factor to consider when developing a potential biomarker of polyphenol intake is the kinetic profile of its metabolism and appearance in the biofluid. Although the metabolic pathways to which most polyphenols are subjected are similar, pharmacokinetic profiles differ markedly among the different classes of polyphenols. Anthocyanins are reported to possess the highest rate of absorption, with time to maximum concentration (T max) as low as 0.5 h following intake of blackcurrant juice, whilst flavanones have a T max in the range 4–6 hReference Erlund, Meririnne, Alfthan and Aro53, Reference Manach, Morand, Gil-Izquierdo, Bouteloup-Demange and Remesy54. The extended T max of flavanones and other flavonoids relative to anthocyanins probably results due to the requirement for de-glycosylation in the intestine prior to absorption. The effect de-glycosylation is highlighted by studies showing that the absorption kinetics of quercetin differ greatly depending on the type of glycoside conjugationReference Hollman, vanTrijp, Buysman, VanderGaag, Mengelers, Devries and Katan36, Reference Graefe, Wittig, Mueller, Riethling, Uehleke, Drewelow, Pforte, Jacobasch, Derendorf and Veit37. Quercetin from onions, which contain only glucosides, is rapidly absorbed (T max 0.7 h), whereas quercetin found in apples had a T max 2.5 h and pure quercetin-3-rutinoside, the major species in tea, showed a markedly delayed absorption (T max 9.3 h). These differing absorption profiles mean that in human investigations there will be different optimal time points for plasma or urine sampling. As metabolite concentrations are generally low in both urine and plasma, it is essential that biofluids are collected when these putative biomarkers are at their highest concentrations. Optimal sampling of both urine and in particular plasma will improve reliability of the biomarkers in human studies.
The time–response relationship between intake and appearance of the metabolite biomarker in plasma will also be dependent on the elimination rate of the metabolite. There is limited data regarding the precise half-lives of polyphenols in plasma. Data suggest that these half-lives are about 2 h for anthocyanins and flavanonesReference Erlund, Meririnne, Alfthan and Aro53–Reference Cao, Muccitelli, Sanchez-Moreno and Prior55 and 2–3 h for flavanolsReference Bell, Donovan, Wong, Waterhouse, German, Walzem and Kasim-Karakas30, Reference Richelle, Tavazzi, Enslen and Offord56, Reference Lee, Maliakal, Chen, Meng, Bondoc, Prabhu, Lambert, Mohr and Yang57. The rapid excretion of polyphenols is facilitated by the conjugation of aglycones or O-methylated forms to sulphate or glucuronic acid. This renders them more polar and thus they undergo more rapid renal excretion. However, some studies have suggested that flavonols, such as quercetin, are eliminated more slowly with half-lives of 11–28 hReference Graefe, Wittig, Mueller, Riethling, Uehleke, Drewelow, Pforte, Jacobasch, Derendorf and Veit37, Reference Hollman and Katan58. It has been suggested that this extended half-life may be due to the particularly high affinity of quercetin for plasma albuminReference Manach, Morand, Texier, Favier, Agullo, Demigne, Regerat and Remesy59, Reference Dangles, Dufour and Bret60. This explains the observation that repeated consumption of onions leads to the accumulation of quercetin in bloodReference Moon, Nakata, Oshima, Inakuma and Terao61. The extent to which protein binding contributes to the half-lives of polyphenols in plasma requires further study. However, before such a question can be effectively answered, one must solve the problem of how to extract polyphenols, including those which are protein bound. Such analytical problems as well as pharmacokinetic data need to be carefully considered when interpreting data from human intervention studies where fasted blood has been sampled. For example, the quantification of polyphenols in a 10–12 h fasting blood sample is unlikely to provide useful information regarding levels of polyphenol intakeReference van het Hof, Wiseman, Yang and Tuburg62.
Many studies to date have analysed plasma polyphenol levels 1–6 h post intake. Such sampling will provide good data regarding the polyphenol metabolites of small-intestinal origin. However, in the majority of cases, such a time course will not provide information regarding metabolic products formed in the large intestine. For example, the half-life of isoflavones is 4–10 h if calculated from measurements of sulphate and glucuronide conjugates onlyReference Setchell, Brown, Zimmer-Nechemias, Brashear, Wolfe, Kirschner and Heubi63, Reference Setchell, Brown, Desai, Zimmer-Nechimias, Wolfe, Jakate, Creutzinger and Heubi64. However, this increases to more than 2 d if one considers metabolites of colonic origin, for example the metabolite equolReference Lu, Grady, Marshall, Ramanujam and Anderson65. The delayed appearance of colonic metabolitesReference Lee, Maliakal, Chen, Meng, Bondoc, Prabhu, Lambert, Mohr and Yang57 may increase the usefulness of large gut metabolites as potential biomarkers of acute intake. However, due to the large variation in gut microflora between individuals, more research is required before considering the use of bacterial metabolites as potential biomarkers. Therefore, a sampling regime of between 1 and 3 h is recommended to maximise the chance of quantifying a blood-borne biomarker of polyphenol intake.
Choice of bio fluid: plasma v. urine
Multiple blood sampling over a 24 h period, and calculations of concentration via area under the curve, has often been used in intervention studies to determine the bioavailability of polyphenols. Urinary excretion of polyphenol metabolites is often also included in such human studies and is generally consistent with plasma kinetic data. However, 24 h urine sampling offers additional advantages over plasma measurements, mainly because it allows for total polyphenol absorption to be more accurately assessed. Urine sampling is particularly useful for polyphenols with short half-lives, where plasma measurements may fail to monitor even acute intake. The biggest advantage of analysing 24 h urinary output is that it is quantitative and will provide a measure of the total output of polyphenol metabolites over a 24 h period. This is likely to be a more robust may of monitoring daily intake than a single measurement in plasma, or indeed in urine. Twenty-four hour urinary data will also provide a better index of intake, as one may monitor the total concentrations of both small- and large-intestinal metabolites, without the need for multiple blood collections. For example, following the ingestion of a polyphenol-rich meal, the urinary profile indicated that the maximal amount of naringenin glucuronide occurred in the first 12 h while the maximal concentrations of the colonic metabolite 3-hydroxyphenylacetic acid was reached 60–84 h post ingestionReference Rechner, Kuhnle, Bremner, Hubbard, Moore and Rice-Evans66. Therefore, urinary measurements of polyphenol metabolites provide more comprehensive data regarding intake, as has been shown for other phytochemicals such as glucosinolates. Several studies have indicated that urinary levels of the metabolites of the glucoraphanin, the predominant glucosinolate in broccoli were proportional to the amount of broccoli eatenReference Conaway, Getahun, Liebes, Pusateri, Topham, Botero-Omary and Chung67, Reference Shapiro, Fahey, Wade, Stephenson and Talalay68.
Although urine sampling appears promising, urinary data may be misleading for certain polyphenols that use alternative routes of excretion. For example, although the systemic bioavailability and the maximum plasma concentration of genistein is higher than for daidzeinReference Setchell, Brown, Desai, Zimmer-Nechimias, Wolfe, Jakate, Creutzinger and Heubi64, a greater urinary excretion of daidzein is observedReference Xu, Wang, Murphy, Cook and Hendrich69. This appears to be because a greater fraction of genistein is eliminated in bileReference Sfakianos and Barnes70, Reference Sfakianos, Coward, Kirk and Barnes71. This explanation is substantiated by plasma kinetic curves in human subjects, which show an early peak of genistein and related metabolites in plasma which is closely followed by a second peak 3 h later reflecting enterohepatic circulationReference Setchell, Brown, Desai, Zimmer-Nechemias, Wolfe, Brashear, Kirschner, Cassidy and Heubi44, Reference Zubik and Meydani72. Considering the above information, it is clear that the usefulness of urinary polyphenol metabolite output as a biomarker of recent (12–72 h) intake remains to be fully established.
Assessment of biomarkers for exposure to specific dietary polyphenols in human blood and urine
The remainder of this review will focus on the potential biomarkers of polyphenol intake which are currently established. We comment on their strengths and limitations based on the criteria established in the previous section.
Flavonoids
Only a limited number of studies have attempted to assess the usefulness of plasma or urine levels of flavonoids as biomarkers of intake. Two such studies have investigated the use of fasting plasma and urine concentrations of quercetin as biomarkers of intake (Table 1). Consumption of three different doses of quercetin, ingested as fruit juice, resulted in a significant dose-dependent increase in urinary quercetin, indicating that urinary quercetin may serve as a quantitative biomarker of intakeReference Young, Nielsen, Haraldsdottir, Daneshvar, Lauridsen, Knuthsen, Crozier, Sandstrom and Dragsted73. In contrast, no dose–response relationship was evident for plasma levels of quercetin concentration in this study. Noroozi and co-workers investigated plasma quercetin concentrations in diabetic subjects consuming high-flavonol diets containing either 57 or 90 mg quercetin for 2 weeksReference Noroozi, Burns, Crozier, Kelly and Lean74. At the end of the study period, the mean fasting plasma concentrations were 48 (sd 12) μg/l and 87 (sd 27) μg/l, respectively. In addition, there was a close correlation between the fasting plasma and 24 h urinary concentration.
Table 1 The European Nutrigenomics Organisation plasma and urine values for representative polyphenols obtained from pharmacokinetic studies

C max, maximum concentration; EGC, epigallocatechin; FBV, fruit, beverage and vegetables; FV, fruit and vegetables.
A positive correlation between plasma concentrations and the intake of citrus flavanones has also been reported by Manach and co-workersReference Manach, Morand, Gil-Izquierdo, Bouteloup-Demange and Remesy54. Here a dose-dependent relationship between the maximum plasma concentration and 24 h urinary excretion of hesperetin and naringenin was observed. However, with a T max of approximately 5 h, plasma levels are only reflective of short-term ( < 12 h) intake. As with quercetin, the results suggest that 24 h urinary flavanone outputs may serve as a more useful biomarker of intake. However, the intake–urinary excretion relationship might be dependent on food source, an observation that requires further investigationReference Erlund, Meririnne, Alfthan and Aro53, Reference Manach, Morand, Gil-Izquierdo, Bouteloup-Demange and Remesy54, Reference Erlund, Silaste, Alfthan, Rantala, Kesaniemi and Aro75. Although positive correlations between dose and concentration in plasma have been observed in kinetic studies for flavonols and anthocyanins, no data are currently available regarding the usefulness of urinary excretion as a biomarker of intakeReference Wang, Schramm, Holt, Ensunsa, Fraga, Schmitz and Keen40, Reference Richelle, Tavazzi, Enslen and Offord56, Reference Nakagawa, Okuda and Miyazawa76. In contrast, although the plasma concentrations and urinary output of isoflavones follow a curvilinear relationshipReference Setchell, Brown, Desai, Zimmer-Nechimias, Wolfe, Jakate, Creutzinger and Heubi64 (Table 1) plasma and urinary isoflavone levels are considered as reliable semi-quantitative indices of intake. Concentrations of isoflavones and their metabolites were higher in fasting plasma and in 24 h urine from subjects who consumed a high-soya diet compared with a low-soya diet for 10 weeksReference Wiseman, Casey, Bowey, Duffy, Davies, Rowland, Lloyd, Murray, Thompson and Clarke77 (Table 1). In addition, urinary excretion differed between subjects having 35 mg isoflavone supplement/d for 3 months and those following a non-supplemented dietReference Ritchie, Morton, Thompson, Deighton, Blake, Cummings and Steel78 (Table 1), and the correlation coefficient (r) describing the relationship between 24 h excretion and isoflavone intake was 0·89. This positive correlation between intake and fasting plasma or urine excretion was used to validate a newly constructed phyto-oestrogen database, containing more than 600 values assigned to foods by using duplicate diet analysisReference Ritchie, Morton, Deighton, Blake and Cummings79. The correlations between estimated intake of phyto-oestrogens by fourteen subjects, measured by 7-d weighed intake, and urinary excretion (24 h) and plasma concentrations were highly significant (r 0·97 and 0·92, respectively). Therefore, in nutrition research both fasting plasma concentration and 24 h urinary output can be used as quantitative biomarkers of isoflavone intake.
Phenolic acids
The estimation of intake of phenolic acids presents problems similar to those for flavonoids. However, the identification of suitable biomarkers of phenolic acids has been hampered by findings that phenolic acid metabolites may also be formed from other dietary sources such as flavonoids (discussed earlier). The main dietary sources of the phenolics gallic acid and chlorogenic acid are tea and coffee respectively. A number of studies have highlighted the potential usefulness of the quantification of the metabolites of these compounds in plasma/urine as biomarkers of tea and coffee intake. A bioavailability study of caffeic acid in human volunteers consuming 100, 200 and 300 ml red wine, indicated that the plasma concentration achieved 1 h post-ingestion was dose-dependentReference Simonetti, Gardana and Pietta80 (Table 1). In another study conducted by Hodgson and co-workers, 24 h urinary excretion of 4-O-methylgalllic acid correlated significantly with usual (r 0·50, P < 0·001) and current (r 0·57, P < 0·001) tea intake. Isoferulic acid, a specific metabolite of chlorogenic acid, was also significantly but weakly related to usual (r 0·26, P = 0·008) and current (r 0·18, P < 0·001) coffee intakeReference Hodgson, Chan and Puddey81 (Table 1). In a recent study fifteen individual polyphenol metabolites were quantified in 24 h urine samples collected from volunteers following consumption of six different polyphenol-rich beveragesReference Ito, Gonthier, Manach, Morand, Mennen, Remesy and Scalbert82. This study highlighted the potential usefulness of the quantification of urinary epicatechin, chlorogenic acid and gallic acid as specific biomarkers of tea, coffee and wine intakes.
Use of flavonoids in intervention and epidemiological studies as biomarkers of the intake of, fruit and vegetables, and other plant-derived foods
Since flavonoids are widely distributed in fruits and vegetables, a number of studies have investigated their usefulness as potential biomarkers of fruit and vegetable intake. In a strictly controlled dietary intervention study, 24 h urinary outputs of flavonoids were measured following 6 weeks consumption of diets either low or high in fruits, vegetables and berriesReference Nielsen, Freese, Kleemola and Mutanen83 (Table 1). Urinary quercetin and flavanones, as well as total flavonoid, outputs were clearly higher after the high-vegetable diet compared with the low-vegetable diet. At the individual level, a significant positive correlation between changes in fruit and vegetable intake and changes in urinary flavonoid excretion was observed. Furthermore, there was a positive correlation (r 0·86) between total polyphenol metabolites in 24 h urine samples and increasing intake of fruit and vegetables following a 1-d consumption of a basic diet supplemented with 300 or 600 g fruit and vegetablesReference Krogholm, Haraldsdottir, Knuthsen and Rasmussen84 (Table 1). The total excretion of flavonoids in the morning urine also increased but the association was weaker (r 0·59). These studies provide evidence that 24 h urinary excretion of flavonoids may be used effectively as a biomarker for fruit and vegetable intake.
However, in epidemiological studies where the potential health effects of fruit and vegetables are investigated, 24 h urine samples are rarely collected. Instead only ‘spot’ urine samples or fasting plasma samples are collected. The usefulness of fasting plasma concentrations of selected flavonoids as markers of ordinary dietary intake has been investigated by Radtke and co-workersReference Radtke, Linseisen and Wolfram85. Mean intake estimates (7-d period) of quercetin, kaempferol, naringenin and hesperetin were estimated using literature data on the flavonoid content of foods. In addition, fasting plasma samples were taken at the end of the record period for flavonoid determination. For all four flavonoids, there were significant correlations between 7-d intake results and fasting plasma concentrations. For flavonoids with short half-lives, distinctly higher correlation coefficients were found for the relationship between intake estimates for the final day before blood sampling, and the fasting plasma concentrations. Furthermore, a recent report has evaluated the associations between the intake of polyphenol-rich foods (estimated from a 2-d dietary record) and the urinary excretion of several phenolic compounds from spot urine samplesReference Mennen, Sapinho, Ito, Bertrais, Galan, Hercberg and Scalbert86. Here, apple consumption was positively correlated with phloretin, grapefruit consumption with naringenin, orange with hesperetin, citrus fruit consumption with both naringenin and hesperetin (r 0·31–0·57, P < 0·05). The combination of fruits and/or fruit juices was positively correlated with gallic acid and 4-O-methylgallic acid, isorhamnetin, kaempferol, hesperetin, naringenin and phloretin (r 0·24–0·44, P < 0·05). In addition, coffee consumption was positively correlated with caffeic and chlorogenic acids (r 0·29 and 0·63, P < 0·05 respectively) and black tea and wine consumption were positively correlated with gallic and 4-O-methylgallic acids (r 0·37–0·54, P < 0·001). This study suggests that several polyphenols measured in a spot urine sample can be used as biomarkers of polyphenol-rich food intakeReference Mennen, Sapinho, Ito, Bertrais, Galan, Hercberg and Scalbert86.
Conclusion
It is clear that the choice of biomarker for the estimation of polyphenol intake is complex with different classes of flavonoids and possibly even individual polyphenols requiring different approaches. For the majority of flavonoids, which possess short plasma half-lives, measurement of plasma concentrations will provide little information regarding even acute intakes and quantification of their output in urine may be a better approach. In addition to scientific considerations, practical issues may determine the choice of method. The quantification of a number of metabolites in 24 h urine samples may be a suitable approach in small-scale human intervention trials but may not be a realistic possibility in large-scale epidemiological studies. This is due to problems in organising the collection of 24 h urine samples from a large study population. An intermediate approach may be the quantification of metabolites in spot urine samples but there is little evidence on which to assess the suitability of this approach and thus it should be highlighted as an important area for future research.
This review has focussed on the identification and validation of individual polyphenols, or their metabolites, that may represent useful biomarkers of the intake of polyphenols in human subjects. The majority of potential biomarkers are those deriving from phase I and II metabolism of flavonoids and other polyphenols in the small intestine and liver. Metabolites such as glucuronide and sulphate conjugates (and related O-methylated forms) are commonly used to determine the extent of polyphenol absorption in human subjects and represent excellent biomarkers providing researchers employ careful analytical techniques. In addition to these well characterised metabolic forms, there is great potential for cellular metabolites of polyphenols, such as glutathionyl and cysteinyl conjugatesReference Spencer, Abd-El-Mohsen and Rice-Evans87, Reference Spencer, Kuhnle, Williams and Rice-Evans88, to act as specific biomarkers of polyphenol intake, as they are likely to be present in significant amounts in both blood and urine. There are also a number of other candidates, such as valerolactones for flavanol intakeReference Lee, Maliakal, Chen, Meng, Bondoc, Prabhu, Lambert, Mohr and Yang57, isoferulic acid for chlorogenic acid ingestionReference Rechner, Spencer, Kuhnle, Hahn and Rice-Evans32 and homovanillic alcohol for the intake of hydroxytyrosolReference Corona, Tzounis, Assunta, Deiana, Debnam, Visioli and Spencer89. In summary, quantification of specific flavonoid and polyphenol biomarkers in accessible fluid/tissues holds great potential as an alternative approach to traditional dietary assessment techniques and will undoubtedly provide invaluable information regarding the intake of polyphenols in human subjects.
Acknowledgements
We kindly acknowledge The European Nutrigenomics Organisation (NuGo), for the support to write this review. (The European Nutrigenomics Organisation: ‘linking genomics, nutrition and health research’ (NuGo, CT-2004-505944) a Network of Excellence funded by the European Commission's Research Directorate General under Priority Thematic Area 5, Food Quality and Safety Priority, of the Sixth Framework programme for Research and Technological Development.) All authors are NuGo members. Further information on NuGo activities can be found at http://www.nugo.org. Dr Spencer is also sponsored by the Biotechnology and Biological Sciences Research Council (BB/C518222/1) and the Medical Research Council (G0400278/NI02).