Introduction
Toxocara canis is a zoonotic nematode and one of the most frequent parasites worldwide, affecting predominantly young dogs under 1 year of age (Barutzki & Schaper, Reference Barutzki and Schaper2013). In Europe, the prevalence in dogs varies between 3.5% and 34% (Overgaauw & van Knapen, Reference Overgaauw and van Knapen2013). Among risk factors for infection of dogs can be age, coprophagy, unsupervised access to outdoor environment and periodical placement in a kennel (Nijsse et al., Reference Nijsse, Ploeger, Wagenaar and Mughini-Gras2015).
Dogs can be infected through vertical transmission (transplacental and transmammary), but also through ingestion of embryonated eggs from the environment or by consuming paratenic hosts with viable larvae of T. canis (Schnieder et al., Reference Schnieder, Laabs and Welz2011). Parasites develop adult stages in the intestinal tract of dogs causing mostly digestive symptoms, but infections may be asymptomatic (Overgaauw & van Knapen, Reference Overgaauw and van Knapen2013). Nevertheless, one adult female of T. canis can lay up to 200,000 unembryonated eggs per day, which are then shed in faeces into the environment. Taking in consideration the cases of high infestation in some dogs, this number can rise up to millions of eggs per day (Glickman & Shofer, Reference Glickman and Shofer1987). Egg embryonation begins in the environment, under proper temperature and humidity, and it takes between 2 and 5 weeks for the larvae to reach infective stage (Despommier, Reference Despommier2003; Gamboa, Reference Gamboa2005). The structure of the eggs provides high resistance to different chemicals and even against high temperature variations for up to 12 months (Bouchet et al., Reference Bouchet, Boulard, Baccain and Leger1986; Azam et al., Reference Azam, Ukpai, Said, Abd-Allah and Morgan2012).
Humans and other paratenic hosts become infected after ingestion of embryonated eggs with second- (Morrondo et al., Reference Morrondo, Díez-Morrondo, Pedreira, Díez-Baños, Sánchez-Andrade, Paz-Silva and Díez-Baños2006) or third-stage larvae (Kolbeková et al., Reference Kolbeková, Větvička, Svoboda, Skírnisson, Leissová, Syrůček and Kolářová2011). In this case, larvae stop development but they migrate via the bloodstream to different organs and tissues where they remain viable for longer time periods (Schnieder et al., Reference Schnieder, Laabs and Welz2011). Nijsse et al. (Reference Nijsse, Ploeger, Wagenaar and Mughini-Gras2015) consider that one of the most important infection sources is contaminated soil or artificial surfaces (paved roads, sidewalks, floors, etc.). Consumption of raw meat containing somatic larvae, contaminated vegetables and water is also considered a major risk (Overgaauw & Nederland, Reference Overgaauw and Nederland1997; Taira et al., Reference Taira, Saeed, Permin and Kapel2004; Lee et al., Reference Lee, Kim, Oh, Kim, Kim, Jeon and Byun2010).
Human toxocariasis can have four major forms: ‘visceral larva migrans’, ‘ocular larva migrans’, neurotoxocariasis (neural larva migrans) and covert toxocariasis (Fan et al., Reference Fan, Holland, Loxton and Barghouth2015). During the last decades, the number of companion animals has significantly increased, and therefore so has the possibility of transmission of various zoonotic agents (Paul et al., Reference Paul, King and Carlin2010). According to the Centers for Control Disease and Prevention, from a public health perspective, human toxocariasis remains a major and often neglected zoonotic disease, especially among children, but also for certain occupational categories such as veterinarians and staff working in clinics, hospitals, shelters and owners. Owing to the high risk of T. canis infection in dogs, humans and other paratenic hosts, there is a permanent interest in evaluating and finding chemicals or other preventive measures that can inactivate the infective form of the parasite (Morrondo et al., Reference Morrondo, Díez-Morrondo, Pedreira, Díez-Baños, Sánchez-Andrade, Paz-Silva and Díez-Baños2006; Araujo et al., Reference Araujo, Araújo, Braga, Ferreira and Tavela2013; Magaña-López et al., Reference Magaña-López, Luna-Pabello, Barrera-Godínez, de Velásquez and Fernández-Villagómez2016; Von Dohlen et al., Reference von Dohlen, Houk-Miles, Zajac and Lindsay2017). In this context, routine evaluation of the efficient disinfectants in high-risk environments becomes an important task, from both a veterinary and public health perspective. The objective of this study was to evaluate the effect of six disinfectant products commonly used in kennels, veterinary clinics and as household cleaning products on the embryogenesis and viability of T. canis eggs.
Material and methods
Preparation of T. canis eggs
Live adult parasites of T. canis were obtained from seven naturally infected freshly dead puppies at necropsy. The animals died due to other causes, and the necropsy was done in 30–45 min. Female T. canis (n = 18) were identified, separated and rigorously washed with saline water. The uteri were isolated and both anterior non-bifurcated portions were dissected under magnification (4×) (Oshima, Reference Oshima1961; Alcântara-Neves et al., Reference Alcântara-Neves, Barbosa dos Santos, Mendonca, Figueiredo and Pontes-de-Carvalho2008). Eggs were collected from the uteri and suspended in distilled water in Petri dishes. The exposure of eggs to disinfectants occurred in the same day.
Egg exposure to disinfectants and cultivation
A total of six disinfectant products recommended for their bactericidal and viricidal properties and commonly used in high-risk areas (i.e. veterinary clinics, hospitals, kennels and shelters) were tested. Even if manufacturers do not specify antiparasitic effects on the label, some veterinarians assume (off-label) that the biocidal effect also includes parasites. The compositions and concentrations of the tested products were (A) 4.5% sodium hypochlorite; (B) mix of 9.9% N-(3-aminopropyl)-N-dodecylpropan-1.3-diamine and 6% didecyldimethylammonium chloride; (C) 77.14% sodium dichloroisocyanurate dehydrate; (D) mix of 10–30% glutaraldehyde and 5–10% quaternary ammonium compounds, benzyl-c12-18- alkyldimethyl, chlorides; (E) mix of 20% 2-propanol, 10% ethanol, 0.2% benzalkonium chloride, 0.01% glucoprotamin; (F) mix of 40–55% pentapotassium bis (peroxymonosulphate) bis (sulphate), 10–12% sodium C10-13-alkylbenzenesulphonate, 7–10% malic acid, 4–6% sulphamidic acid, 1–5% sodium toluenesulphonate, <3% dipotassium peroxodisulphate and <0.25% dipentene; (G) control group, represented by eggs solely exposed to distilled water.
For testing, the commercial products were diluted to maximal concentration recommended by the manufacturer for a biocidal effect: A – 2.4%, B – 1%, C – 0.05%, D – 0.06%, E – 95%, F – 1%. From each diluted product samples of 1.9 ml were mixed in sterile tubes with 0.1-ml aliquots containing 10,000 T. canis eggs in a total volume of 2 ml. In order to mimic the real-life situations (daily disinfections) that occur in veterinary clinics, five different contact times were applied for each mixture: 5, 10, 15, 30 and 60 min, at a constant temperature of 25 °C.
Permanent stirring was provided to avoid egg sedimentation. After exposure, mixtures were centrifuged for 5 min at 1015 RCF (relative centrifugal force). The supernatant was removed and the egg sediment was washed twice with distilled water and twice centrifuged for 5 min at 1015 RCF. Three replicates were tested for each diluted disinfectant and for each contact time. Each procedure was also repeated for the control group. All samples were transferred to 12 multiwell culture plates, each containing 3 ml of distilled water, and incubated for 2 weeks at 27 °C. Daily oxygenation and 10/14 h light/darkness cycles were provided.
Larval development assay/disinfectant efficacy
Egg morphology and larval motility were assessed by daily examination of 20 µl of each sample under light microscopy (10×, 20×, 30×, 40×). As eggs may become infective in 2 weeks under controlled temperature, all statistical analyses were set at this time point (Gamboa, Reference Gamboa2005). The percentage of larval development was calculated applying the formula: % larval development = number of fully embryonated eggs/total number of eggs × 100 (Oh et al., Reference Oh, Kim, Ahn and Shin2016). Degenerated eggs were not counted.
For every disinfectant tested, a mean of three samples of 50 µl of each replicate was used in obtaining the larval development percentage. For the control group, a mean of all samples was calculated in the same manner. Effect on embryogenesis was expressed by subtracting the larval development percentage from 100.
Statistical analyses
The statistical software package GraphPad© InStat was used to perform the statistical evaluations using one-way analysis of variance. The comparison was made by using the Tukey–Kramer multiple comparisons test. The significance level for all statistical tests was predetermined at P < 0.05.
Results
The efficacy of each disinfectant product, for all exposure times, is shown in table 1 as the arithmetic mean of the three replicates. In all samples, embryonation of T. canis started on the second day of incubation. Fully embryonated eggs were observed starting at day 7 post exposure. All samples contained L2 motile T. canis larvae on day 14. Regardless of the contact time, none of the tested disinfectants succeeded in completely stopping embryonation nor in destroying all eggs. Statistical analysis of egg embryonation inhibition did not reveal any significance, regardless of the disinfectant used or contact time. Comparing the results between disinfectants, the probability factor was without statistical significance (P > 0.05). Results proved that product C, containing sodium dichloroisocyanurate dehydrate, with a 30-min contact time, was the most efficient (31.44%). Morphological changes, consisting of decorticated eggs with a more transparent outer layer, were detected for product A, containing sodium hypochlorite (fig. 1). Furthermore, for this product, egg development was faster than the control group.
Table 1. Efficacy of disinfectants for each exposure time after 2 weeks of incubation (replicates arithmetic mean).

LD, larval development; E, efficacy; SD, standard deviation.
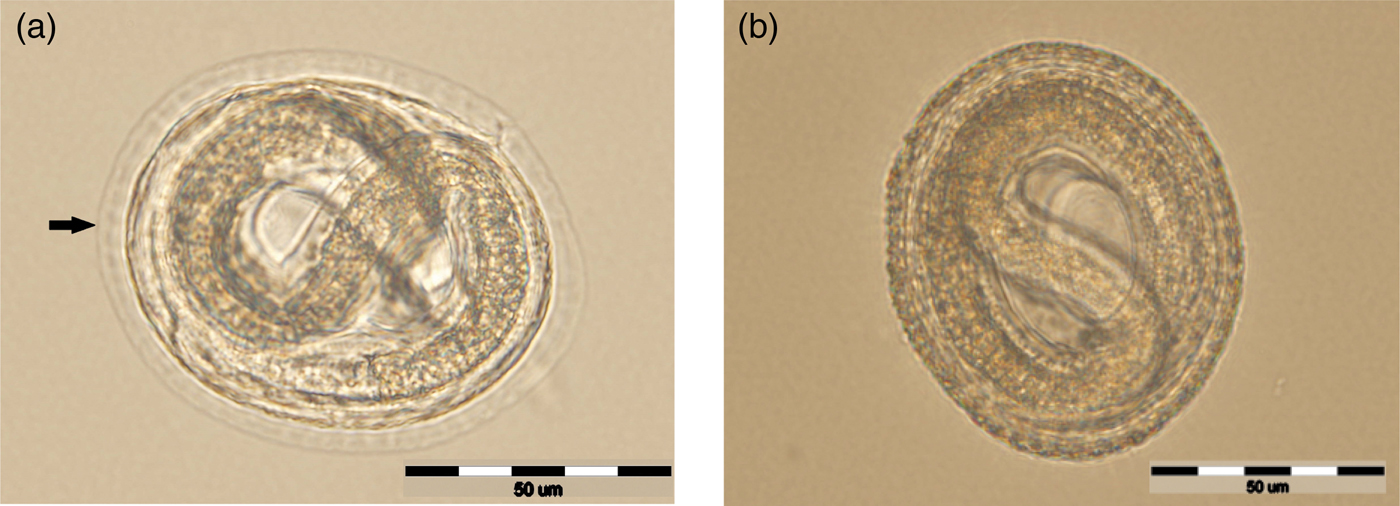
Fig. 1. Egg exposed to sodium hypochlorite with changes in the eggshell (arrow), but with a normal larval morphology (a) compared with untreated egg with an intact outer layer (b).
Discussion
Hygiene and preventive measures for biological risks in veterinary clinics or hospitals, veterinary diagnostic laboratories, shelters and kennels include disinfection against bacterial, viral and fungal agents. Nevertheless, surfaces, soil and dog playgrounds are also potential sources of parasitic infections for both humans and animals. In the present study, six routinely used disinfectants were tested at different exposure times in order to assess their effect on embryogenesis of T. canis eggs. The results of the study revealed that preventive measures based on common disinfectants against other various pathogens are inefficient against T. canis eggs, with the zoonotic risk still present. None of the chemicals used, regardless of the contact time, were able to destroy all eggs, nor to completely stop their development to infective stages. Shorter or prolonged contact time had no influence on egg development inhibition.
Studies concerning the efficacy of various disinfectants against T. canis eggs concluded in different, often contrasting, results. Sodium hypochlorite is frequently used due to its availability and efficacy against different microorganisms. Morrondo et al. (Reference Morrondo, Díez-Morrondo, Pedreira, Díez-Baños, Sánchez-Andrade, Paz-Silva and Díez-Baños2006) reported that, when kept for 15 days in 2% sodium hypochlorite, only 2% of T. canis eggs reach the L2 stage, and if contact time is prolonged to 24 days, L2 stage egg development reaches 8%. Mice infection with these L2 stage eggs did not reveal migratory capacities in the nervous system. Verocai et al. (Reference Verocai, Tavares, De Ribeiro, Correia and Scott2010) a noticed low embryonation percentage when using 2–2.5% sodium hypochlorite. The same authors also noticed total egg degeneration when 70% ethanol was used. Variability of scientific data concerning disinfectant efficacy can be explained by the use of different testing methods: incubation within the diluted disinfectant for a certain time period or using different contact times followed by removal of chemicals before incubation. Von Dohlen et al. (Reference von Dohlen, Houk-Miles, Zajac and Lindsay2017) found results similar to the current study, noting that the use of sodium hypochlorite was not sufficient to inhibit T. canis eggs embryogenesis at different contact times (15–120 min). Moreover, the same authors reported that sodium hypochlorite solution induced morphological changes of the egg shell and faster embryonation, emphasizing the possibility of a more intense egg oxygenation. Disinfectants containing benzalkonium chloride and glutaraldehyde proved to be ineffective in inhibiting T. canis larval development (Verocai et al., Reference Verocai, Tavares, De Ribeiro, Correia and Scott2010).
The disinfectant containing a mix of pentapotassium, sulphamidic acid, sodium dodecylbenzenesulphonate and dipotassium peroxodisulphate (product F) has never been tested on T. canis eggs before. The product was only tested on T. leonina and proved effective in stopping larval development (El-Dakhly et al., Reference El-Dakhly, Aboshinaf, Arafa, Mahrous, El-Nahass, Gharib and Craig2018). Sodium hypochlorite, in concentrations of 0.2% and 0.02%, showed no efficacy in inhibiting Ascaris suum egg development (Oh et al., Reference Oh, Kim, Ahn and Shin2016). Oh et al. (Reference Oh, Kim, Ahn and Shin2016) obtained the same results for ethanol, methanol and chlorhexidine.
Different concentrations of iodine (2.5%, 5%, 7.5% and 10%) did not induce morphological changes in eggs of T. canis, but succeeded in inhibiting larvae motility in vitro and migratory capacities in the brains of infected mice, whereas eggs treated with 10% glutaraldehyde, 3% phenol, 7% sodium hypochlorite, 10% benzalkonium chloride, 1% potassium permanganate or 70% ethyl alcohol solutions still showed larval motility (Ayçiçek et al., Reference Ayçiçek, Yarsan, Sarimehmetoğlu, Tanyüksel, Girginkardeşler and Özyurt2001). Povidone iodine solutions only managed to inactivate the majority of A. suum eggs after 5 min of exposure at a concentration of 10%, but it did not completely destroy all eggs (Oh et al., Reference Oh, Kim, Ahn and Shin2016).
The tested exposure times were chosen to mimic the real-life situations that occur in veterinary clinics during daily disinfections. The difference between this study and other studies on disinfectant efficacy is that the majority of cited authors tested the disinfectants by keeping them for several days in contact with the eggs.
Further and more complex studies are required in order to assess efficient methods to completely destroy or inhibit T. canis embryogenesis and prevent animal and human contamination, especially in areas with high biological risk. The disinfectants chosen in this study were used off label for decontaminant purposes, but they are frequently used for disinfecting potentially contaminated areas in veterinary clinics, hospitals, laboratories, kennels and shelters or as household products.
In conclusion, current measures of disinfection used in places with high risk of contamination are not sufficient for infective stages of T. canis; therefore, the persistence of zoonotic and animal-to-animal infection hazards still remains. Further studies on other active compounds, new combinations and concentrations, optimal exposure times or even new disinfectants are required in order to completely inactivate T. canis eggs or inhibit embryogenesis.
Author ORCIDs
M. Cernea, 0000-0001-5829-1638
Acknowledgements
This project was funded by the Romanian Ministry of Research and Innovation through Program 1 – Development of the National Research and Development System, Subprogram 1.2 – Institutional Performance – Projects for Financing the Excellence in CDI, Contract no. 37PFE/06.11.2018. Title of the project: Increasing the institutional performance through consolidation and development of research directions within the USAMVCN.
Conflict of interest
None.
Ethical standards
This article does not contain any studies with human participants or animals performed by any of the authors.