Introduction
The genus Echinochloa contains the most troublesome weeds of rice (Oryza sativa L.) production systems worldwide. It comprises about 50 species, most of which are found in the tropics (Michael Reference Michael1983). Echinochloa spp. are highly adaptive and competitive due to traits such as high fecundity, seed dormancy, and genetic diversity (Lopez-Martinez et al. Reference Lopez-Martinez, Salva, Finch, Marshall and De Prado1999; Maun and Barrett 1986). Barnyardgrass [Echinochloa crus-galli (L.) P. Beauv.] and junglerice [Echinochloa colona (L.) Link] are the two most problematic Echinochloa species infesting rice fields in the southern United States (Bryson and Reddy Reference Bryson and Reddy2012). Echinochloa crus-galli is an annual grass weed that mimics rice, especially at the seedling stage. However, by the time it can be easily differentiated from rice, crop yield loss may have already occurred (Holm et al. Reference Holm, Plucknett, Pancho and Herberger1977). It is an erect-growing plant, reaching up to 2 m tall. The leaves are linear to lanceolate, extending up to 40-cm long and 0.5- to 1.5-cm wide (Chin Reference Chin2001). Echinochloa colona, a species like E. crus-galli in appearance, is also an annual grass weed, but has a prostrate growth habit, with plants growing up to 0.6-m tall (Hruševar et al. Reference Hruševar, Mitić, Sandev and Alegro2015). In general, E. colona has lanceolate leaves with green or purple stems that are often branched at the base (Zimdahl et al. Reference Zimdahl, Lubigan, Moody and Mabbayad1989). Rough barnyardgrass [Echinochloa muricata (P. Beauv.) Fernald], another species found in U.S. rice production, often grows erect (0.8- to 1.6-m tall), with stem nodes sometimes having sparse hairs (Gould et al. Reference Gould, Ali and Fairbrothers1972). Echinochloa colona and E. crus-galli can flower throughout the year, whereas E. muricata usually flowers during midsummer to early fall (Chauhan and Johnson Reference Chauhan and Johnson2009; Tahir Reference Tahir2016; Vengris et al. Reference Vengris, Kacperska-Palacz and Livingston1966).
The taxonomy of the genus Echinochloa is very complex due to continuous morphological variation exhibited by the taxa and the formation of several intergrading polymorphic complexes, which are difficult to classify (Barrett and Wilson Reference Barrett and Wilson1981). In many cases, Echinochloa spp. lack conspicuous identification characteristics, which can lead to misidentification (Costea and Tardif Reference Costea and Tardif2002; Michael Reference Michael1983). Although Echinochloa species show a high degree of autogamy, occasional outcrossing is sufficient to facilitate gene exchange among populations (Maun and Barrett 1986). In rice production in Texas, E. crus-galli has long been thought to be the most prominent species of Echinochloa (popularly known as “barnyard” among growers and crop consultants in the region). However, visits to Texas rice fields by the authors have suggested the distribution of more E. colona populations than what was previously perceived.
In U.S. rice production, different Echinochloa spp. were reported to have evolved resistance to various herbicides (Heap Reference Heap2021), and multiple herbicide resistance is also common (Rouse et al. Reference Rouse, Roma-Burgos, Norsworthy, Tseng, Starkey and Scott2018). Herbicide resistance is often associated with certain plant morphological characteristics. For example, in a detailed survey of Echinochloa in rice fields in northern Greece, Damalas et al. (Reference Damalas, Dhima and Eleftherohorinos2006) found significant morphological differences among the populations, which were consistently linked with the level of sensitivity to different rice herbicides used in the region. Tsuji et al. (Reference Tsuji, Fischer, Yoshino, Roel, Hill and Yamasue2003) compared the morphology of 15 herbicide-resistant and 8 susceptible late watergrass [Echinochloa phyllopogon (Stapf) Kosso-Pol.] populations from rice fields in Sacramento Valley, CA, and found that the resistant populations were shorter in height, with shorter and narrower flag leaves, thinner culms, and smaller spikelets compared with the susceptible plants. In that study, the morphological similarity among the resistant populations was also greater than that of the susceptible populations. Maity et al. (Reference Maity, Singh, Jessup and Bagavathiannan2021) reported positive correlation between herbicide resistance and 100-seed weight as well as seed dormancy in Italian ryegrass [Lolium perenne L. ssp. multiflorum (Lam.) Husnot].
Though Echinochloa spp. are important weeds in rice production in Texas, knowledge of the morphophysiological diversity and species composition of different ecotypes is limited. Moreover, multiple resistance (different combinations) was confirmed for propanil, quinclorac, imazethapyr, and fenoxaprop in several Echinochloa ecotypes collected in Texas (Liu Reference Liu2018), but whether certain morphophysiological characteristics are associated with altered sensitivity to herbicides in these ecotypes is unknown. The objectives of this research were to (1) characterize different Echinochloa ecotypes collected from rice fields in Texas for different morphophysiological traits and (2) determine potential association between plant morphophysiological traits and herbicide-resistance status in the ecotypes studied.
Materials and Methods
Plant Materials
Echinochloa samples were collected across the rice-growing region in Texas, known as the “Texas Rice Belt” (Figure 1), during late July/early August in 2015 and 2016. Surveys followed a semi-stratified methodology, and the survey sites were randomly selected on a Google® map using the ITN Converter software (v. 1.88; Benichou Software, http://www.benichou-software.com) without any prior knowledge of the distribution of Echinochloa spp. in specific fields. More details of the survey methodology can be found in Liu et al. (Reference Liu, Singh, Zhou and Bagavathiannan2020). A total of 232 fields were surveyed, but samples were collected only in fields where the Echinochloa escapes were present. In each survey field, approximately 20 mature panicles were randomly collected and pooled into a single composite sample. A total of 54 samples were collected, representing 54 Echinochloa ecotypes. Samples were subsequently dried in a hot-air oven at 50 C for 48 h, hand threshed, cleaned, and stored in ziplock bags before use in the experiment. Sensitivity of the collected Echinochloa ecotypes to five different herbicides (clomazone, propanil, quinclorac, imazethapyr, and fenoxaprop) commonly used in Texas rice production was evaluated in a parallel study (Liu Reference Liu2018).

Figure 1. Collection sites (dots) of different Echinochloa ecotypes used in the experiment. Field surveys were conducted in all rice-growing counties highlighted in green; however, seeds were available in harvestable quantities only in select fields.
Morphological Characterization
Seedlings of each ecotype were raised at the Norman Borlaug Center for Southern Crop Improvement Greenhouse Research Facility at Texas A&M University, College Station, during March 2017. Seeds were germinated in petri dishes (9-cm diameter) and transplanted to individual pots (10-cm diameter, 9-cm height) at the 1-leaf seedling growth stage. When the seedlings were about 15- to 25-cm tall, they were transplanted in a common garden established at the David Wintermann Rice Research and Extension Center located near Eagle Lake, TX. The soil type at the study location was Hockley silt loam (fine, smectitic, hyperthermic Typic Albaqualfs; 61% sand, 31% silt, and 8% clay), with 0.75% organic matter content and 6.1 pH.
The climate at this location is characterized by mild winters and hot and humid summers, with an average annual rainfall of 114 cm. The experiment included all the 54 Echinochloa ecotypes established in rows (7 plants per row), arranged in a randomized complete block design with four replications (i.e., 4 rows of 7 plants each per ecotype). The distance between plants within a row was 1 m, and the distance between rows was 1.2 m. The experimental area was maintained weed-free throughout the study period by a combination of hand weeding and glyphosate application between rows using a sponge roller at approximately biweekly intervals. The plots were surface irrigated as needed. Rice (variety: ‘Clearfield™ 111’) was planted at 78.4 kg ha−1 all around the plots to provide a safe border. In each row, 3 plants (out of the 7 plants) were randomly selected (avoiding borders) and tagged for detailed morphophysiological characterization.
A total of 14 traits were recorded from each tagged plant: stem angle; stem color; leaf color; leaf texture; flag leaf length, width, and angle; days to flowering; panicle length; plant height at maturity; plant biomass; seed shattering; seed yield; and seed dormancy. Stem angle (growth habit) was determined visually relative to the horizontal plane (1 = prostrate/open canopy; 2 = intermediate; 3 = erect/closed canopy). Flag leaf angle was determined visually relative to the main culm (1 = leaf angle ≤45°; 2 = leaf angle >45°). The length and width (measured at the widest point of the leaf) of flag leaves were measured from five random tillers plant-1. Leaf texture was assessed by rubbing fingers along the surface of three random flag leaves in each plant (1 = smooth; 2 = intermediate; 3 = rough). Plant height was measured from the base of the plant to the tip of the panicle on the main stem. Plants were monitored weekly for initiation of flowering. The length of five randomly selected panicles (fully developed) was measured for each plant. To collect any shattered seed, the panicles of each plant were enclosed in Delnet® bags (Delstar Technologies, Middletown, DE, USA) before seed maturity. Plants were harvested at maturity by clipping the entire plant from the base. Samples were dried and weighed for plant biomass determination. Seeds were threshed and weighed for estimation of seed yield. Taxonomical classification of each Echinochloa ecotype was carried out based on Carretero (Reference Carretero1981).
Evaluation of Seed Dormancy
Seed samples were stored at room temperature (25 C) after harvest. Seed germination tests were carried out for all Echinochloa ecotypes at 210 d after harvest. Twenty-five seeds of each ecotype were placed on a moistened filter paper in a petri dish (9-cm diameter) and incubated at 30 C with a light intensity of 90 µmol m–2 s-1. The test included two replications and two experimental runs for each ecotype. Petri dishes were arranged in a completely randomized design. Seedlings were counted and removed every 4 d, up to 12 d. The petri dishes were re-randomized after each evaluation timing. Viability of nongerminated seeds was determined using the tetrazolium test (Overaa Reference Overaa1984). Seed were immersed in 2, 3, 5-triphenyltetrazolium chloride (1%) staining solution and incubated at 30 C for 24 h before being examined. Seeds with pink or red embryos were considered alive and dormant.
Data Analysis
Statistical analyses were performed using JMP® Pro (v. 13.1, SAS Institute, Cary, NC, USA). A principal component analysis (PCA) was conducted using JMP® Pro based on the 14 morphophysiological variables measured in this study to determine the most important traits that contribute to grouping of ecotypes based on eigenvalues. From the PCA output, four variables were chosen as principal traits for K-means clustering to group the Echinochloa ecotypes. The number of clusters was determined based on the fit statistic with the largest cubic clustering criterion value, using JMP® Pro. The resistance status of each ecotype for the herbicides clomazone, propanil, quinclorac, imazethapyr, and fenoxaprop (screening methodology and results are described in Liu [Reference Liu2018]) was used to calculate the percentage of ecotypes resistant to one or more herbicide sites of action (SOAs) within each hierarchical cluster. A multiple resistance (MR) status number was assigned to each ecotype representing the total SOAs to which each ecotype showed resistance (MR = 0, susceptible ecotype; MR = 1, resistant to one SOA; etc.). A Pearson correlation analysis between the herbicide-resistance status and the 14 morphophysiological traits measured was performed in JMP® Pro.
Results and Discussion
Species Composition
Based on the taxonomic characteristics of the plants, 52 of the total 54 ecotypes studied were identified as E. colona and 1 each as E. crus-galli and E. muricata (Table 1; Figure 2). Results show that E. colona is the most prevalent Echinochloa species infesting rice fields in Texas. Similar findings have been reported by Bryson and Reddy (Reference Bryson and Reddy2012) in a study involving 240 Echinochloa accessions collected from six midsouthern U.S. states (Alabama, Arkansas, Kentucky, Louisiana, Mississippi, and Tennessee), where E. colona was the most commonly found species throughout the region. Likewise, in eastern Arkansas, 73 of the 94 accessions of Echinochloa collected from rice and soybean [Glycine max (L.) Merr.] fields were identified as E. colona (Tahir Reference Tahir2016). The rest of the samples included E. crus-galli, E. muricata, and coast cockspurgrass [Echinochloa walteri (Pursh) A. Heller]. In the present survey, E. walteri was not observed in rice fields in Texas. Tahir (Reference Tahir2016) indicated that high fecundity and low seed dormancy of E. colona have likely contributed to its widespread occurrence in the region.
Table 1. Details of the Echinochloa ecotypes collected in rice production fields in Texas.


Figure 2. Photos of three Echinochloa ecotypes: (A) E. colona; (B) E. crus-galli; (C) E. muricata.
Phenotypic Differentiation within and among Echinochloa Ecotypes
About half (48%) of the E. colona ecotypes showed prostrate or intermediate growth habit with short plants (average height: 48 cm), whereas E. crus-galli (127 cm) and E. muricata (137 cm) exhibited erect growth habit (Table 2; Figure 2). Our results corroborate the observations of Tahir (Reference Tahir2016) on Echinochloa ecotypes characterized in Arkansas with respect to species characteristics. Prostrate growth habit allows a plant to have maximum ground coverage with open canopy, whereas an erect growth habit with closed canopy helps avoid shading from nearby plants. Rice breeders intentionally select for an erect plant growth characteristic to improve production efficiency (Monsi et al. Reference Monsi, Uchijima and Oikawa1973). The erect growth habit of E. crus-galli is one of the examples of crop mimicry in weed species (Barrett Reference Barrett1983). Moreover, plants tend to exhibit erect growth habit when they grow in a dense population (van Hinsberg and van Tienderen Reference van Hinsberg and van Tienderen1997). Observations from our field survey showed that E. colona plants were like rice plants in height, though E. crus-galli and E. muricata were much taller than the rice canopy.
Table 2. Morphophysiological characteristics of different Echinochloa spp. collected in rice production fields in Texas.
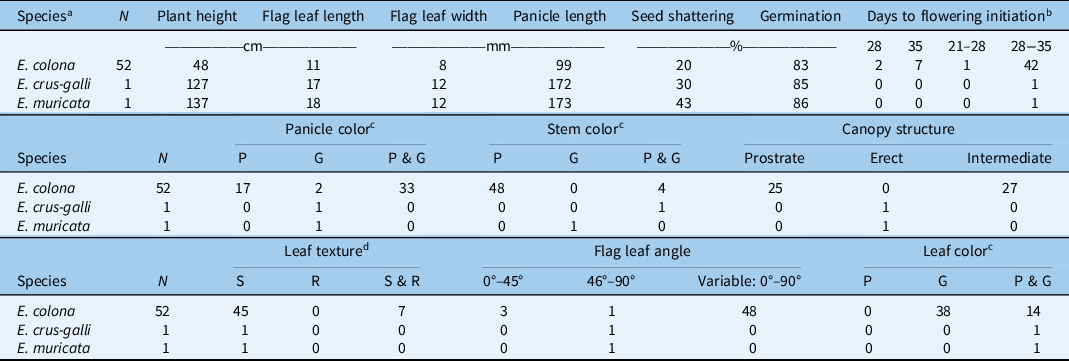
a Species identified based on taxonomic classification.
b Measured as days after transplanting; the range indicates intra-ecotype variability in initiation of first flowering.
c Color: P, all plants within the ecotype exhibiting purple color; G, all plants within the ecotype exhibiting green color; P & G, plants within a given ecotype with both plants of purple color and plants of green color.
d Leaf texture: S, smooth; R, rough; S & R, both smooth and rough.
The majority (73%) of E. colona ecotypes had green color leaves, while the rest (27%) had a mixture of plants with purple or green color leaves within the same ecotype (Table 2). The E. crus-galli and E. muricata ecotypes characterized here also had a mixture of plants with either purple or green color leaves within each ecotype (Table 2). It is likely that ecotypes showing a mixture of phenotypic traits might have resulted from inter/intraspecific hybridization (Burgos et al. Reference Burgos, Singh, Tseng, Black, Young, Huang, Hyma, Gealy and Caicedo2014; Singh et al. Reference Singh, Singh, Black, Boyett, Basu, Gealy, Gbur, Pereira, Scott, Caicedo and Burgos2017). It is often observed in the field that the leaf blades of E. colona have purple marks; however, this is not a reliable key for species identification (Bryson and Reddy Reference Bryson and Reddy2012). Tahir (Reference Tahir2016) indicated that E. muricata was the only species that has green leaves with purple margins, which may help distinguish this species from E. crus-galli. This trait was not observed in the E. muricata ecotype studied here. With respect to stem color, 92% of the E. colona ecotypes had purple stems, and the rest had a mixture of plants with purple or green stems. Echinochloa muricata had green-based stems, whereas E. crus-galli had plants with both green- or purple-based stems. Tabacchi et al. (Reference Tabacchi, Mantegazza, Spada and Ferrero2006) reported in an Italian rice system that basal stem color was an important morphological trait for distinguishing different Echinochloa accessions. The ecological advantage of stem color, however, is not clear.
Nearly 87% of the E. colona ecotypes had smooth (hairless) leaf texture, and the rest had a mixture of individuals with both smooth and rough-textured leaves; E. crus-galli and E. muricata had smooth leaves. This finding is largely consistent with the report of Holm et al. (Reference Holm, Plucknett, Pancho and Herberger1977) on Echinochloa spp. collected from the Mississippi delta region of Arkansas, where E. colona, E. crus-galli, and E. muricata all had hairless leaf blades. They did indicate that E. muricata may have some long hairs at the base of the leaf blades, but this characteristic was not observed in the current study. Leaf hairs may directly influence the retention and uptake of herbicides (Himel et al. Reference Himel, Loats, Bailey and Cheng1990). For instance, Sterling and Jochem (Reference Sterling and Jochem1999) found that the white locoweed (Oxytropis sericea Nutt.), which has fine hairs on the leaf surface, exhibited high uptake of the herbicide metsulfuron.
The average flag leaf length of E. colona was 11 cm (range: 5.3 to 17 cm), considerably shorter than that of E. crus-galli (17 cm) and E. muricata (18 cm) (Table 2). Likewise, E. colona had narrower (8-mm wide) flag leaves than E. crus-galli (12-mm wide) or E. muricata (12-mm wide). The surface area of a flag leaf is associated with photosynthetic capacity (Damalas et al. Reference Damalas, Dhima and Eleftherohorinos2008; Evans and Rawson Reference Evans and Rawson1970). High flag leaf surface area allows the plant to intercept more sunlight for photosynthesis and assimilate carbon. The longer and wider flag leaves of E. crus-galli and E. muricata could produce sufficient energy to support the taller plant stature, compared with E. colona. With respect to flag leaf angle, erect angles (<45°) in grasses allow for more light penetration to the lower parts of the plant canopy and improve overall light interception by the plant (Mantilla-Perez and Salas Fernandez Reference Mantilla-Perez and Salas Fernandez2017). In fact, self-shading rather than leaf angle per se is important for light interception and carbon gain (Falster and Westoby Reference Falster and Westoby2003). In the current study, the majority (48 out of 52, 92%) of E. colona ecotypes exhibited high within-ecotype variation (0° to 90°) for flag leaf angle. Considering that E. colona largely mimics rice crop height, the within-ecotype variability for leaf angle might allow it to maximize light and carbon capture depending on crop density. In contrast, E. crus-galli and E. muricata had flag leaves with much wider angles (46° to 90°). Given that these two species grew taller than the rice canopy, the broader flag leaves and wider flag leaf angles may have provided better light interception, while shading the rice crop underneath (Weiner and Thomas Reference Weiner and Thomas1986).
The majority (95%) of E. colona began flowering within 28 to 35 d after transplanting, and flowering continued for several weeks. Both E. crus-galli and E. muricata also initiated flowering within the same time frame as the majority of E. colona. Reports indicate that E. colona and E. crus-galli can flower throughout the year (Chauhan and Johnson Reference Chauhan and Johnson2009; Vengris et al. Reference Vengris, Kacperska-Palacz and Livingston1966), whereas E. muricata usually flowers during midsummer to early fall (Tahir Reference Tahir2016). A long flowering window increases opportunities for outcrossing among different Echinochloa biotypes and species. Even though members of Echinochloa are autogamous and predominantly self-pollinating, occasional outcrossing could still happen (Maun and Barrett 1986). Pollen-mediated gene flow (PMGF) was detected among E. crus-galli plants at the maximum tested distance of 50 m (Bagavathiannan and Norsworthy Reference Bagavathiannan and Norsworthy2014). An extended flowering window has implications for the spread of herbicide resistance through PMGF across fields.
With respect to panicle length, E. colona had the shortest (9.9 cm) panicles compared with E. crus-galli (17.2 cm) and E. muricata (17.3 cm). It was reported that the inflorescence length and seed production are positively correlated in E. crus-galli (Norris Reference Norris1992). Two different panicle colors are usually observed in Echinochloa: purple and green. The majority (63%) of E. colona had a mix of both purple- and green-colored panicles within the ecotype (Table 2), whereas the remaining 33% or 4% of the ecotypes had purple- or green-colored panicles, respectively. Both E. crus-galli and E. muricata had green-colored panicles. Upon maturity, the caryopsis of the green panicles typically turned brown, while the purple-colored panicles turned dark brown.
Seed shattering is an important weedy trait that contributes to species’ persistence in the field. Results showed that E. crus-galli and E. muricata had greater seed-shattering abilities with 30% and 43% at plant maturity, respectively, compared with E. colona (20%). When tested at 7 mo after harvest, the vast majority of the ecotypes had already overcome dormancy, exhibiting 83%, 85%, and 86% germination for E. colona, E. crus-galli, and E. muricata, respectively. Honěk and Martinková (Reference Honěk and Martinková1996) reported that freshly harvested seeds of Echinochloa spp. typically exhibit dormancy, the length of which can vary from 3 to 7 mo. Seed dormancy is an important bet-hedging mechanism that facilitates temporal dispersal of a species, allowing for persistence under unpredictable environmental conditions (Baskin and Baskin Reference Baskin and Baskin2004). Unfortunately, seed dormancy of the freshly harvested seed could not be tested in the present study due to practical circumstances. Nevertheless, tetrazolium test results suggest that the vast majority of the seed might germinate in the following season should germination requirements be fulfilled. Animal grazing during the rotational year typically practiced in the region might be beneficial for the management of Echinochloa spp.
Clustering of Ecotypes Based on Four Significant Phenotypic Traits
The PCA (Figure 3) indicated that 4 of the 14 phenological traits characterized in the study— plant height, flag leaf length, seed shattering, and seed germination—contributed significantly to the overall morphological diversity of Echinochloa spp. The frequency distribution of the four traits that significantly contributed to Echinochloa ecotype diversity is displayed in Figure 4. Based on these four traits, K-means cluster analysis grouped the 54 Echinochloa ecotypes into five clusters (Table 3; Figure 5).

Figure 3. Principal component analysis showing the traits (plant height, flag leaf length, seed shattering, and seed germination) that significantly contributed to the overall morphological diversity of Echinochloa ecotypes investigated in this study.

Figure 4. Histogram for the four important traits identified by the principal component analysis among the Echinochloa ecotypes collected from Texas rice fields: (A) plant height, (B) flag leaf length, (C) seed shattering, and (D) seed germination.
Table 3. K-means cluster analysis of different Echinochloa ecotypes based on the four most discriminating traits. a
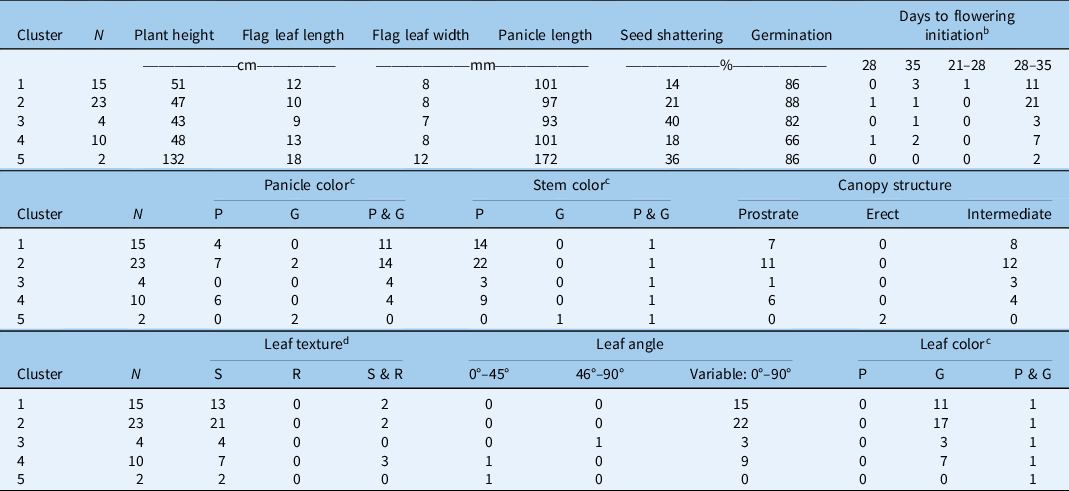
a Clusters based on plant height, flag leaf length, seed shattering, and seed germination.
b Measured as days after transplanting; the range indicates intra-ecotype variability in initiation of first flowering.
c Color: P, all plants within the ecotype exhibiting purple color; G, all plants within the ecotype exhibiting green color; P & G, plants within a given ecotype with both plants of purple color and plants of green color.
d Leaf texture: S, smooth; R, rough; S & R, smooth and rough.

Figure 5. Hierarchical cluster analysis for the 54 Echinochloa ecotypes, along with the herbicide-resistance status for the ecotypes grouped within each cluster shown in pie charts. Green indicates proportion of susceptible ecotypes within the cluster, whereas other colors indicate resistance to one or more herbicide sites of action (SOAs).
Cluster 1 comprised 28% of the characterized ecotypes, with an average plant height of 51 cm, slightly taller than the plants in the Cluster 2 (47 cm), and an average flag leaf length of 12 cm. Seed-shattering potential was the lowest (14%) in this cluster, and seed germination was 86%. Seed shattering is an important weedy trait that typically favors dispersal and persistence of a weed population through seedbank replenishment (Holm et al. Reference Holm, Plucknett, Pancho and Herberger1977). Low weed seed shattering ability of certain ecotypes can be exploited for harvest weed seed control strategies, which rely on the ability of weeds to retain seeds at the time of harvest for seed collection and destruction (Walsh et al. Reference Walsh, Broster, Schwartz-Lazaro, Norsworthy, Davis, Tidemann, Beckie, Lyon, Soni, Neve and Bagavathiannan2018). Cluster 2, which represented the highest number of ecotypes (43%), was characterized by the highest germination capacity (88%). It also had purple- or green-colored panicles.
Cluster 3 consisted of 7% of the total ecotypes, having the shortest (9 cm) flag leaf length and the greatest (40%) seed shattering. The plants in this cluster were also the shortest (43 cm) compared with other groups. Cluster 4 consisted of 18% of the ecotypes characterized. This cluster had the lowest germination rate (66%). This is notable, because plants in other clusters showed very high germination. Low germination could be attributed to seed dormancy, which is regulated by genetic and environmental factors (Bewley Reference Bewley1997; Chauhan and Johnson Reference Chauhan and Johnson2009). As discussed earlier, seed dormancy allows for species’ persistence (Baskin and Baskin Reference Baskin and Baskin2004).
Cluster 5 had two ecotypes (4% of the total ecotypes) that were phenotypically very distinct from others. A taxonomic identification revealed that these two ecotypes were of different species: E. crus-galli and E. muricata. Plants were the tallest (132 cm) with the largest flag leaf (18-cm length, 1.2-cm width) and panicle length (17.2 cm). Tall plants can suppress the surrounding vegetation through shading and thereby can be more competitive for resources (Weiner and Thomas Reference Weiner and Thomas1986). This cluster had the second highest seed shattering (36%) and germination capacity (86%).
Correlation Analysis of Morphophysiological Traits and Their Association with Herbicide Resistance
Correlation analysis (Figure 6) performed on 14 morphophysiological traits revealed that plant height was highly correlated with flag leaf width (r = 0.79), panicle length (r = 0.92), stem color (r = 0.91), and canopy structure (r = 0.83). In general, E. crus-galli and E. muricata in the current study were taller and exhibited wider flag leaves and longer panicles compared with E. colona. Similar findings were reported by Tahir (Reference Tahir2016) in Arkansas, where the widest flag leaves and longest panicles were observed in E. crus-galli and E. muricata, rather than in E. colona. Stem color was highly correlated with canopy structure (0.81), which represents the plant growth habit. Specifically, Echinochloa ecotypes with purple stem color tend to have open geometry with shorter stature. Conversely, ecotypes with greener stems were taller, with a closed canopy. An open canopy allows for better light penetration, though open canopy with decumbent or prostrate growth habit may pose disadvantages for hybridization. In this respect, E. crus-galli and E. muricata may have greater chances of hybridization in a rice field, owing to taller stature and larger inflorescences (OLA and MAFF 2002).

Figure 6. Correlation analysis between herbicide-resistance status and 13 morphophysiological traits measured in 54 Echinochloa ecotypes collected in rice production fields in Texas.
No significant association was observed between phenotypic traits and herbicide-resistance status (Figure 6). The multiple herbicide resistance profile of Echinochloa within each cluster is displayed in Figure 5. In Cluster 1 (total 15 ecotypes), 26% were susceptible to all four herbicides, whereas 37%, 11%, 21%, and 5% of the ecotypes were resistant to one, two, three, and four herbicide SOAs, respectively. In Cluster 2 (total 23 ecotypes), 24%, 38%, and 14% of the ecotypes were resistant to one, two, and three herbicide SOAs, respectively, whereas the rest (24%) were susceptible. None of the ecotypes in this cluster were resistant to all four tested herbicides.
Cluster 3 consisted of three ecotypes, of which two were resistant to one SOA, and one ecotype was resistant to three SOAs. In Cluster 4, which comprised nine ecotypes, 22% were susceptible, whereas 42% and 11% were resistant to two and three SOAs, respectively. All four clusters were composed of E. colona ecotypes only, and frequency of resistance to one or more SOAs was independent of cluster groupings. Similar results have been reported by Rouse et al. (Reference Rouse, Roma-Burgos, Norsworthy, Tseng, Starkey and Scott2018) in a study conducted in Arkansas, where 40% of the E. colona ecotypes were found resistant to only one SOA and 33% were resistant to two or more herbicides. Cluster 5 consisted of two ecotypes, one of them was susceptible (E. crus-galli) and the other one (E. muricata) was resistant to one SOA. In contrast to the current findings, E. crus-galli in Arkansas exhibited higher frequency of three-way resistance (three SOAs) compared with other species (Rouse et al. Reference Rouse, Roma-Burgos, Norsworthy, Tseng, Starkey and Scott2018). However, due to low sample size of E. crus-galli and E. muricata available in the current study, resistance frequencies could not be compared across the different Echinochloa spp.
This common garden study revealed the occurrence of three Echinochloa species in Texas rice production, namely E. colona, E. crus-galli, and E. muricata, among which E. colona is the predominant species. Even though Echinochloa spp. are commonly referred to as “barnyardgrass,” it is vital that the research and extension community is cognizant of the inaccuracy of attribution. The 14 morphophysiological traits investigated in this study were found to vary greatly within and among the ecotypes. Certain morphological traits, notably plant height and flag leaf size, could help with Echinochloa species classification. However, association between herbicide resistance and weed morphophysiological traits was not evident. Overall, the presence of high diversity for different morphological traits among different Echinochloa ecotypes characterized in this study suggests their ability for adaptation to different selection agents. The findings support the fact that Echinochloa spp. show high risk for the evolution of herbicide resistance and emphasize the need for proactive diversified management to tackle this species.
Acknowledgments
Assistance provided by Zachary Schaefer, Russ Garetson, Cynthia Sias, and Shirley Engelke on sample collection; and Prabhu Govindasamy, Spencer Samuelson, Blake Young, Kaisa Werner, Queli Ruchel, Vitor Damião, Camille Werner, and Michael Escobar on plot maintenance and data collection are gratefully acknowledged. The authors also appreciate the help provided by the staff (Jason Samford, Bob Hensley, Patrick Parma, Henry Nieto, and Coleen Meitzen) at the David R. Wintermann Rice Research and Extension Center, Eagle Lake, TX. This research was funded in part by the Texas Rice Research Foundation and the Rice Belt Endowment Fellowship. No conflicts of interest have been declared.