Introduction
The zonation of organisms along the intertidal gradient is one of the most striking characteristics of communities on intertidal shores. Patterns of species distribution are strongly determined by tolerance to desiccation and freezing on emergent substrates (Metaxas & Scheibling, Reference Metaxas and Scheibling1993). Intertidal zonation patterns can be modified by tidepools. These represent specific structural entities of the substrate, which provide submerged living conditions for organisms in the intertidal zone during low tide and, therefore, can extend the upper vertical limits of many organisms that are susceptible to desiccation (Firth et al., Reference Firth, Schofield, White, Skov and Hawkins2014). They are most commonly found on rocky intertidal shores, where they have been intensively studied and show specific species communities and interactions that differ from the emergent rock (Metaxas & Scheibling, Reference Metaxas and Scheibling1993; Hunt & Scheibling, Reference Hunt and Scheibling1998; Araujo et al., Reference Araujo, Sousa-Pinto, Barbara and Quintino2006; Firth & Crowe, Reference Firth and Crowe2008, Reference Firth and Crowe2010; Firth et al., Reference Firth, Schofield, White, Skov and Hawkins2014).
However, tidepools also occur in intertidal soft-bottom coastal systems (Kunishima & Tachihara, Reference Kunishima and Tachihara2020), where they are depressions in tidal flats that develop depending on local hydrography, bottom topography and sediment composition (Brenha-Nunes et al., Reference Brenha-Nunes, Contente and Rossi-Wongtschowski2016). Tidepools in sedimentary coastal ecosystems can also be formed by habitat-structuring epibenthic organisms on the sediment surface when seawater is retained by these structures at ebb-tide conditions or when these structures initiate morphological changes of the bottom, that indirectly lead to the development of tidepools. For example, Bouma et al. (Reference Bouma, Ortells and Ysebaert2009) showed that changes in hydrodynamics around patches of the non-native cordgrass Spartina anglica C.E. Hubbard may cause erosion troughs around the tussocks in the high intertidal zone of the European Wadden Sea (North Sea, North-east Atlantic), which are filled with water during low tide. Further examples of habitat-altering and tidepool-forming species in the Wadden Sea are epibenthic native blue mussels Mytilus edulis L. and non-native Pacific oysters Magallana gigas (Thunberg, 1793) (Buschbaum et al., Reference Buschbaum, Dittmann, Hong, Hwang, Strasser, Thiel, Valdivia, Yoon and Reise2009; Reise et al., Reference Reise, Buschbaum, Büttger and Wegner2017). Since the introduction of the Pacific oyster and its successful establishment on native blue mussel beds in the area in the 1980s, both species co-occur in mixed reefs of mussels and oysters. The shift from blue mussel beds to mixed oyster reefs was accompanied by a strong increase in habitat heterogeneity because Pacific oysters reach much larger sizes than blue mussels and can achieve densities of more than 1000 individuals per m2 with shells often partly fused to one another (Reise et al., Reference Reise, Buschbaum, Büttger and Wegner2017). This has caused the development of complex, towering reef structures on the sediment surface and also of an increasing formation of tidepools with a size of several m2 within the reefs (Figure 1).
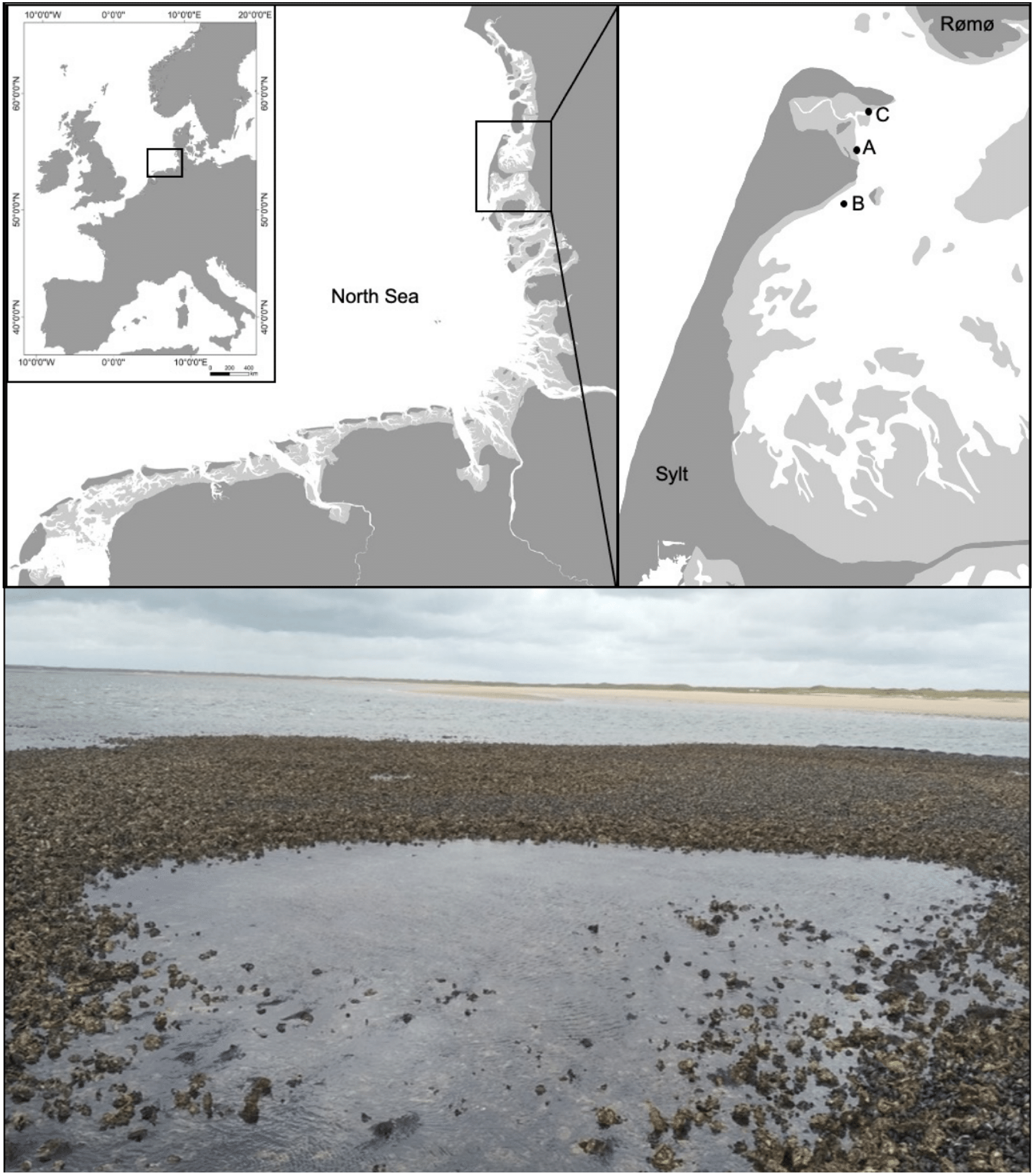
Fig. 1. The study area with the sampling sites Oddewatt (A), Blidselbucht (B) and Diedrichsenbank (C) in the north of the island of Sylt in the south-eastern North Sea. Tidepools occur within mixed reefs of mussels and oysters (photo).
It is well known that intertidal mixed reefs of blue mussels and Pacific oysters in the Wadden Sea harbour a highly diverse species community (Kochmann et al., Reference Kochmann, Buschbaum, Volkenborn and Reise2008; Markert et al., Reference Markert, Wehrmann and Kröncke2010). The newly developed tidepools represent a microhabitat within the reefs, that has the same structural components of blue mussels and Pacific oysters as the surrounding reef areas, but is permanently submerged. Therefore, this study explicitly asks whether tidepools show the same composition of key species and species relationships as surrounding oyster reefs. To compare tidepools and surrounding reef areas we selected species and their interactions, which are known for their ecological role in oyster reefs (e.g. epibionts and their consumers) and which have been intensively studied in former investigations.
Barnacles are the most abundant epibionts on the shells of blue mussels and Pacific oysters in the study area (Buschbaum, Reference Buschbaum2000; Buschbaum et al., Reference Buschbaum, Cornelius and Goedknegt2016). However, barnacle recruitment success is strongly affected by predatory crabs and the grazing activity of snails Littorina littorea L., 1758, which may achieve densities of several hundreds of snails per m2 oyster reef (Cornelius & Buschbaum, Reference Cornelius and Buschbaum2020). To compare the occurrence of barnacles and L. littorea, we quantified densities of both species inside and outside tidepools. In addition, we investigated the effects of predation and grazing on barnacle recruitment in both areas by conducting an exclusion experiment in the field and studied the snail grazing activity under emerged and submerged conditions in the laboratory.
Besides investigating densities and interactions of species living on and between the habitat-structuring blue mussels and Pacific oysters, we additionally studied the occurrence of endoparasites living inside mussels. The relationship between parasites and their host is an often neglected, but important species interaction in coastal ecosystems (Minchella & Scott, Reference Minchella and Scott1991; Poulin, Reference Poulin1999; Mouritsen & Poulin, Reference Mouritsen and Poulin2002; Lafferty & Harvell, Reference Lafferty, Harvell, Bertness, Bruno, Silliman and Stachowicz2014). Parasites can have strong direct effects on host populations by leading to increased mortality, as has been shown for blue mussels when being infected with parasitic copepods. They can also cause indirect effects on trophic interactions by altering prey preferences of predators. European shore crabs Carcinus maenas L., 1785, for example, prefer blue mussels parasitized by shell-boring polychaetes. They weaken the shell structure of blue mussels and make them easier for crabs to prey on (Ambariyanto & Seed, Reference Ambariyanto and Seed1991). In the Wadden Sea, parasites occur from primary producers to apex predators. Therefore, they can impact all trophic levels (Thieltges et al., Reference Thieltges, Engelsma, Wendling and Wegner2013), and with their effects on host species and trophic interactions, they can also influence the food web as a whole. Due to their ecological importance, we included parasites in our study as another parameter in the comparison of species distribution patterns inside and outside of tidepools.
Parasitic trematodes and copepods belong to the most prevalent groups of metazoan parasites in coastal systems (Thieltges et al., Reference Thieltges, Krakau, Andresen, Fottner and Reise2006, Reference Thieltges, Engelsma, Wendling and Wegner2013). A widely distributed trematode parasite species is Renicola roscovita Stunkard, 1932, which has a complex life cycle utilizing snails as their first intermediate host, bivalves as second and birds as final host (Werding, Reference Werding1969; Lauckner, Reference Lauckner1984; Krakau et al., Reference Krakau, Thieltges and Reise2006). Another prevalent group of parasites are copepods of the genus Mytilicola spp., which shows a direct life cycle (no intermediate host, Goedknegt et al., Reference Goedknegt, Buschbaum, van der Meer, Wegner and Thieltges2020). Both the trematode R. roscovita and the copepods Mytilicola spp. use blue mussels M. edulis as hosts (Pogoda et al., Reference Pogoda, Jungblut, Buck and Hagen2012; Goedknegt et al., Reference Goedknegt, Schuster, Buschbaum, Gergs, Jung, Luttikhuizen, van der Meer, Troost, Wegner and Thieltges2017). Therefore, we determined the densities of Mytilicola spp. and R. roscovita in blue mussels collected inside and outside of tidepools. Additionally, we quantified the occurrence of amphipods in both areas, which are well known to prey on free-living cercarial stages of R. roscovita and may, therefore, affect the prevalence of this parasite in blue mussels (Welsh et al., Reference Welsh, van der Meer, Brussaard and Thieltges2014).
We hypothesize that the density of selected organisms associated with an oyster reef, such as barnacles, amphipods, snails and parasites, differs between tidepools and surrounding oyster reefs. As a consequence, we assume that specific species interactions such as grazing activity of snails also differ inside and outside tidepools. Different distribution patterns of selected species inside and outside tidepools would indicate that they represent specific habitats within reefs of native mussels and oysters and do not only represent permanently submerged branches of surrounding reefs.
Materials and methods
Study area
All samples were taken and field experiments were performed along the north-east coast of the island Sylt in the northern Wadden Sea (Figure 1). In this area, the tides are semidiurnal (mean tidal range 2 m) with a relatively constant salinity close to 30 (Thieltges & Reise, Reference Thieltges and Reise2006). More detailed information about biotic and abiotic factors in the study area can be found in Reise (Reference Reise1985), Reise et al. (Reference Reise, Herre and Sturm1994) and Reise & Riethmüller (Reference Reise, Riethmüller, Gätje and Reise1998). For this study, tidepools were defined as follows: tidepools have a size of at least 2 m2 surface area and a water depth of at least 15 cm during low tide and they must be situated within mixed reefs (Figure 1). Samples from the tidepool habitat are further labelled as ITP (inside tidepools) and compared with samples collected on the surrounding oyster reef outside tidepools labelled as OTP (outside tidepools, with a maximum of 1 m and a minimum of 15 cm distance to a tidepool). Three structurally very similar major mixed reefs with at least 10 tidepools per reef were selected as sampling areas for this study. Site A, called Oddewatt (55°01′54.5″N 8°26′44.8″E), is located between site B, called Blidselbucht (54°59′19.7″N 8°22′51.5″E) and site C, called Diedrichsenbank (55°02′31.2″N 8°26′57.2″E). The distance between the sites is not more than 5 km (Figure 1).
Field observations
Density of barnacles, amphipods and Littorina littorea
The main barnacle species present in the study area in summer 2020 were the introduced Australian barnacle Austrominius modestus Darwin, 1854 and, at lower densities, the native balanid Semibalanus balanoides L., 1767. To determine the densities of barnacles, Littorina littorea and amphipods ITP and OTP, samples were taken from sites A and C, between 6 and 14 July 2020. At both sites, a total of six ITP and six OTP samples were taken.
A square sampling frame with a surface area of 200 cm2 was randomly placed in the ITP and OTP areas. All biogenic hard substrate (living bivalves and dead shell material) inside the square was collected and transported to the laboratory, where samples were washed with fresh water over a 1000 μm sieve. All remaining individuals of L. littorea and all amphipods in the sieve were counted. Additionally, epibiotic barnacles attached to the shells were counted and after removing the barnacles, the biovolume of the bivalve shells (living bivalves and dead shell material) per sample was measured using calibrated beakers.
Parasite distribution
To investigate the prevalence (% infected individuals from total number of investigated hosts) and intensity (number of parasites per infected host) of parasitic copepods Mytilicola spp. and the parasitic trematode R. roscovita ITP and OTP, blue mussels were collected at sites A and B in August 2020 as summer is the main time of prevalence for both parasite species (Grainger, Reference Grainger1951; Poulin, Reference Poulin2006; Thieltges & Rick, Reference Thieltges and Rick2006). Each parasite species was collected at different sampling events. Per sampling site and parasite species, we randomly collected 12 blue mussels (5–6 cm shell length) ITP and 12 blue mussels OTP. To detect parasite infection and to count the number of parasites per infected blue mussel (intensity), the mussel flesh was dissected. Afterwards, the soft tissue was squeezed between two glass plates and investigated using a stereo microscope.
In the Wadden Sea, blue mussels are infected with the parasitic copepod species Mytilicola orientalis Mori, Reference Mori1935, which was co-introduced with Pacific oysters and recently spilled over to blue mussels, and the congeneric Mytilicola intestinalis Steuer, 1902. In this study, we did not differentiate between both parasitic copepod species, because both species have a similar life cycle (Goedknegt et al., Reference Goedknegt, Buschbaum, van der Meer, Wegner and Thieltges2020).
Consumer exclusion experiment
To examine effects of predators and grazers on barnacle coverage ITP and OTP, a consumer exclusion experiment in the field was conducted using cages. Oyster spat collectors were used as settlement substrates for barnacle larvae. Oyster spat collectors are standardized plastic discs with a diameter of 15 cm. Two discs were stacked upon each other with a distance of 4 cm between them and then attached to an iron rod of 50 cm length, that was deeply (40 cm) anchored in the sediment. In total, 12 experimental units were placed ITP and OTP, respectively. Half of them were enclosed with cylindrical cages (20 cm in diameter, 22 cm height) made of a PVC net of 0.5 cm mesh size resulting in six caged and six non-caged ITP experimental units and six caged and non-caged OTP experimental units. The experiment was set up at site A (Oddewatt, Figure 1) and installed at six tidepools and surrounding oyster reefs. The maximum distance between experimental units ITP and OTP was 1 m. All grazers and predators were removed from the ‘treatment’ (caged) and ‘control’ (non-caged) plots before the beginning of the experiment, which was started on 17 July 2020 and finished after 14 days on 31 July 2020. During the course of the experiment, all experimental units were controlled daily to keep them free from e.g. floating macroalgae. Number of barnacle recruits attached to the spat collectors at the end of the experiment was extremely high and we decided to only count barnacles attached to the underside of the upper discs, that represented a well standardized settlement surface.
Snail mobility experiment
To examine the effect of submersion and emersion conditions on the movement activity of L. littorea, a laboratory experiment was conducted in August 2020. Snails of two size classes were collected from site A (Oddewatt), because we expected size dependent mobility differences. Littorina littorea between 9–15 mm were categorized as small, and snails between 18–24 mm as large (measured from base to apex of the shell). For the experiment, 10 aquaria (35 × 25 × 20 cm) were used and filled with a 1 cm layer of moistened sandy sediment, representing a low tide situation. For simulation of high tide conditions, the sediment in another 10 aquaria was additionally covered by a water layer of about 2 cm. We ensured that the snails in this treatment were completely below water during the experiment. We used this amount of water to test whether the mobility of the snails is already affected at low water coverage. The abiotic conditions for all aquaria during the experiment were kept constant by using a constant-temperature room, that also enabled constant light conditions. The snails were put into the aquaria (one snail per aquarium) and after 2 min of acclimation, the starting positions of L. littorea were marked. Snails leave a trail when moving on sediment and the length of the trail was measured with a measuring tape to the nearest mm after an experimental period of 5 min. In total, we measured the mobility of 10 small and 10 large snails at low tide and high tide conditions, respectively.
Statistical analysis
All data were processed and analysed with the statistical software R (version 3.6.3, R Core Team, 2017), and the predefined functions from the ‘car’, ‘DHARMa’, ‘ggplot2’ and ‘lme4’ packages. Data are given as arithmetic means with standard error (SE). Effects were considered to be statistically significant if the P-value was <0.05.
Different numbers of barnacles, L. littorea and amphipods were each fitted with linear mixed models (LMM) and analysed with location (outside tidepools OTP and inside tidepools ITP) as fixed factor. We fitted the factor sampling site (A and C) as random intercept to account for possible differences between sampling sites. Data regarding the barnacle density were transformed logarithmically to achieve homoscedasticity. All model assumptions were checked using ‘DHARMa residual diagnostics’ from the ‘DHARMa’ package.
Differences in prevalence and intensity of Mytilicola spp. and Renicola roscovita detected in blue mussels outside (OTP) and inside (ITP) tidepools at two sampling sites (A, B) were analysed by Chi-square tests (prevalence) and non-parametric Mann–Whitney U-tests (intensity).
Barnacle recruitment in the consumer exclusion experiment was analysed by a two-factor analysis of variance (ANOVA) with location (OTP and ITP) and cage (with and without) as experimental fixed factors. A non-constant Variance Score Test was used to test for homoscedasticity of variances. Variances of the data were heterogeneous and, therefore, data were transformed (Box–Cox transformation) to achieve homoscedasticity. We used Tukey's Honest-Significant-Difference (HSD) multiple comparison test for pairwise comparisons between treatments.
To test snail mobility of the two snail size categories (small and large) at simulated low and high tide conditions, we used a two-factor analysis of variance (ANOVA) with snail size and water coverage as fixed experimental factors. Variances of the data were also heterogeneous in this experiment (Non-constant Variance Score Test) and transformed (Box–Cox transformation) to achieve homoscedasticity.
Results
Field observations
Density of barnacles
Barnacle densities were significantly higher OTP than ITP (estimate = −1.08 ± 0.11, df = 21, t-value = −9.61, P < 0.001; Figure 2). The highest number of barnacles on blue mussels and Pacific oysters was found OTP at site C with 1283 ± 107 barnacles l−1 bivalve biovolume, which was about three times higher than ITP with 417 ± 53 barnacles l−1 bivalve biovolume. At site A barnacle densities were also higher OTP with 815 ± 52 barnacles l−1 bivalve biovolume in comparison to 308 ± 37 barnacles l−1 bivalve biovolume ITP.

Fig. 2. Mean number of barnacles L−1 bivalve biovolume (± SE) outside (OTP) and inside (ITP) tidepools at oyster reefs at site A and site C.
Density of Littorina littorea and amphipods
At site A, mean density of L. littorea OTP (589 ± 73 individuals m−2) was similar to the mean snail density ITP (556 ± 76 individuals m−2, Figure 3). At site C mean density of L. littorea was less similar with 481 ± 57 individuals m−2 OTP and 340 ± 39 individuals m−2 ITP. Densities of L. littorea were not significantly different OTP and ITP (estimate = −87.07 ± 62.82, df = 21, t-value = −1.39, P = 0.180).

Fig. 3. Mean number of Littorina littorea and amphipods per m2 (± SE) outside (OTP) and inside (ITP) tidepools at oyster reefs at site A and site C.
At site A, mean amphipod density OTP was 331 ± 94 individuals m−2 and lower than ITP with 721 ± 163 individuals m−2 and this was also the case at site C with 223 ± 45 individuals m−2 OTP and 389 ± 100 individuals m−2 ITP (Figure 3). Thus, mean numbers of amphipods showed a similar distribution pattern at site A and C, with overall significantly lower densities OTP than ITP (estimate = 277.78 ± 109.43, df = 21, t-value = 2.54, P = 0.019).
Prevalence and intensity of Mytilicola spp. and Renicola roscovita in Mytilus edulis
Prevalence and intensity of Mytilicola spp. in blue mussels differed between mussels collected OTP and ITP at both study sites. At site A prevalence of Mytilicola spp. was 17% OTP and significantly lower compared with ITP with 58% (Chi-square test: χ2 = 4.4, df = 1, P = 0.035, Figure 4A). The intensity (number of parasites per infected mussel) at site A was also lower OTP (1.0 ± 0.0 Mytilicola spp. mussel−1) than ITP (2.7 ± 0.4 Mytilicola spp. mussel−1; Figure 4B).

Fig. 4. Prevalence (% infected individuals from total number of investigated mussels) and intensity (number of parasites per infected mussel, ±SE) of Mytilicola spp. (A, B) and Renicola roscovita (C, D) in blue mussel outside (OTP) and inside (ITP) tidepools at oyster reefs at site A and site B.
At site B we found the same pattern with a significantly lower prevalence of Mytilicola spp. OTP (25%) than ITP (100%, Figure 4A; Chi-square test: χ2 = 14.4, df = 1, P < 0.001) and also a lower intensity OTP (2.0 ± 0.6 Mytilicola spp. mussel−1) than ITP (3.8 ± 0.5 Mytilicola spp. mussel−1; Figure 4B). Due to the low number of blue mussels infected with Mytilicola spp. OTP at both study sites (two individuals at site A and three individuals at site B), we analysed the pooled data from both sites (parasite intensity of 5 infected individuals OTP and of 19 infected individuals ITP), which revealed a significant difference between the intensity OTP and ITP (Mann–Whitney U-test: U = 14.5, P = 0.018).
At both study sites, the prevalence of R. roscovita was 100% OTP and 100% ITP (Figure 4C). The intensity of R. roscovita in infected blue mussels showed an opposite pattern to Mytilicola spp. and was higher OTP (603 ± 146 cercariae mussel−1) than ITP (308 ± 121 cercariae mussel−1) at site A (Figure 4D). However, this difference was marginally not significant, although the number of cercariae per blue mussel OTP was almost twice as high as ITP (Mann–Whitney U-test: U = 39, P = 0.057). At site B, intensity was also higher OTP (574 ± 139 cercariae mussel−1) than ITP (233 ± 59 cercariae mussel−1, Figure 4D). In this case the difference was significant (Mann–Whitney U-test: U = 35, P = 0.033). When the data of both sample sites were pooled, the intensity of R. roscovita showed a significant difference between OTP and ITP (Mann–Whitney U-test: U = 146, P = 0.004).
Consumer exclusion experiment
The experimental exclusion of consumers by using cages significantly affected barnacle recruitment (two-way ANOVA, df = 1, F = 6.80, P = 0.017). Additionally, barnacle recruitment was significantly affected by the location OTP and ITP (two-way ANOVA, df = 1, F = 5.81, P = 0.026; Figure 5). Barnacle recruitment OTP was similar in the treatment with cages (55 ± 12 barnacles per spat collector) and without cages (46 ± 15 barnacles per spat collector). However, number of barnacle recruits was strongly different ITP with 196 ± 53 barnacles per spat collector in the treatment with cages, which was significantly higher than 57 ± 15 barnacles per spat collector in the treatment without cages (Tukey's test: P = 0.033) and also significantly different from the treatments with cages (Tukey's test: P = 0.044) and without cages OTP (Tukey's test: P = 0.016).

Fig. 5. Mean number of barnacles per spat collector (±SE) in a field caging experiment outside (OTP) and inside (ITP) tidepools at an oyster reef at site A after an experimental period of 14 days in summer 2020.
There were no significant interactions between exclusion of consumers and location on barnacle recruitment (two-way ANOVAconsumer exclusion × location, df = 1; F = 5.81; P = 0.116).
Snail mobility
Water coverage in the aquaria significantly affected snail mobility (two-way ANOVA, df = 1, F = 63.1, P < 0.001, Figure 6) in the laboratory experiment, while mobility was not significantly different between large- and small-sized L. littorea (two-way ANOVA, df = 1, F = 2.65, P = 0.112, Figure 6).

Fig. 6. Mean distance travelled (±SE) of Littorina littorea in a laboratory aquarium experiment. Two different size classes of snails (small = 9–15 mm; large = 18–24 mm) and aquaria without and with water coverage were used.
Mean distance travelled by large snails (size of 18–24 mm shell height) in aquaria without water coverage (7 ± 3 mm 5 min−1) was less than the distance travelled by large snails in aquaria with water coverage (97 ± 21 mm 5 min−1). This pattern was also found for small snails (size of 9–15 mm shell height) with a distance travelled of 24 ± 10 mm 5 min−1 in aquaria without water coverage in contrast to a distance travelled of 100 ± 10 mm 5 min−1 in aquaria with water coverage. There were no significant interactions between water coverage and size of L. littorea on mobility (two-way ANOVAwater coverage × snail size, df = 1, F = 0.79, P = 0.379).
Discussion
The results of this study reveal that associated species of mixed reefs of mussels and oysters in the Wadden Sea do not show a uniform distribution pattern within a reef, which supports our hypothesis of different communities occurring inside tidepools (ITP) and outside tidepools (OTP). We found different densities of barnacles, amphipods as well as for parasitic copepods Mytilicola spp. and trematodes R. roscovita ITP and OTP, but also similar densities as for the snail L. littorea. The divergent distribution patterns may result from differences in abiotic conditions ITP and OTP. However, they may also be caused by species traits and species interactions in the respective areas as our consumer exclusion experiment revealed.
Densities of barnacles and snails L. littorea
Our field investigations on the distribution pattern of barnacles A. modestus and S. balanoides attached to mussels and oysters showed that barnacle densities were lower ITP than OTP. This result is in contrast to our cage experiment with similar numbers of barnacles ITP and OTP in treatments without cages and much more barnacles on mussels and oysters ITP than OTP in treatments with cages, which prevented grazing and predation. This indicates that barnacle settlement is generally higher ITP, which may be caused by aggregation of barnacle larvae within tidepools during low-tide conditions, more settling time for barnacle larvae and less desiccation stress for just settled larvae. After the settlement phase, however, it seems that post-settlement processes cause high barnacle mortality ITP, although in our experiment we did not find a significant interaction between consumer exclusion and location with respect to barnacle settlement. High barnacle post-settlement mortality within tidepools appears to be a common pattern because it is also known from many rocky shores (Singletary & Shadlou, Reference Singletary and Shadlou1983).
Barnacle survival rate OTP seems to be much higher, which finally results in higher barnacle densities OTP than ITP. The grazing and bulldozing activity of L. littorea is an important factor affecting overgrowth of epibionts on mussel and oyster shells in the Wadden Sea, and high snail densities may keep barnacle coverage on mixed oyster reefs low (Buschbaum, Reference Buschbaum2000; Cornelius & Buschbaum, Reference Cornelius and Buschbaum2020). We did not find significant snail density differences ITP and OTP, but the snail mobility experiment indicated that L. littorea has an increased movement activity when submerged, which was also found by other studies (Haseman, Reference Haseman1911; Newell, Reference Newell1958). Therefore, it is very likely that the permanent grazing activity ITP contributes to lower barnacle densities in comparison to OTP, where the snail activity is regularly reduced by low tide conditions. By using cages, we did not only exclude snails but also other consumers of barnacles such as predatory crabs and fish. They may also have contributed to the low barnacle recruitment ITP in treatments with consumer access. For example, native shore crabs C. maenas and introduced Asian shore crabs Hemigrapsus takanoi Asakura & Watanabe, 2005 and Hemigrapsus sanguineus De Haan, 1853 are very abundant on mixed reefs of mussels and oysters and cause strong predation pressure on barnacles (Cornelius et al., Reference Cornelius, Wagner and Buschbaum2021).
The similar densities of barnacle recruits ITP and OTP found in treatments without cages may be due to the short time of the experiment. The experimental time period of two weeks within the settlement phase of A. modestus only allowed to detect settlement and early post-settlement effects of L. littorea and other consumers on barnacle densities. However, we suggest that in the long term, consumption pressure on barnacles is higher ITP than OTP, because predation on barnacles is not interrupted by low-tide conditions ITP. This would result in lower barnacle densities ITP and, therefore, the pattern we found in our descriptive field investigation.
Parasite distribution patterns
The copepod parasites (M. orientalis and M. intestinalis) and the trematode parasite (R. roscovita) showed different distribution patterns. Prevalence and intensity of Mytilicola spp. were higher in blue mussels ITP than OTP, while the intensity of R. roscovita was higher in blue mussels OTP (with the same prevalence of 100% ITP and OTP). This result was different from our assumptions. We expected higher prevalence and intensities of all parasite species ITP because of constant submersion and, therefore, permanent exposition of blue mussels to planktonic infectious parasite stages of trematodes and parasitic copepods. Thus, the distribution patterns of the investigated parasites seem to be affected by additional processes. These can comprise further abiotic factors but also species interactions and parasite species traits including their life cycles.
The parasite R. roscovita shows a complex life cycle and the infection process of blue mussels starts when free-living cercariae emerge from L. littorea, its first intermediate host. The lifespan of the emerged cercariae is quite short (<1 day) and the infective period is still shorter (<12 h, Thieltges & Rick, Reference Thieltges and Rick2006). Therefore, trematode infections of blue mussels are usually highest in the close vicinity (within the range of metres) of high densities of L. littorea (Thieltges & Reise, Reference Thieltges and Reise2006; Thieltges, Reference Thieltges2007; Goedknegt et al., Reference Goedknegt, Buschbaum, van der Meer, Wegner and Thieltges2020). We suggest, however, that additional processes are responsible for the observed pattern of R. roscovita intensity in blue mussels because we did not find different densities of snails ITP and OTP. We only determined L. littorea densities in both areas at low tide conditions. However, the L. littorea distribution pattern may be different at high tide with perhaps much higher snail densities OTP, which crawl from the tidepools to the surrounding upper parts of the oyster reef. This would also have effects on the trematode infection levels in blue mussels. This cannot be answered by our investigation and should be considered in further studies.
Trematode infection processes are most intense at low tide conditions and this is explained by an accumulation of infective stages at the bottom of intertidal bivalve beds (Goedknegt et al., Reference Goedknegt, Buschbaum, van der Meer, Wegner and Thieltges2020). Here, they concentrate in remaining small puddles with a few centimetres of water depth, that maximizes contact with blue mussels and, therefore, increases transmission rates. This process may explain the high prevalence of R. roscovita OTP.
An enrichment of cercariae within tidepools at low tide is also very likely but this may be lower than OTP because of the larger water volume. It can also be masked by other factors, that may result in lower trematode occurrence ITP. For example, free-living parasite larvae are a food source for several consumers such as crabs and shrimps (Thieltges et al., Reference Thieltges, Bordalo, Caballero Hernández, Prinz and Jensen2008). This predation effect can strongly influence parasite population dynamics by interfering with transmission pathways because parasites are removed from the system preventing successful host infection. This process has been termed the ‘dilution effect’, and can result in a reduced infection risk in host species populations (Keesing et al., Reference Keesing, Holt and Ostfeld2006; Prinz et al., Reference Prinz, Kelly, O'Riordan and Culloty2009; Thieltges et al., Reference Thieltges, Reise, Prinz and Jensen2009; Johnson & Thieltges, Reference Johnson and Thieltges2010; Goedknegt et al., Reference Goedknegt, Feis, Wegner, Luttikhuizen, Buschbaum, Camphuysen, van der Meer and Thieltges2016). In marine systems, dilution effects have been intensively investigated in trematode parasites. Welsh et al. (Reference Welsh, van der Meer, Brussaard and Thieltges2014), for example, reveal that especially shrimps and amphipods strongly affect the number of planktonic free-living cercarial stages of the trematode parasite Himasthla elongata Mehlis, 1831. Cercariae of this species can be completely removed from the water column by amphipods over a 3-hour experimental period, and similar effects are also very likely for other trematode species (Welsh et al., Reference Welsh, van der Meer, Brussaard and Thieltges2014). In the study area, the amphipods Gammarus locusta L. 1758 and Echinogammarus marinus (Leach, 1815) represent the dominant amphipod species within oyster reefs. Both are omnivorous species with a diverse food spectrum and show predatory effects on smaller prey organisms (Costa & Costa, Reference Costa and Costa1999; Dick et al., Reference Dick, Johnson, McCambridge, Johnson, Carson, Kelly and MacNeil2005; Alexander et al., Reference Alexander, Dick and O'Connor2015). Therefore, we suggest that a dilution effect caused the lower prevalence of cercarial stages of R. roscovita ITP, because here densities of amphipods and shrimps were higher, and consumption is not interrupted by low tide conditions.
In contrast to R. roscovita, the parasitic copepods Mytilicola spp. show higher prevalence and intensities ITP. Perhaps, predation pressure on the comparatively large larvae of Mytilicola spp. (about 1 mm) is lower than on the much smaller cercarial stages of trematodes (about 150 μm; Werding, Reference Werding1969; Gee & Davey, Reference Gee and Davey1986). Another explanation is that the concentration of Mytilicola larvae within the tidepools during low tide is so high that despite the presence of predators enough larvae survive to cause high infection levels in blue mussels. High larval densities ITP may be a consequence of the direct life cycle of Mytilicola spp. without any intermediate hosts. Female copepods release free-living planktonic nauplii larvae from their egg sacks. After metamorphosis to the infective first copepodid stage, the larvae can spend about 2–3 weeks in the water column before moving to the bottom by a photonegative response (Gee & Davey, Reference Gee and Davey1986; Thieltges & Rick, Reference Thieltges and Rick2006; Goedknegt et al., Reference Goedknegt, Buschbaum, van der Meer, Wegner and Thieltges2020). All further development stages of Mytilicola spp. (copepodid stages II–V) take place within the bivalve host (Gee & Davey, Reference Gee and Davey1986). Thus, a single infective larva has a period of several tidal cycles to find and infect a suitable blue mussel host, because this process is not restricted to such a narrow time window as for larvae of R. roscovita. The longer larval phase also implies that recruitment of infective stages of Mytilicola spp. is independent from production of larvae of the respective oyster reef where the tidepool is located. A combination of these factors could cause a high supply of infective larval stages of Mytilicola spp. and result in high infection rates of blue mussels within tidepools.
On the other hand, the lower prevalence and intensity of Mytilicola spp. OTP than ITP may result from the structure of mixed reefs of blue mussels and Pacific oysters in the Wadden Sea in combination with the significantly higher barnacle densities OTP. Oyster reefs represent multi-layered structures with most blue mussels occurring near the bottom underneath a canopy of oysters (Reise et al., Reference Reise, Buschbaum, Büttger and Wegner2017), to which most barnacles are attached (Buschbaum et al., Reference Buschbaum, Cornelius and Goedknegt2016). Therefore, the top layer of Pacific oysters with barnacles OTP poses a barrier of filter feeders the planktonic Mytilicola larvae have to pass before reaching its blue mussel hosts (Goedknegt et al., Reference Goedknegt, Buschbaum, van der Meer, Wegner and Thieltges2020). This may result in a decreased exposure of blue mussels at the bottom of the reefs because barnacles can prey on Mytilicola larvae. Additionally, the Pacific oysters may be considered as suitable first hosts the planktonic parasites larvae encounter when reaching the oyster reef. Interestingly, Pacific oysters do not serve as host for both Mytilcola species. Pacific oysters are infected by M. orientalis as host, as this parasite-host relationship originates from the native range of both species (Mori, Reference Mori1935; Goedknegt et al., Reference Goedknegt, Buschbaum, van der Meer, Wegner and Thieltges2020). For M. intestinalis, by contrast, the Pacific oyster does not seem to be a suitable host, because this parasite has not been reported from Pacific oysters in the Wadden Sea (Goedknegt et al., Reference Goedknegt, Schuster, Buschbaum, Gergs, Jung, Luttikhuizen, van der Meer, Troost, Wegner and Thieltges2017; personal observations). Despite not being a suitable host, Pacific oysters may also contribute to lower infection levels of blue mussels positioned deep in the oyster matrix by filtering M. intestinalis larvae out of the water column but remaining uninfected. However, this process also occurs ITP, and we suggest that the cumulative effect of the filter activity of Pacific oysters and barnacles cause the lower infection levels of Mytilicola spp. in blue mussels OTP.
Conclusions
Tidepools in oyster reefs represent new habitats in the Wadden Sea and provide constant submersion to a species community that has otherwise to deal with emergence at low tide conditions. Our study shows that tidepools are not only submerged counterparts of intertidal oyster reefs with beneficial living conditions for the associated community but also finds that within the tidepools specific patterns of species densities and also species interactions emerge. Therefore, tidepools in oyster reefs have similar ecological characteristics to their counterparts on rocky shores with different community patterns compared with the surrounding emergent substrates (Metaxas & Scheibling, Reference Metaxas and Scheibling1993). In general, tidepools are important structures, that increase habitat complexity and spatial heterogeneity and, therefore, contribute to community diversity in coastal systems worldwide (Kostylev et al., Reference Kostylev, Erlandsson, Ming and Williams2005; Firth et al., Reference Firth, Schofield, White, Skov and Hawkins2014). In the Wadden Sea, tidepools within reefs of mussels and oysters are of particular importance, as they only evolved on a large scale with the introduction and successful establishment of Pacific oysters. Although comparatively small tide pools already occurred in former pure mussel beds, it was only the development of oyster reefs that led to a considerable increase in the number and size of tidepools, which also increased their ecological importance. Today, they provide the only natural and permanently large-scale submerged hard substrate in the intertidal area of the Wadden Sea and are, therefore, a completely new habitat that did not exist in this form before.
The new habitat is also not an equivalent of bivalve aggregations in the subtidal zone, because the associated species compositions and species interactions differ, too. For example, Saier (Reference Saier2000) revealed that snail (L. littorea) densities are much lower on subtidal mussel beds in comparison to intertidal beds, a difference we did not find between tidepools and surrounding oyster reefs. Although some distribution patterns are similar between tidepools in oyster reefs and subtidal bivalve aggregations, the underlying processes may be different. For example, barnacle coverage on mussels in tidepools and on mussels in the subtidal zone is lower than in the intertidal (Buschbaum & Saier, Reference Buschbaum and Saier2001; this study). Furthermore, we could show that snail grazing and bulldozing cause low barnacle occurrence ITP, but other factors such as predation by further consumers are mainly responsible for low barnacle occurrence on mussels in the subtidal zone due to low snail densities in this area (Buschbaum, Reference Buschbaum2002).
In summary, the introduction and establishment of Pacific oysters in the Wadden Sea caused the formation of new extensive tidepools within mixed reefs of blue mussels and Pacific oysters. This new habitat shows its own species density patterns and species interactions with potential effects on surrounding areas. This is exemplified with the increased prevalence of the parasite copepod Mytilicola spp. ITP, which can cause an increased production of parasite larvae and, thus, increased infection of mussels inside but also outside tidepools.
Our study is a first approach to get insights in this newly developed and expanding habitat in the intertidal zone of the Wadden Sea. We have only focused on selected species but our findings may be an initial impulse for further studies on the overall species composition, further species interactions and ecological function (e.g. nursery for fish, food source) of tidepools within oyster reefs. This would provide information on their importance for the entire Wadden Sea. It would also allow a more comprehensive comparison with comparatively well-studied tide pools on rocky coasts and, therefore, provide general insights into the ecological role of tidepools in different coastal systems.
Acknowledgements
We thank Eike Petersen, Cassandra Scheibel, Nele Scheuer and Eva Lammek for their help in the field, as well as Tobias Dolch for constructive comments on an earlier version of the manuscript. Mathias Wegner helped us a lot with statistical advice. We are extremely grateful to two reviewers for their valuable comments and suggestions, which have contributed to a considerable improvement of the manuscript. This study was funded by the German Environmental Foundation (DBU), grant no. 20018/530. We acknowledge support by the Open Access Publication Funds of Alfred-Wegener-Institut Helmholtz- Zentrum für Polar- und Meeresforschung.