Introduction
Gestational diabetes mellitus (GDM) is a pregnancy complication marked by hyperglycaemia, which, while resolving following delivery, can increase the risk in the mother of developing later metabolic dysfunction post-pregnancy, such as type 2 diabetes mellitus (T2DM). Reference Plows, Stanley and Baker1 Contributing factors to the development of GDM include overweight/obesity and poor maternal nutrition. For example, certain diets during pregnancy, such as those high in sugar, can exacerbate natural alterations to insulin sensitivity that occur across gestation, and lead to a worsening state of maternal metabolic dysfunction. For instance, a maternal diet supplemented with fructose has been shown to increase glucose intolerance in pregnant and lactating rats. Reference Zou, Arentson and Teegarden2 Currently, the best practice treatment strategy for women at risk of GDM is maintaining a healthy diet and exercise. Given the common association of sugar sweetened beverages with weight gain and metabolic dysfunction, many therefore look to artificial sweeteners (ASs) as the ‘healthy’ alternative.
AS are food additives which provide the sweet taste of sugar without the associated calories. These low-calorie sweeteners have grown in popularity in recent decades. Reference Sylvetsky, Jin and Clark3,Reference Farhat, Dewison and Stevenson4 Acesulfame-K (Ace-K) is one of the most popular AS and is a common component in diet soda beverages, Reference Buffini, Goscinny and Van Loco5 which is the most prevalent mode of AS ingestion. Consumption in pregnant women is high with over a quarter (29.5%) of pregnant women reporting intake of AS during pregnancy, of which over 5% do so daily. Reference Azad, Sharma and de Souza6,Reference Halldorsson, Strøm and Petersen7 Consumption of AS is also increased in women diagnosed with GDM, where just under half (45.4%) report AS consumption during pregnancy, and 9.2% report daily intake. Reference Zhu, Olsen and Mendola8 Further, a study of lactating mothers detected Ace-K in breast milk samples in women who self-reported not consuming AS, indicating the pervasiveness of AS in our everyday diet. Reference Sylvetsky, Gardner and Bauman9 While AS consumption has been promoted as an effective tool to help weight loss, a number of studies have suggested that it can elevate the risk for obesity and related disorders including cardiovascular disease, insulin resistance (IR) and T2DM. Reference Mathur, Agrawal and Nagpure10–Reference Fagherazzi, Gusto and Affret13 Investigations assessing Ace-K’s influence on precursor adipocytes have also determined that AS alter adipocyte differentiation Reference Simon, Parlee and Learman14 and in humans, contribute to dysregulation of adipose tissue inflammatory pathways. Reference Sylvetsky, Sen and Merkel15 Further, human studies have illustrated that both sugar sweetened and AS beverages impair oocyte and embryo quality, and implantation and pregnancy rates in women, Reference Setti, Braga and Halpern16 implicating AS in the dysregulation of reproductive as well as metabolic systems.
Despite the growing evidence suggesting adverse effects associated with the intake of AS, regulations and guidelines relating to the consumption of AS remain unclear. This is particularly true regarding AS intake during pregnancy and lactation. Currently, the Academy of Nutrition and Dietetics considers consumption of AS during pregnancy acceptable, Reference Fitch and Keim17 with current recommended acceptable daily intake level (ADI) for Ace-K for non-pregnant and pregnant individuals being 15 mg/kg body weight. Reference Yalamanchi, Srinath, Dobs, Caballero, Finglas and Toldrá18 The lack of regulations surrounding AS consumption results from a paucity of data regarding their impact on maternal metabolic and reproductive health. A study in humans has demonstrated that consumption of AS during pregnancy increases the risk of preterm birth, Reference Halldorsson, Strøm and Petersen7 which was echoed in a recent preclinical study by our laboratory, where C57Bl/6 pregnant mice supplemented with AS during pregnancy demonstrated a reduced time from conception to birth. Reference Plows, Morton-Jones and Bridge-Comer19 The same study in mice also reported maternal metabolic dysfunction following consumption of Ace-K or fructose during pregnancy.
Given the association between metabolic dysfunction during pregnancy and the increased risk of developing later disease post-pregnancy, such as the association between GDM and T2DM, Reference Plows, Stanley and Baker1 it is important to determine whether these maternal diets influence the mother’s health post-partum (PP). These influences not only impact the future health of the mother, but potentially that of her offspring. Given the difficulties often associated with delineating confounding factors in human studies, the use of animal models is essential in helping to elucidate the effects of AS consumption. Therefore, this study utilised a mouse model to investigate the impact of a maternal diet including the AS Ace-K or fructose during pregnancy and lactation on the metabolic and reproductive health of mothers post-pregnancy. Ace-K or fructose consumption across gestation and lactation increased hyperglycaemia in dams 8 weeks PP and contributed to gonadal adipocyte hypertrophy in the absence of weight gain. Further, Ace-K increased ovarian gene expression of genes associated with ovulation and folliculogenesis.
Methods
Animal procedures
All animal procedures were approved by the Animal Ethics Committee, University of Auckland (Approval number 001846) in accordance with the New Zealand Animal Welfare Act, 1999. C57Bl/6 mice breeding pairs were purchased from the Vernon Jansen Unit at the University of Auckland and housed under standard conditions (wood shavings as bedding, 22°C, 40–45% humidity, and a 12-h light: 12-h dark cycle).
All mice were maintained on a standard chow diet (Envigo, 2018 Teklad Global 18% Protein Rodent Diet, Table S1) ad libitum throughout the experiment. At 10 weeks of age, age-matched female mice were housed with an unrelated C57Bl/6 male for mating. Pregnancy was confirmed by vaginal plug and recorded as gestational day (GD) 0.5. Pregnant dams were then randomly assigned to one of three dietary groups (n = 8/group):
-
a. Control (CD; standard diet and drinking water)
-
b. Artificial sweetener (AS; standard diet supplemented with 12.5 mm Ace-K in drinking water)
-
c. Fructose (Fr; standard diet supplemented with 34.7 mm fructose in drinking water)
AS and Fr doses were calculated to be equivalent to a human dose of one standard can of soda a day. Diets were maintained throughout pregnancy and lactation. Body weight and food and liquid intakes were measured weekly. At weaning (3 weeks PP) the maternal mice were moved onto the control diet for the remainder of the experiment (9 weeks PP). An oral glucose tolerance test (OGTT) was performed 1 week prior to the end of the experimental period (8 weeks PP). At 9 weeks PP, mice were fasted for 6 h and tail blood and plasma samples were collected as detailed below. Mice were weighed then humanely killed by cervical dislocation. Prior to humane killing, maternal mice were staged to ensure all mice were in diestrus. Gonadal adipose tissue and ovaries were dissected, weighed, snap-frozen, and stored at −80°C for later analysis.
Oral glucose tolerance test
At 8 weeks PP, mice were fasted for 6 h. Following recording of body weight, the tail tip was snipped (<1 mm) and the second drop of blood was read using a glucometer (Accu-Chek Performa, Roche Diabetes Care). 2 g/kg D-glucose was administered via oral gavage. Blood glucose concentrations were measured at 0, 15, 30, 60, 90, and 120 min from the tail tip. Tail blood was also collected into EDTA microvettes (CB300, Sarstedt) at 0, 15, and 60 min. Samples were then centrifuged for 10 min/1500× g/4°C and the resulting plasma was stored at −20°C for plasma insulin analysis.
Histological analysis
Gonadal adipose tissue was fixed in 10% neutral-buffered formalin, then paraffin embedded and sectioned (10 μm) using a Leica R 2135 rotary microtome (Leica Instruments). The slides underwent hematoxylin and eosin staining followed by mounting with DPX mountant. The tissues were visualized at 20× magnification and images were captured using a light microscope and NIS Elements-D software (Nikon 800). Four representative images of each section were taken and analysed in a blinded manner with ImageJ software (NIH) for the determination of mean adipocyte size and distribution.
Plasma analysis
Mouse-specific insulin, leptin, and testosterone ELISA kits (Ultra Sensitive Mouse Insulin ELISA Kit (Cat. # 90080), Mouse Leptin ELISA kit (Cat. # 90030), and Mouse Testosterone ELISA Kit (Cat. # 80552), Crystal Chem Inc., IL, USA) were used according to the manufacturer’s instructions on a single plate.
Gene expression analysis
Ovarian RNA was extracted using RNeasy Mini Kits (Cat. No 74104, Qiagen, Hilden, Germany) and a TissueLyser (Qiagen, Hilden, Germany) as per the manufacturers’ instructions.
Adipose tissue RNA was extracted using the Trizol extraction method and a TissueLyser (Qiagen, Hilden, Germany). Following homogenization, the lysate was centrifuged for 10 min/1200 × g/4°C and the accumulated fat layer removed to improve RNA yield. Following addition of isopropanol, samples were incubated overnight at −20°C.
Following extraction, RNA was assessed with a NanoDrop spectrophotometer (NanoPhotometer N60, Implen). A High Capacity cDNA Reverse Transcription Kit (Life Technologies Ltd, Applied Biosystems) was used to generate cDNA as per the manufacturer’s instructions. PCR was performed using the Applied Biosystems QuantStudio 6 Flex Real-Time PCR system (Applied Biosystems). Genes (Table S1) were normalized to the geomean of Rps29 and Rps13 expression. The comparative CT method was utilized to analyse the results. Reference Hsieh, Lee and Panigone24
Statistical analysis
Statistical analysis was performed using GraphPad Prism and Sigma Plot. Shapiro–Wilk test was used to assess normality. Any data that was not normally distributed was transformed as appropriate. Repeated measures ANOVA was performed for the OGTT data. All other data were analysed using one-way ANOVA. Holm–Sidak post hoc tests were performed as indicated for testing comparisons between the groups. Significance between the groups was given at p < 0.05. All data are presented as mean ± SEM unless otherwise stated
Results
Body weight and caloric intake
Consumption of AS and Fr had no impact on body weight gain across pregnancy, lactation or post-weaning (Fig. 1A). Across pregnancy and post-weaning, food intake was also not altered by AS or Fr. However, across lactation (birth to weaning), female mice on the Fr diet consumed less food compared to both CD and AS groups (Fig. 1B). Despite this, caloric intake remained unchanged across the experiment regardless of diet, when the caloric contribution of fructose was accounted for (Fig. 1C). Liquid intake across pregnancy demonstrated a dietary effect, where mothers supplemented with AS and Fr consumed higher volumes of liquid compared to CD. This effect was not seen across the lactation period (Table 1). Similarly, there was no difference in absolute or relative ovarian or gonadal fat tissue weight following AS or Fr supplementation compared to CD (Table 1).
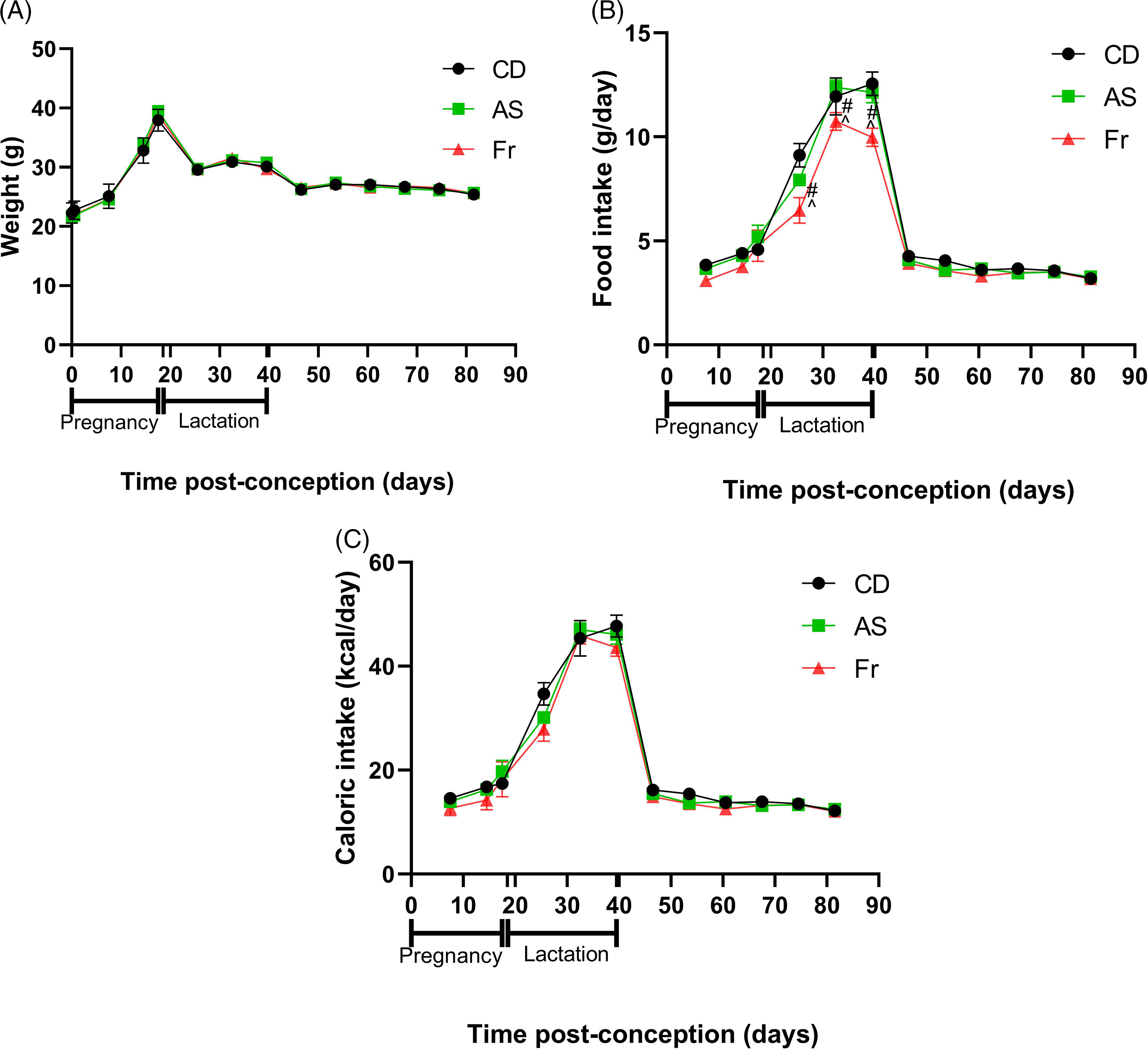
Fig. 1. The impact of Ace-K (AS) and fructose (Fr) intake compared to Controls (CD) in female C57Bl/6 mice on (A) weight, (B) food intake, and (C) caloric intake. Data presented as mean ± SEM. #P < 0.05 Fr w.r.t CD. ^P < 0.05 Fr w.r.t AS. N = 4–8/group.
Table 1. Weight of gonads and gonadal fat weight, and average liquid consumption

Data presented as mean ± SEM. N = 8/group; NS = Not significant.
* P < 0.05 w.r.t CD.
Plasma analysis
Plasma leptin, insulin or testosterone concentrations at 9 weeks PP were not different across the maternal dietary groups. Glucose and HOMA-IR were also not significantly different following AS or Fr supplementation as compared to controls (Table 2).
Table 2. Plasma biochemical analysis in female mice at 9 weeks post-partum

Data are presented as mean ± SEM, where; n = 8/group; NS = Not significant; HOMA = fasting insulin (microU/L) × fasting glucose (nmol/L)/22.5.
Oral glucose tolerance test (OGTT)
At week 8 PP, both AS and Fr groups had greater blood glucose at 30 min post oral gavage compared to CD (Fig. 2A). Fr impaired glucose tolerance compared to CD as reflected in the area under the curve (AUC), with a significant diet effect (Fig. 2B). There was no difference in plasma insulin response across the groups (Fig. 2C, 2D).
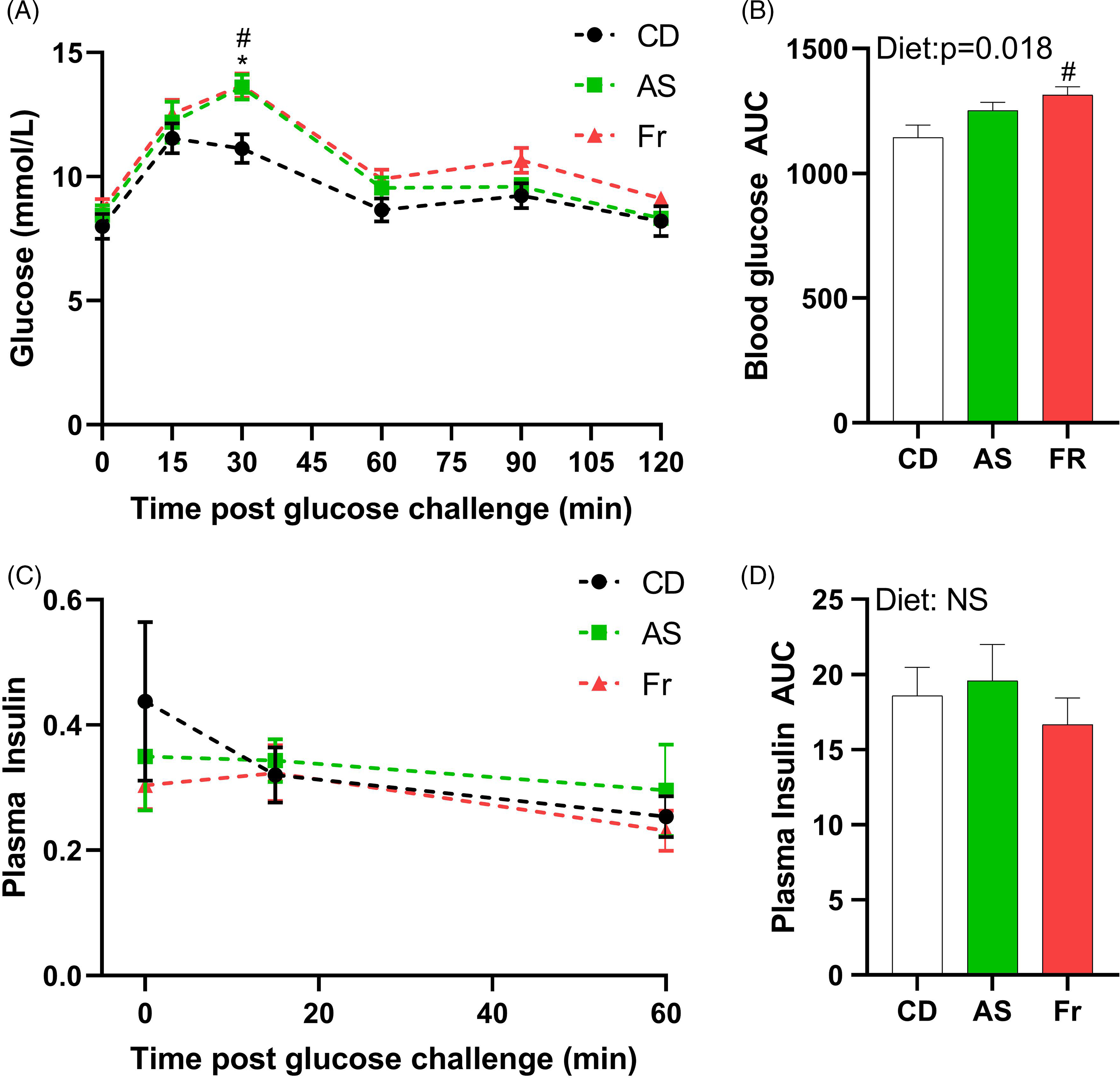
Fig. 2. The impact of Ace-K (AS) and fructose (Fr) intake compared to Controls (CD) on glucose homeostasis at 8 weeks PP female C57BL/6 mice. (A) OGTT (2 g/kg). (B) Area under the OGTT curves. (C) Plasma insulin secretion curve at 0, 15, and 60 min post OGTT. (D) Area under the curve in insulin. Data analysed using two-way repeated measures ANOVA. Data are expressed as mean ± SEM. *P < 0.05 AS w.r.t CD. #P < 0.05 Fr w.r.t CD; n = 8/group.
Gonadal adipocyte hypertrophy
Adipocyte size distribution was altered by maternal dietary group, with AS and Fr groups reduced at 1–2000, and 2–3000 µm2, but increased at 6–7000 µm2 adipocyte area in comparison to CD. AS also had a higher proportion of adipocytes at 7–8000 and 8–9000 µm2 compared to CD (Fig. 3A). There was a significant dietary effect on gonadal adipocyte area, with AS and Fr consumption increasing adipocyte area in comparison to CD (Fig. 3B).
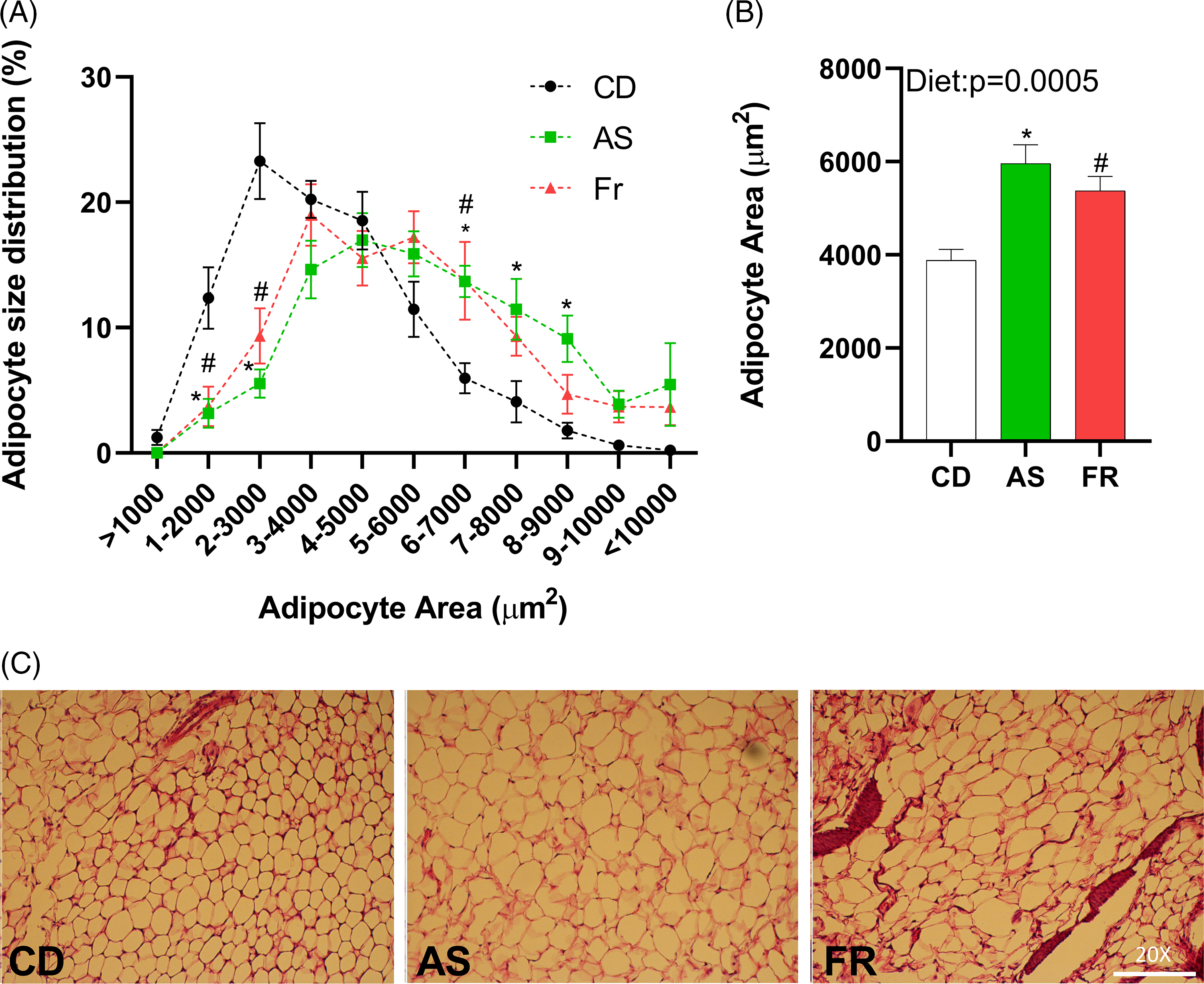
Fig. 3. The impact of maternal Ace-K (AS) and fructose (Fr) intake compared to Controls (CD) on adipocyte size in gonadal adipose tissue in female C57BL/6 mice. (A) Adipocyte size distribution. (B) Adipocyte average size. (C) Representative gonadal adipose tissue sections (haematoxylin and eosin staining), magnification 20×. Data were analysed by one-way ANOVA. Data are expressed as mean ± SEM. *P < 0.05 AS w.r.t CD. #P < 0.05 Fr w.r.t CD; n = 8/group.
Gonadal adipose tissue gene expression
There was no significant difference in gene expression of Fasn, but there was a strong trend towards an overall diet effect (p = 0.054). There were no other differences in gene expression in female mice across the different maternal dietary groups for Cluster of differentiation 68 molecule (Cd68), insulin receptor substrate 1 (Irs1), forkhead box O1 (foxo1), SRY-box transcription factor 9 (Sox9), tumor necrosis factor (Tnfα), delta like non-canonical notch ligand 1 (Dlk1), toll-like receptor 4 (Tlr4), leptin receptor (Lepr), nuclear factor kappa-light-chain-enhancer of activated b cells (Nfkb), solute carrier family 2 member 4 (Slc2a4), peroxisome proliferator-activated receptor gamma (Pparγ), peroxisome proliferator-activated receptor gamma coactivator 1 (Ppargc1) or interleukin-1 (Il-1).
Ovarian gene expression
Consumption of AS increased expression of epiregulin (Ereg) in comparison to Fr and induced an overall diet effect (Fig. 4A). Forkhead Box O3 (Foxo3a) exhibited a diet effect, with AS increasing expression in comparison to CD (Fig. 4B). There were no differences in ovarian gene expression for follicle stimulating hormone receptor (Fshr), leptin receptor (Lepr), growth differentiation factor 9 (Gdf9), bone morphogenetic protein 15 (Bmp-15), estrogen Receptor 2 (Esr2), luteinizing hormone/choriogonadotropin receptor (Lhcgr), hydroxysteroid 17-beta dehydrogenase 1 (Hsd17a1), cytochrome P450 Family 17 Subfamily A Member 1 (Cyp17a1), and progesterone receptor (Pgr) across the different maternal dietary groups.
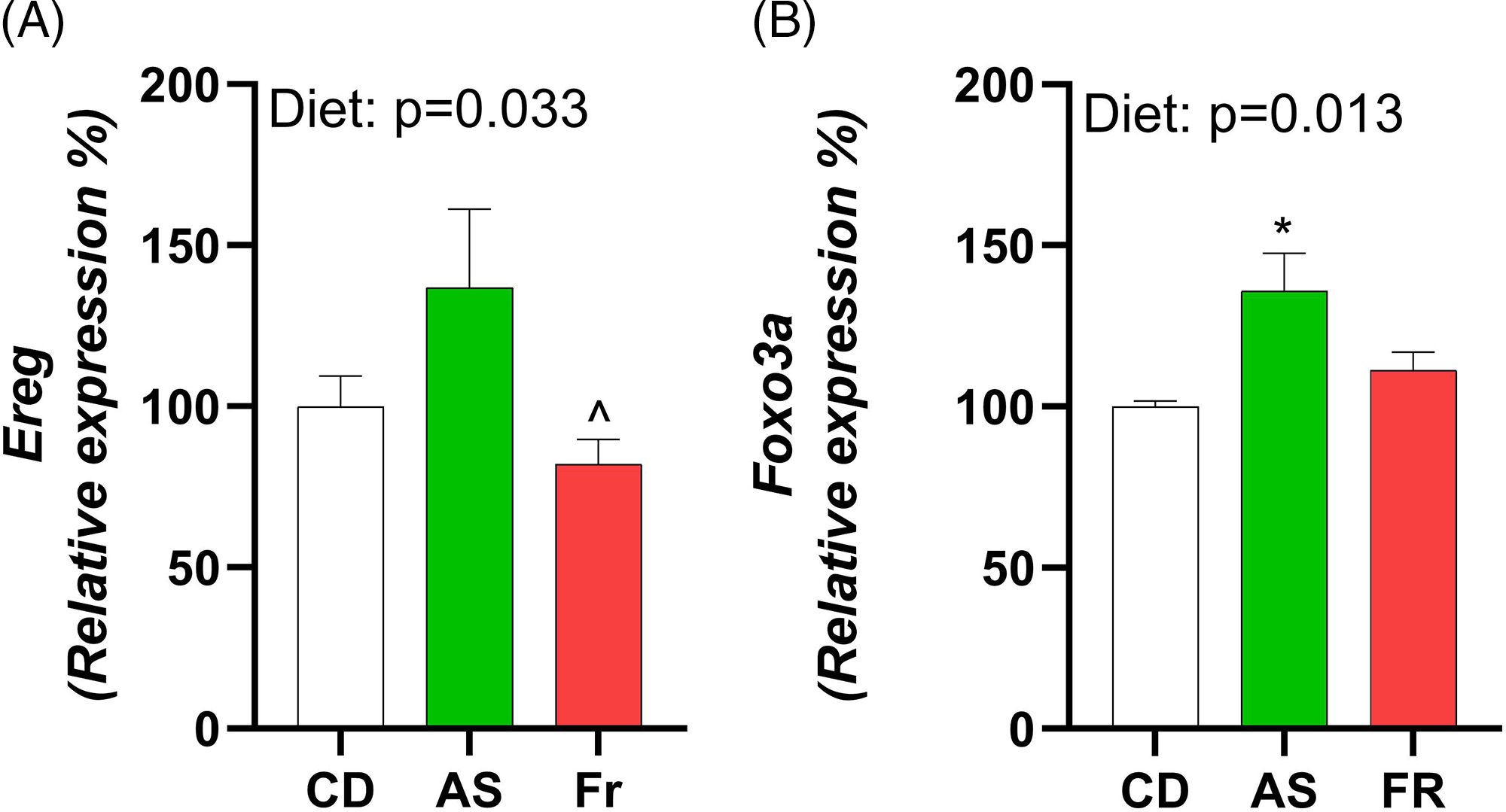
Fig. 4. The impact of Ace-K (AS) and fructose (Fr) intake compared to Controls (CD) on gonadal tissue gene expression in female C57BL/6 post-partum mice. (A) Ereg, (B) Foxo3a. Data were analysed using one-way ANOVA. Data are expressed as mean ± SEM. *P < 0.05 AS w.r.t CD. ^P < 0.05 Fr w.r.t AS. n = 8/group.
Discussion
The association of sugar sweetened beverages with increased risk of obesity, IR, and T2DM is well established. As such, given the propensity of sugars including fructose to induce negative changes in metabolic and reproductive health during pregnancy, the use of AS has been promoted as the ‘healthier’ alternative. This is despite conflicting evidence in non-pregnant human and animal studies suggesting that AS contribute to metabolic and reproductive dysfunction, and studies during pregnancy implicating AS in the inducement of glucose intolerance and further pregnancy complications. This mounting evidence is therefore concerning given the pervasiveness of AS consumption in pregnant and lactating women, and especially in those at risk of GDM. Reference Azad, Sharma and de Souza6,Reference Halldorsson, Strøm and Petersen7 However, less is known about the impact these maternal diets have on the health of the mother PP. We therefore examined the influence of Ace-K in the maternal diet on the metabolic and reproductive health of PP female mice. We found that at 8 weeks PP, Fr induced glucose intolerance compared to the control group. Despite no difference in weight or caloric intake, both AS and Fr induced adipocyte hypertrophy within gonadal adipose tissue. Further, AS may also influence reproductive function through alteration of gene markers associated with follicular development and ovulation.
There was no alteration in body weight across the groups during pregnancy, lactation and post-weaning, indicating that the effects of both AS and Fr were independent of body weight. This is consistent with previous studies that have found no difference in weight gain following supplementation with low doses of AS Reference Palmnäs, Cowan and Bomhof20–Reference Mitsutomi, Masaki and Shimasaki22 or Fr, Reference Zou, Arentson and Teegarden2 and with our own previous study in pregnant mice. Reference Plows, Morton-Jones and Bridge-Comer19 This is unsurprising given the lack of change in caloric intake between the groups across the study. It should be noted that although mice supplemented with Fr had reduced food consumption across weaning, their caloric consumption was unchanged due to the remaining energy being derived from the Fr supplemented drinking water. One theory regarding AS influence on metabolic health is caloric compensation, where the sweet taste associated with AS primes the body to expect a heightened glucose load. As AS is non-caloric, the body potentially consumes more food to compensate for the ‘missing’ calories, or, as has also been suggested, it decouples the association of sweet taste with increased calories and impairs the efficient utilisation of energy when other sweet foods are consumed. Reference Swithers23 While this study shows that food intake was not altered in the AS groups compared to CD, the standard diets used in this study were not sweetened. It could be of interest to offer a secondary diet to determine whether AS consumption alters caloric prediction and utilisation.
Our study further illustrated that Fr consumption increased plasma glucose concentrations compared to CD following the OGTT, at both the 30-min post glucose challenge mark and overall, as seen in the AUC. AS consumption increased glucose at the 30-min time point, however, was not significantly higher overall compared to CD. The OGTT was undertaken at 8 weeks PP, which is also 5 weeks following cessation of the AS and Fr diets, indicating that the effects of AS and Fr consumption across pregnancy and lactation may be long-lasting. In previous work, glucose intolerance was seen during pregnancy in mice supplemented with AS and Fr. Reference Plows, Morton-Jones and Bridge-Comer19 In women, increased glucose concentrations during pregnancy can increase the risk of developing T2DM post-pregnancy, Reference Plows, Stanley and Baker1 a result which can be implied from the glucose intolerance presented in this study.
Despite no difference in weight gain across the experiment, adipocyte hypertrophy was induced following both AS and Fr consumption across pregnancy and lactation. This effect was especially significant in AS groups compared to CD, with distribution skewed towards the larger sizes following AS consumption. Differentiation of adipocytes from their preadipocyte stage is an essential process in maintaining the health and function of adipose tissue. Distortions to this can lead to adipocyte hypertrophy. Adipocyte hypertrophy is frequently associated with increased IR, dyslipidaemia, T2DM, and the increased recruitment of pro-inflammatory markers and immune cells such as macrophages. Reference Plows, Stanley and Baker1,Reference Zou, Arentson and Teegarden2 Even in the absence of obesity, such metabolic dysfunction can persist when adipocyte hypertrophy occurs, with the enlarged adipocytes frequently a predictor of later adipose metabolic dysfunction. In previous work in mice, we determined that adipocyte area was not significantly altered following maternal diet of AS over pregnancy alone, although Fr did increase the proportion of adipocytes in the highest size category compared to CD. Reference Sylvetsky, Jin and Clark3 This contrasts with the results seen in the present study and may indicate that the alterations to adipocyte size occurred post-pregnancy, during lactation, at least in response to AS exposure.
A panel of genes associated with adipogenesis, inflammation and glucose metabolism were examined to further investigate the influence of AS and Fr on PP metabolic health. There was a strong dietary trend towards expression of Fasn following AS consumption. Fasn is involved in the synthesis of Acetyl-Co A to fatty acids. Increased expression of Fasn is associated with IR, obesity, Reference Farhat, Dewison and Stevenson4 and frequently found in actively proliferating cells, especially tumour cells where the demand for lipids requires increased lipogenesis. Reference Buffini, Goscinny and Van Loco5 However, while Fasn expression only trended higher, it may be indicative of slight metabolic perturbations that could be exacerbated if a secondary negative environmental cue were to occur. Given the increased adipocyte hypertrophy previously mentioned, it is surprising that the adipose tissue did not exhibit further alterations to inflammatory and adipogenesis markers. While the adipose tissue is a metabolically active part of the body and analysis enables a good snapshot of metabolic health, it would also be worth investigating other organs associated with metabolic health, such as the liver or gut.
As metabolic and reproductive systems are intrinsically linked, it was of interest to investigate any alterations to maternal ovarian gene expression following AS or Fr consumption. Ereg expression was increased in mice fed AS in comparison to Fr. Foxo3a also experienced a dietary effect, with AS increasing expression in comparison to CD. Ereg is an epidermal growth factor expressed in ovarian granulosa cells that mediates luteinising hormone (LH) induced ovulation. Deficiency of Ereg in mice has been associated with impaired oocyte maturation and cumulus expansion. Reference Hsieh, Lee and Panigone24 Foxo3a plays an essential role in primordial follicle development, where knockout of Foxo3a are associated with oocyte death and early depletion of ovarian follicles following global activation of primordial follicles. Reference Liu, Luo and Qian25,Reference John, Shirley and Gallardo26 Tight regulation of primordial follicular development is essential given the finite number a woman is born with. Foxo3a is therefore often called a master regulator of this process and, in turn, may be regulated through the phosphatidylinositol 3-kinase (PI3K)-Akt pathway. Given the increase in Foxo3a ovarian expression, it may be worthwhile further investigating the noted pathway to determine if there are any further effects within the ovaries. Few studies have investigated the impact of AS on ovarian gene expression, much less PP. However studies in women undergoing infertility treatments found that AS reduced the quality of oocytes and impaired assisted reproductive outcomes, Reference Setti, Braga and Halpern16,Reference Halpern, Braga and Setti27 while a study in mice fed a high-sucrose, high-fat diet found similar upregulation of Ereg to those seen in the AS group. Reference Roberts, Perets and Sarfert28 Of note, this contrasted with the effect seen on the Fr group in this study and may indicate an alternative pathway through which AS alters Ereg ovarian function.
The use of physiologically equivalent doses of AS and Fr in the current study enables a better translation between mice and human consumption. Further, it demonstrates that, compared to studies which utilise supraphysiological doses, lower concentrations can exert significant influence on the health of the mother PP. While this study focused on adipose tissue and ovarian health as areas of metabolic and reproductive function respectively, it would be worthwhile investigating other avenues such as the gut microbiome and pancreatic function. Ace-K has been shown in recent studies to perturb gut bacterial composition, and alter pathways associated with fatty acid and energy metabolism within the gut in a sex-specific manner. Through these alterations, systemic changes to health may be induced. Further, it has been suggested that distortions to the gut microbiome could contribute to chronic inflammation. Reference Bian, Chi and Gao12,Reference Shahriar, Ahsan and Khan29 There is evidence that the taste receptors activated by AS are present in the pancreas and play a role in insulin secretion. Whether this contributes to the glucose intolerance displayed in our mice during pregnancy and later life is unknown. Determining whether these effects persist past the completion of the diets would be of interest. Further, given the links between obesity and GDM, and the higher rate of AS consumption by women at risk of GDM, it would be beneficial to investigate a model of maternal AS supplementation in conjunction with a HFD to determine whether these exposures synergistically exacerbate metabolic or reproductive dysfunction. Finally, due to limited sample volumes, plasma protein and steroid hormone analysis were not performed. PCR analysis offers valuable insight through the use of key reproductive and metabolic markers, however further insights might be obtainable with the inclusion of a wider range of endpoint measures.
Maternal nutrition across pregnancy and lactation not only moulds the development of the offspring, but it can influence the health of the mother in both pregnancy and PP. AS, while promoted as the healthier alternative to sugar sweetened beverages, may induce negative metabolic and reproductive effects post-pregnancy in female mice. We showed that although AS and Fr consumption across pregnancy and lactation did not alter body weight, both diets induced adipocyte hypertrophy in gonadal adipose tissue and Fr increased glucose intolerance. In pregnant women already at risk, such as those with GDM, these perturbations may exacerbate already present metabolic dysfunction and increase the risk of T2DM or diet-induced inflammation post-pregnancy. Further, the reproductive health of the mice supplemented with AS was also impacted, with the potential alteration of primordial follicle activation and maturation noted. Currently, the literature on maternal outcomes following AS consumption is lacking in both metabolic and reproductive outcomes, with most research focused on the developmental effects of offspring. As such, the present study adds to the experimental evidence to date suggesting that AS consumption over pregnancy and lactation may not be beneficial alternatives to sugar sweetened products and emphasises the need for more research to be done with regard to this dietary component on the PP health of the mother.
Supplementary materials
For supplementary material for this article, please visit https://doi.org/10.1017/S2040174422000022
Acknowledgments
The authors acknowledge Satya Amirapu for assistance with adipose tissue preparation and processing for the histology-related work.
Financial Support
This work was supported by funding from the Faculty Research Development Fund (#3710414) at the University of Auckland and The University of Auckland Foundation (#3721401). PBC was supported by a Maori Health Research PhD Scholarship from the Health Research Council of New Zealand (#3714791).
Conflicts of Interest
The authors declare no conflict of interest.
Ethical Standards
All animal work was approved by the Animal Ethics Committee at the University of Auckland in accordance with all appropriate institutional and international guidelines and regulations of animal research.