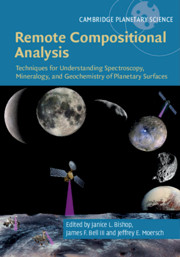
Book contents
- Remote Compositional Analysis
- Cambridge Planetary Science
- Remote Compositional Analysis
- Copyright page
- Contents
- Contributors
- Foreword
- Preface
- Acknowledgments
- Part I Theory of Remote Compositional Analysis Techniques and Laboratory Measurements
- Part II Terrestrial Field and Airborne Applications
- Part III Analysis Methods
- Part IV Applications to Planetary Surfaces
- 17 Spectral Analyses of Mercury
- 18 Compositional Analysis of the Moon in the Visible and Near-Infrared Regions
- 19 Spectral Analyses of Asteroids
- 20 Visible and Near-Infrared Spectral Analyses of Asteroids and Comets from Dawn and Rosetta
- 21 Spectral Analyses of Saturn’s Moons Using the Cassini Visual Infrared Mapping Spectrometer
- 22 Spectroscopy of Pluto and Its Satellites
- 23 Visible to Short-Wave Infrared Spectral Analyses of Mars from Orbit Using CRISM and OMEGA
- 24 Thermal Infrared Spectral Analyses of Mars from Orbit Using the Thermal Emission Spectrometer and Thermal Emission Imaging System
- 25 Thermal Infrared Remote Sensing of Mars from Rovers Using the Miniature Thermal Emission Spectrometer
- 26 Compositional and Mineralogic Analyses of Mars Using Multispectral Imaging on the Mars Exploration Rover, Phoenix, and Mars Science Laboratory Missions
- 27 Mössbauer Spectroscopy at Gusev Crater and Meridiani Planum
- 28 Elemental Analyses of Mars from Rovers Using the Alpha-Particle X-Ray Spectrometer
- 29 Elemental Analyses of Mars from Rovers with Laser-Induced Breakdown Spectroscopy by ChemCam and SuperCam
- 30 Neutron, Gamma-Ray, and X-Ray Spectroscopy of Planetary Bodies
- 31 Radar Remote Sensing of Planetary Bodies
- Index
- References
28 - Elemental Analyses of Mars from Rovers Using the Alpha-Particle X-Ray Spectrometer
from Part IV - Applications to Planetary Surfaces
Published online by Cambridge University Press: 15 November 2019
- Remote Compositional Analysis
- Cambridge Planetary Science
- Remote Compositional Analysis
- Copyright page
- Contents
- Contributors
- Foreword
- Preface
- Acknowledgments
- Part I Theory of Remote Compositional Analysis Techniques and Laboratory Measurements
- Part II Terrestrial Field and Airborne Applications
- Part III Analysis Methods
- Part IV Applications to Planetary Surfaces
- 17 Spectral Analyses of Mercury
- 18 Compositional Analysis of the Moon in the Visible and Near-Infrared Regions
- 19 Spectral Analyses of Asteroids
- 20 Visible and Near-Infrared Spectral Analyses of Asteroids and Comets from Dawn and Rosetta
- 21 Spectral Analyses of Saturn’s Moons Using the Cassini Visual Infrared Mapping Spectrometer
- 22 Spectroscopy of Pluto and Its Satellites
- 23 Visible to Short-Wave Infrared Spectral Analyses of Mars from Orbit Using CRISM and OMEGA
- 24 Thermal Infrared Spectral Analyses of Mars from Orbit Using the Thermal Emission Spectrometer and Thermal Emission Imaging System
- 25 Thermal Infrared Remote Sensing of Mars from Rovers Using the Miniature Thermal Emission Spectrometer
- 26 Compositional and Mineralogic Analyses of Mars Using Multispectral Imaging on the Mars Exploration Rover, Phoenix, and Mars Science Laboratory Missions
- 27 Mössbauer Spectroscopy at Gusev Crater and Meridiani Planum
- 28 Elemental Analyses of Mars from Rovers Using the Alpha-Particle X-Ray Spectrometer
- 29 Elemental Analyses of Mars from Rovers with Laser-Induced Breakdown Spectroscopy by ChemCam and SuperCam
- 30 Neutron, Gamma-Ray, and X-Ray Spectroscopy of Planetary Bodies
- 31 Radar Remote Sensing of Planetary Bodies
- Index
- References
Summary
The Alpha-Particle X-ray Spectrometer (APXS) is part of the scientific payload of all four Mars rovers to date. It determines the chemical composition of rocks and soils using X-ray spectroscopy during irradiation with alpha particles and X-rays from 244 cm. All elements heavier than fluorine can be detected by their characteristic X-ray lines. Typically, 16 elements are quantified for each martian sample. An additional 10 trace elements can be quantified for unusual high abundances. The APXS has provided compositional data at 4 landing sites, analyzing more than 1000 samples along a combined traverse of ~70 km. The diverse composition of soils and rocks has provided insights about martian geology and environmental conditions. Soils at all landing sites are similar and basaltic, but enriched in S, Cl, and Zn, likely from volcanic exhalations. A variety of igneous rocks have been documented. High sulfur concentrations in Ca sulfate veins, ferric sulfate subsurface soil deposits, and the extensive Burns formation with ~30% sulfate indicate extensive interactions with acidic fluids in the past. APXS bulk geochemistry complements mineralogy data and images and delivers crucial constraints for the interpretation of other investigations, like ground truth for orbital remote sensing instruments or comparison with martian meteorites.
Keywords
- Type
- Chapter
- Information
- Remote Compositional AnalysisTechniques for Understanding Spectroscopy, Mineralogy, and Geochemistry of Planetary Surfaces, pp. 555 - 572Publisher: Cambridge University PressPrint publication year: 2019
References
- 6
- Cited by