1.1 Introduction
All vibrations, more or less important to human safety and comfort, are in the frequency spectra, which can be symbolically divided into the ranges close to 0 Hz, <10 Hz, and 10–100 Hz. This paradigm exists due to the human body that remains mostly invariable and, at the same time, due to variability and huge trend to a certain miniaturization of the machine mechanisms and other aids generating and transmitting the vibrations to humans. This is determined with the dimensions, shape, other specifics of elastic and dissipative elements, and spatial location of a human vibration protection system (VPS) inside or outside the machine.
The above ranges become critically important, as new objects appear that require high quality of the infra-low vibration protection in the first place, such as in bioengineering and medical research, in micro- and nanoelectronics, and in transport vehicles such as high-speed trains, helicopters, nanosats, and other modern and next-generation machines. This is clear when considering a key parameter of a VPS, namely, its natural frequency spectra, which are changed as the VPS structure and geometry features and other characteristics are changed. For instance, the natural frequency of a single-degree-of-freedom (1DOF) VPS is

where is the natural frequency of undamped VPS,
is the coefficient of gravitation,
(m/s2), and
(m) is the parameter determining the system travel in a direction of vibration motion (see Table 1.1).
Table 1.1. The VPSs and their frequency spectra of vibrations
Type of a VPS | System parameter | Forced frequency f (Hz) | Samples of vibrating machines and mechanisms inside/outside affecting human VPSs | |
---|---|---|---|---|
Travel ![]() | Natural frequency f0 (Hz) | |||
“Macro” | (0.05–1) | 0.5–2.5 | 2–40 and over | Transport or automotive technological machines: land passenger vehicles and trucks, railroad rolling stock and infrastructural objects, subsonic vehicles, helicopters, construction equipment, etc. |
“Mini” | (1–10) ⋅ 10−3 | 5–16 | 5–70 and over | Aids of the vehicles and equipment, the engine units and elements |
“Micro” | (1–10) ⋅ 10−7 | 50–160 | 100–400 | Movable joints (bearings), MEMS, multilayer structures, and coatings |
“Nano” | (3–10) ⋅ 10−11 | 500–900 | <1,000 | Micro/nanoelectronic elements of machines, mechanisms, and structures |
In Table 1.1, a VPS we symbolically consider as the “macro” if relative displacements and deformations of its elements are measured in dozens or hundreds of millimeters. For instance, a VPS like “suspended vehicle – track” can be considered as “macro,” and human biomechanism is also a “macro-VPS.” If the displacements and deformations are within the limits of few millimeters, then we deal with a “mini-VPS.” This concerns mechanical, pneumatic, hydraulic, gas, or electromagnetic mechanisms used in a vehicle. And a system refers to a “micro/nano-VPS” if the displacements and deformations are measured in micrometers or hundreds of nanometers. Finally, the human life support systems are interrelated “mini and micro/nano” VPSs.
There are indefinite number of the models to describe a certain vibration motion of human body and parts depending on the purpose and objectives. Figure 1.1 illustrates such models. In Figure 1.1a, the natural frequencies of the human biomechanism and life systems envelop the range of . There are an infinite number of literature regarding the effects of the low-frequency vibrations on the whole human body (see one of these many, e.g., [Reference Griffin1]).
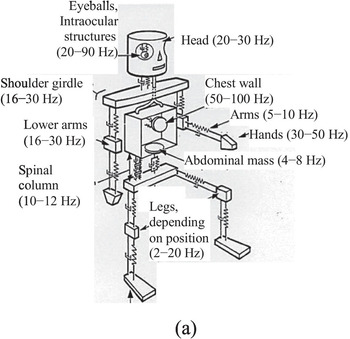

Figure 1.1 Simplest models of “macro-VPSs.” Natural frequencies of (a) human biomechanism and (b) a two-mass system of a land vehicle “carbody–1/4-car suspension−road.”
As seen, the main resonant frequencies of human VPSs are located in the range of . Besides, some human systems vibration and noise-related complaints account for 35 percent or over of all environment-related ones since human biosystems can very sensitive to the perturbation in the bandwidth close to zero values, namely,
[Reference Sim, Sung, Cheon, Lee, Lee and Lee2]. This problem is ubiquitous regardless of age and residence region. The impacts increase direct health-related effects such as performance issues, sleep disturbance, and annoyance, as well as indirect ones such as dysfunction of the autonomic nervous and cardiovascular systems. Obviously, these effects will increase as the transportation distance and time increase.
Furthermore, a composite spectrum of a land vehicle “macro”-VPS includes three typical bandwidths of natural frequencies. These are vibrations of sprung mass () and unsprung mass (
) and the structure-borne vibrations (usually,
) of the vehicle body and its structural elements (Figure 1.1b). A sprung mass of a car includes the masses of chassis (or body), engine, exhaust system, transmission parts (e.g., differential gear), as well as the masses of a human operator, passengers, and/or cargo. While an unsprung mass consists of the tires, elements of function-generating mechanisms, control arms, braking systems, and so on.
Excitation in a “mini”-VPS is generated by the vibrations in the numerous movable joints (bearings, guiderails, etc.) of the mechanisms and other aids or due to vibration of unbalanced elements of operating engines depending on the driving mode as sampled, for example, in Chapter 10 (see Figure 10.8) [Reference Lee and Goverdovskiy3]. The relative displacements in the joints are small in comparison with the overall dimensions of the mechanisms and units. The natural frequency spectra of these “mini”-VPSs are from , however, the resonances are distributed in a range from
to hundreds of Hz due to relative displacements and deformations of individual joints and drivetrains (kinematic chains joined). We will discuss these aspects in Chapters 8–10 in more detail.
It would seem that there is little in common in the vibration dynamics of a “mini/nano”-VPS like conventional widespread rolling bearings and unmanned aircraft such as nanosats (especially at the starting, i.e., least reliable, flight phase).
The nanosats took advantages of smart telephony and other widespread technology based on the MEMS and NEMS. They provide the most cost-effective way of using the space. However, in terms of the vibration spectrum generated by both VPSs, they have a common feature. Namely, the most intense vibrations in different types of rollers and sliding bearings are generated at the low- and medium-frequency spectra (an envelope spectrum) from [Reference Hirabayashi, McCaa, Rebandt, Rusch and Saha4]. While, a variety of micro/nanoelectronics elements inside the nanosats orbital modules and other cutting-edge technologies are under intensive low-frequency vibrations in the frequency range from almost zero to 125–150 Hz, as well as intense linear accelerations (the crest factor is 4 and over), when risking being destroyed long before the start of orbital work. However, the nanosats suffer infant mortality. The environment vibration and shock impacts affect the mission failure. For instance, 80 percent of the deployable mechanisms and chips have failures [Reference Batsuren, Hatamura, Masui and Cho5].
A comparison of the natural frequency spectra ,
of the VPSs sampled above and forced frequencies
of vibration excitation shows that humans using machines are permanently under broadband resonance vibrations. Most of these vibrations occupy the spectra up to 100 Hz and the most intensive at
. These are the infra-low-frequency vibrations, which are most harmful and dangerous for human health and work activity. These vibrations are most harmful and dangerous for many types of machine as well.
Below we selected several types of transport vehicles that are most widespread in a human?machine system and whose vibration activity is most harmful and dangerous to humans. We focus on such types of vehicles since their vibrations affect humans most intensively and harmfully just in the infra-low-frequency range due to dramatic coincidence of the natural frequency spectra of the human VPS and external sources of forced vibrations acting on these systems. Next, we will try to theoretically and practically show how to break this “vicious circle” that worsens human health and activity, as well as the productivity of human–machine systems both operating and most promising.
1.2 Vibrations and Vibration Protection in High-Speed Train Systems
1.2.1 The Limits of Transport Infra-frequency Vibrations: Quality Criteria for Vibration Protection Systems
The transport vibrations affect passengers and crewmembers and have even greater impact on factory and office workers and residential areas [Reference Hanson, Towers and Meister6]. Therefore, the vibrations are strictly limited by general health and industrial standards [7, 8]. The standards are used to compare the limits and intensity of vibrations in the infra-low-frequency range. The standards define a frequency-weighted vibration acceleration (m/s2):

where is a root-mean-square value (RMS) measured in the x, y, or z direction of vibration motion within a time T(s) and filtered at ith frequency.
The weighted curves are displayed in the frequency domain. If the frequency domain vibration analysis is the method of measurement, then the RMS of accelerations at the central frequencies in the range given are defined in absolute (m/s2) or relative (dB) units as


where is the total integration time of the accelerations
,
m/s2 is the limit value of the acceleration. The time measurement is, for example,
s in steady-state vibration motion, and, for example,
s in a transient [Reference Harris9].
Most transport vibrations are random. Therefore, a fast Fourier transform (FFT) can be a more efficient algorithm of the vibration analysis both in lab and field conditions [10, Reference Rao, Kim and Hwang11]. The power spectral densities (PSD) are used to characterize random vibrations as well. A PSD is computed by multiplying each frequency bin in an FFT by its complex conjugate, which results in the only real spectrum of amplitude measured in g2 units. The key aspect of a PSD, which makes it more useful for random vibration analysis, is that this amplitude is then normalized to the frequency bin width to get units of g2/Hz. By normalizing the result, one can get rid of the dependency on bin width so that we can compare vibration levels in signals of different lengths.
Based on the measurement data, one may define nondimensional measures of VPS efficiency. The transmissibility and crest factor
are among the parameters of the system efficiency [Reference Magnus12]:

where is the damping ratio informing that a VPS can be undamped
, underdamped
, critically damped
, or overdamped
; here Q is the system Q-factor, and
is the frequency ratio.
The vibration transmissibility is a criterion of the VPS quality in steady-state vibration motion. This is used to evaluate the mechanical Q-factor in a certain range of stiffness and damping control.
The crest factor is the criterion to estimate the quality of a VPS under impulsive excitation:

where is a peak value of the frequency-weighted accelerations.
The and
measured values shall not exceed the vibration limits by recommendations of ISO 2631 in assessing the human exposed to whole-body vibration and shock [7]. These data are used to compare them with the limits, as well as compare the quality of different types of VPSs.
The above measured data are then used to evaluate the ride comfort by nondimensional parameter, for example,

Vibration-induced health conditions progress slowly. In the beginning, people are tired of the vibrations, leading to distractibility, wrong decision-making under stress, and so on. As the vibration exposure continues, pain appears, and then the pain develops into an injury or disease. The pain is the first health condition that is noticed and should be addressed in order to stop the injury. A special parameter is introduced to evaluate the harmful and dangerous effects of vibration and, accordingly, to interrupt this harmful process for humans in a timely manner. This is the vibration dose value (m/s2):

which is used to define the vibration impact for a certain exposure time (hr) [7].
Figure 1.2a illustrates the vibration limits for all categories of humans (whole-body) if vibration exposure times are 2.5 to 24 hr [7]. By using these data, one can estimate discomfort experienced inside a moving train and the efficiency of the VPSs of rolling stock. For instance, for passengers and crew of a train, the vibrations are limited to the levels of m/s2 (measured by using 1/3-octave filters), if exposure time is
. This limitation concerns short-distance railroad lines. Evidently, the levels must be limited to
m/s2 for a long distance, since the exposure time would be increased
.


Figure 1.2 Vibration limits by general and industrial standards for human safety and comfort. Shown are the limits for vertical (solid line) and horizontal (dashed line) vibrations with reference to railroad transport (a) inside a moving train for different exposure time and (b) outside the train.
The limits of vibrations affecting different categories of people within a workday or a 24-hr period are shown in Figure 1.2b. By using these limits, one can estimate efficiency of the VPSs designed to protect, for example, railroad infrastructural objects (tracks, bays, depots, tunnels, bridges, etc.) from ground-borne vibration and thus the human environment outside a passing train. For workplaces and residential areas surrounding the railroad, the vibration limits are stricter: m/s2 [8].
1.2.2 Vibration Exposure Affecting Humans Inside and Outside a High-Speed Train
1.2.2.1 Actual Levels of Railroad Vibrations
High-speed railroad systems are under intensive development. They became dominant in some domestic transportation and are expected to produce long-distance intercontinental passenger traffic and freight service [Reference Hung, Brunello and Bunker13, 14]. This developmental trend requires a new strategy to improve vibration protection inside and outside moving trains, especially in the infra-low-frequency range, , which is the most harmful for human health and work activity [15].
The rail transport generates complex vibrations [15–18], some of which are increased at higher speeds of a moving train. Figure 1.3 shows the data measured on the ground within a radius of 7.5 m from ballasted track when a train passed at speeds of 70 and 200 kph [Reference Iwnicki18]. Thus, actual levels of vibrations outside the train can reach aw ≥ 0.1−12 m/s2, which exceed the limits by 250 percent and more. This is a serious issue for humans living and/or working near a high-speed railroad.

Figure 1.3 Infra-low-frequency vibrations generated by a moving high-speed train: actual vibration levels in comparison with vibration limits outside the train (on the ground), where 1 and 2 are the levels at the speed of 70 and 200 kph.
Furthermore, in work [Reference Iwnicki18] the data of simulating the motion of a passenger high-speed train carbody under vibrations in the frequency range are presented. From analysis of these data, one can conclude that the passengers and crew are in comfortable conditions if the train speed is less than 200−250 kph. However, train speeds already reach 350–600 kph and faster.
Discomfort appears and increases as the speed increases. Since the parameter of ride comfort calculated by Eq. (1.3) reaches . Perhaps, the conditions inside the train that are considered as comfortable on short lines would become uncomfortable as the distance and time of transportation increases. Thus, a long distance of high-speed railroad passenger traffic is another issue for humans inside the train due to increased speed (see Figure 1.4).

Figure 1.4 Infra-low-frequency vibrations generated by a moving high-speed train: actual vibration levels and their gain inside the train as the speed increases.
The speed increase results in drastic differences between actual levels of the infra-low-frequency vibrations and the limits set by health standards. These high vibrations affect humans inside and outside of a high-speed train. The rolling stock and infrastructure of high-speed rail systems for a long-distance from 1,000–2,000 to 14,000 km are planned for an intensive development in the foreseeable future (see, e.g., [Reference Hung, Brunello and Bunker13]). Apparently, duration of a leg of a route of a passenger high-speed train will increase from 10–20 min to 2–4 hr. Then the issue of infra-low-frequency vibrations will sharply aggravate, and by Eq. (1.4), their harmful effects on humans will increase in proportion to the dose of vibration, , where TL и TS are the vibration exposure time for long and short distances. In this case, the up-to-date railroad VPSs will become ineffective for protecting the passengers and crew. Apparently, fundamentally new railroad VPSs will be required, which must be much more effective especially in the infra-frequency range,
.
1.2.2.2 Trends in Improvement of the Existing Railroad VPSs
Many types of VPSs for railroad rolling stock and infrastructural objects have been created and are continuously improved to increase ride comfort of crewman and passengers as well as protect people in residential and industrial areas located near railroad systems.
In general, a railroad VPS is multicascade. Not all the cascades are effective enough, especially in the infra- and low-frequency ranges, which are the most critical for human health and work activities as previously mentioned. However, one can (quite conventionally) focus three cascades that make a major contribution for reducing the most harmful and dangerous vibrations affecting humans inside a moving train and outside the train at a distance of some meters to several tens of meters from the train. These VPS cascades include the engineer or passenger seat suspensions (it could be conventionally cascade 1), a car or locomotive bogie suspensions (cascade 2), and a set of track bed elements (cascade 3).
As to cascade 1 (seat suspensions), Figures 1.5 and 1.6 illustrate the vibrations affecting humans inside a regular fast train. These vibrations are most intensive in the frequency range, [Reference Orvnäs19]. Thus, these kinds of railroad VPSs are the amplifiers of vibrations rather than vibration isolators in some frequency bandwidths most harmful to humans.

Figure 1.5 Actual levels and the limits of the infra-low-frequency vibrations on the stage 1 (engineman seat) of railroad VPSs in a regular passenger fast train, where 1 and 2 are the vibrations on the cab floor and seat cushion at the train speed of 120 kph.

Figure 1.6 Actual levels and the limits of the infra-low-frequency vibrations on the stage 2 (carbody) of railroad VPSs in a regular passenger fast train, where 1 are the vibrations on the cab floor at the train speed of 120 kph and 3 and 4 are the limits for exposure times of 12 hr and 8 hr.
A methodology of improvement of existing railroad VPSs and development of new ones (cascade 1), namely, the engineman and passenger seats without/with passive/active mechanical, pneumatic, or combined suspensions with/without external dampers are discussed in more detail in Chapters 2, 4, 7, and 10.
As to cascade 2 of railroad VPSs, the designers mainly focus on softening the bogie suspensions to reduce the vibrations affecting carbodies. A bogie is usually double-suspended, a primary suspension provides tonnage and safety of the bogie, as well as to a certain extent, reduces the dynamic impact on the track. While a secondary suspension ensures ride comfort inside the train (see in more detail, for example, the works [Reference Wang, Zeng, Wei and Zhu16, Reference Orvnäs19–Reference Sugahara, Kazato, Kogan and Nakaura22]). The layouts, type diagrams, and data of structural enumeration of the most widespread mechanical, pneumatic, and combined bogie suspensions (single and double) with/without systems of external dampers are presented in Chapter 4 (see sampling in Tables 4.12–4.14).
By studying and analyzing the simulation and development test data presented in numerous reference books and papers, including the above mentioned, one can conclude the following. The bogie suspensions provide effective vibration protection in discrete bandwidths of the infra-low-frequency range (Figure 1.7). First of all, this is achieved due to a certain compromise between the train speed, on the one hand, and selection of the type and combination of elastic elements and the level of damping in bogie suspensions, on the other hand.

Figure 1.7 Vibration protection efficiency of the railroad train bogie suspensions, where 1 and 2 are the min and max vibration levels on the floor of a carbody of the high-speed passenger train, in comparison with vibration limits for exposure times of 8 hr and 24 hr.
As seen from Figure 1.7, deficient vibration protection efficiency of the cascade 2 (bogie suspensions) of the railroad VPSs takes place. And the actual levels of vibrations exceed the limits by a greater as the train speed increases.
This requires new methods and technology to increase ride comfort especially as the duration of high-speed transportation will be increased. However, the designers of existing VPSs focus still on softening the secondary pneumatic suspensions with variable (a minimal) air-damping. For instance, in work [Reference Sugahara, Kazato, Kogan and Nakaura22] the vibrations on the carbody floor in a running test of the high-speed train up to 300 kph are simulated and studied. These models of bogie suspensions provided vibration protection in some discrete bandwidths of the infra-frequency range. Similar methods of damping control in the primary and secondary bogie suspensions can decrease 1.4–1.5 times the resonance peaks. However, such VPSs are not effective enough or completely ineffective in the most critical infra-bandwidth of , regardless of the damping control mode.
Furthermore, the vibrations of carbodies tend to increase due to the weight reduction and application of rigid air springs in the bogie suspensions. Therefore, the natural frequency spectra, , of this cascade 2 of the railroad VPSs tends also to increase, where
is the complex stiffness of the suspension and bending stiffness of the carbody, and
is the sprung mass. Besides, the spectra of forcing frequencies are extended also.
Different elastic and damping elements are embedded under rails, sleepers, and slabs that are reasonable in terms of usefulness and cost playing a role in the elements of the cascade 3 when using ballasted tracks. However, efficiency of the infra-low-frequency vibration protection outside a moving train by using such railroad VPSs is very poor (see Figure 1.8, curves 1 and 2).

Figure 1.8 Vibration protection efficiency of the rail tracks, where 1 is the infra-low-frequency vibrations on the ballasted tracks, 2 and 3 are the responses of the ground when using the ballasted tracks and sections with floating track bed elements, and 4 and 5 are the limits.
As is also illustrated in Figure 1.8 (curve 3), the floating tracks can improve vibration protection outside a moving train, including road foundation and ground, much effectively. Therefore, development and application of floating track bed elements are a widespread trend for track sections of different types of rail transport near depots, as well as near uptown and territories of contiguous high-tech industry (see, e.g., the works [14, Reference Iwnicki18, 23]).
Floating tracks are highly effective in the middle- and high-frequency ranges of 100 to 1,000 Hz. However, the floating tracks are designed by using coil compression springs [23]. These rigid metal springs have linear elasticity. Therefore, when the spring travel is limited (usually, ), a VPS using such springs is effective in the frequency range
and completely inefficient in most of the infra-frequency range (less than 10 Hz). This cascade 3 of the railroad VPSs (floating sections) reduces the resonance peaks, however, without vibration protection of required level in the infra-low-frequency range as required by the standards (see Figure 1.8, curves 4 and 5). Thus, the quality of vibration protection of humans in factories and residential areas neighboring the railroads is far from the required level even by using the floating tracks.
Finally, let us briefly analyze the quality of the infra-low-frequency vibration protection when using, perhaps, one of the most promising types of rail transport.
This is the maglev train. The name “maglev” is derived from “magnetic levitation” and represents a system of rail transportation that uses, for example, two sets of magnets: one set to repel and push the train up off the track, and another set to move the elevated train ahead (see Figure 1.9a). A maglev train does not touch the surface of the track due to the controllable gap between the carbody and guiderail, taking advantage of the lack of friction. The maglev system looks like a VPS with quasi-zero stiffness. This technology can minimize both the stiffness and damping between the carbody and track and thus could provide perfect vibration protection in a range including close-to-zero frequencies. Figure 1.9b illustrates how close the developers came to a solution so as to minimize such vibrations. However, the resonances remain in the infra-frequency range due to bending of the guiderail [Reference Ma, Song, Xu, Zang and Luo24]. Thus, the problem of the infra-frequency vibrations and thus the need of vibration protection remain open.


Figure 1.9 A perspective for improvement of the high-speed railroad VPSs: (a) schematics of production prototypes of the maglev systems and (b) frequency responses of the maglev VPS-models in the infra-low-frequency range.
The maglev systems could be applied to different types of railroad transportation. However, they are potentially the best trains for urban and intercity lines of 30‒100 km. Since there is a great number of various engineering and economic restrictions for long-distance systems. For instance, the maglev cannot operate on existing infrastructure the magnets are heavy, and construction of guiderails and power inputs and of their maintainenance are extremely expensive.
1.3 Vibrations and Vibration Protection in Helicopters
1.3.1 Dangerous Vibrations Generated by Mechanical Systems of a Helicopter
Civil and special-purpose helicopters are global dominants in the aerospace industry [25]. However, their infra-low-frequency vibrations are a serious disadvantage and thus hamper the progress of the development of next-generation helicopters.
Abnormal vibrations affect the fuselage and degrade crew efficiency, passenger comfort, and the safety of airborne equipment, especially in the infra-low-frequency range of 1–20 Hz [7, 8, Reference Narain, Punia and Yadav26]. In Figure 1.10 we selected some instant fragments of development test of the helicopter. One can see, in a matter of seconds, that the infra-low-frequency vibrations destroy the helicopter, the consequences of which are catastrophically dangerous for the structure [27], and thus for humans inside and outside the helicopter.




Figure 1.10 Some instants of a development test of the helicopter: (a) first 15 s (normal operation), (b) between 16 and 22 s (initiation of a fuselage fracture), (c) at 28 s (destruction of engine unit and transmission), and (d) at 35 s (complete failure).
There are many sources that cause the vibrations in a helicopter. Such sources include the main and tail rotors, main and tail blades, power unit, transmission gearboxes, and tail shafts. The rotating unbalanced elements of machinery and gears, and the structural resonances in operating frequencies and their harmonics, are the main sources of abnormal vibrations in the frequency range . These vibrations impact the fuselage of a helicopter and thus crew, passengers, airborne equipment, and cargo in a flight.
All parts of the helicopters are subjected to forced vibrations in the infra- and low-frequency ranges, which can be considered as input vibrations to estimate an influence of them on humans or airborne equipment inside a helicopter. The vibrations in the range are very intensive. Thus, one may expect that just these vibrations have the most effect on crew and passengers. This note is especially important since the daily vibration exposure time in the helicopters used for passenger traffic and freight service can reach 4–8 hr and longer. Despite considerable progress due to improvement of helicopter designs, the vibrations in the above range remain significantly higher than the levels required to ensure safety and flight comfort by the vibration standards. The above mentioned and many other studies have shown that conventional technologies of vibration protection in helicopters are ineffective in this frequency range. These technologies can neither predict vibration levels under specific flight conditions nor reduce infra-low-frequency responses to the levels specified by the standards (see next section) [7, 8].
1.3.2 Efficiency of Vibration Protection Technologies in Helicopters
The developers create various technologies to suppress helicopter vibrations. However, the method of dynamic absorbing vibrations of the main rotor has received the most focus. For instance, dynamic antiresonant vibration isolators (DAVIs) and similar mechanisms are still dominant [Reference Newton28–Reference Welsh30]. DAVIs suppress the vibration of the main rotor and thereby reduce fuselage vibrations in a narrow bandwidth. Figure 1.11 illustrates that such systems can reduce 2, 3 times the excitation affecting seated pilots, at discrete infra-low frequencies of 1, 10, and 16 Hz [Reference Lee, Goverdovskiy and Sotenko31]. Nevertheless, this input reduction is not sufficient to meet the vibration limits by the standard [7, 8] within a regular flight, not to mention a long-time flight with refueling (when using an external tank).

Figure 1.11 Effectiveness of DAVI technology for the main rotor as the most widespread narrow-band vibration protection method in helicopters.
Furthermore, aircraft companies develop and actively use the technology by the method of structural vibration protection. The “structural manipulation” with soft mountings for the power unit, and absorbing liners in appropriate zones on the fuselage can provide vibration protection in the middle- and high-frequency ranges of 100–1,000 Hz. Active control systems produce better results. The researchers have focused on two main areas: applying higher harmonic pitch control to the rotor blades and active control of structural responses. A number of actuators are connected on the fuselage to generate vibration at a certain frequency. This vibration is to be in antiphase to input vibration and thereby minimizes the response. The flexi absorbers and active structural vibration protection systems are under development, however, their efficiency is significantly inferior compared to the absorbers of the DAVI-type [Reference Konstanzer, Enenkl, Anbourg and Cranga32].
Although the vibrations are mitigated by improving the helicopter design, the overall levels of vibrations affecting the fuselage remain significantly higher than the vibration limits. Therefore, different types of individual VPSs are required to protect crew, passengers, and airborne equipment. For these purposes, the methods and technology of vibration protection by using soft mountings made of rubbers, wire ropes, and other elastic-dissipative materials have received the most focus nowadays [Reference Bramwell, Done and Balmford29–Reference Lee, Goverdovskiy and Sotenko31]. The ergonomics of seats for pilots and other crew members is improved through comfortable soft cushions made of synthetic or natural materials and additional options increasing comfort and seat serviceability. These improvements provide effective vibration protection in the range of . Besides, the seat cushions can be supplied with shock-absorbers [33].
The pneumatic suspensions have recently been designed for aircraft crew by analogy with the VPSs to the land vehicles to improve the infra-low vibration protection. These suspensions are designed by using conventional parametric elements such as rodless (rigid) air springs [Reference Camille and Jonathan34]. In combination with active control, they could be effective in a certain frequency range. However, such improvement involves reducing the resonance peaks rather than vibration isolation to a required level since the VPSs designed using rigid metal, rubber, and air springs or their combinations are inherently inefficient in the infra-frequency range of . Also, the VPSs for airborne equipment designed using similar rigid springs can become “collection points” for vibrations that result in loss of stability or even fatalities [27, Reference Robins35].
1.4 Vibrations and Vibration Protection in Transport Land Vehicles and Automotive Construction Equipment
1.4.1 Vibrations in Land Vehicles and Construction Equipment
There is no counting the numbers and variety of degradations and failures that occur in any kind of transport land vehicles or automotive technological machines due to infra-low-frequency vibrations. Excessive vibrations in this frequency range occur from everywhere and seriously affect the operators and passengers. These are polyharmonic vibrations of engines, mechanical aids (control mechanisms) and different types of rigging, random vibrations of the structures and transmission drives, chaotic vibrations of the structures, and working substances of pneumatic and hydraulic mechanisms [Reference Jazar36–Reference Hua and Gandee38]. We illustrate in Chapter 10 the results of our experiments in the different fields for some types of automotive technological machines.
However, the more intensive vibrations in the infra-low-frequency range affect the drivers and passengers of all types of land vehicles and the operators of the automotive technological machines due to the micro and macro irregularities of the road surface and ground roughness. Our unpretentious contribution to experimental study of the transport vehicle vibrations, illustrated also in Chapter 10, could be supplemented by an endless stream of measurement data obtained by many researchers.
Similar and higher intensity of infra-low-frequency vibrations, , affecting a human operator, passenger, or cargo take place in small and medium trucks [Reference Chonhenchob, Singh, Singh, Stallings and Grewal39, Reference Hartwig40]. Furthermore, these harmful and dangerous vibrations are increased many times in a heavy truck as sampled, for example, in work. The test data indicate the vibrations on the floor of a vehicle’s body or cabin, that is, passed through several cascades of the land vehicle VPSs, including the tires (cascade 1) and body or/and cabin suspensions (cascade 2 and/or 3). These intensive vibrations can be considered as input vibrations into a driver and/or passenger seat (cascade 4) of the human VPSs inside the vehicles. However, this cascade (seat suspensions) is also inefficient from the infra-low-frequency vibrations. For instance, the results of measurements of a heavy truck ride quality indicated unacceptably high levels of the driver’s daily vibration dose in all roads [Reference Granlund41].
All the aforementioned and many other experimental studies indicate and confirm human health and safety troubles due to essential intensity and duration of infra-low-frequency vibrations, despite the fact that the norms of the human whole-body vibrations (in accelerations) are only 0.5 m/s2, while the exposure times are reasonable (medicogenetic) [7].
1.4.2 Efficiency of Existing Vibration Protection Systems in Vehicles
Taking into account the models of two and more cascade transport VPSs like those schematically shown in Figure 1.1b, the curve in Figure 1.12 can be considered as a paradigm explaining how inadequate the efficiency of the infra-low vibration protection of humans in land vehicles is. This is nondimensional displacements of sprung mass relatively to excitation inputs due to a road macro and micro roughness. These inputs pass to the cabin floor from the road surface through the wheel and body suspension and can result in the gain of vibration accelerations of the floor until and more. Evidently, such responses, with a small change, are typical for many land vehicles and automotive construction equipment, as examined in Chapter 10.

Figure 1.12 A possible (max) combined efficiency of the first (low-pressure tires) and second (soft suspensions of the carbody or cabin) cascades of a land vehicle VPS under forced vibrations in the infra-low-frequency range.
From comparison of the response in Figure 1.12 with the natural frequencies of the human body (see the model in Figure 1.1a), it becomes clear why a seated human operator or passenger of a moving vehicle or construction equipment is permanently at resonance condition in the frequency range of .
Thus, the seat suspensions can be considered as the last hope to provide vibration protection at least to the level of general and industrial standards. Therefore, seat suspensions have to be the most effective equipment of a vehicle cabin to protect the human operators and passengers against whole-body vibration. Suspensions are discussed in detail in many publications (see, e.g., [Reference Krejcir42‒55]). A multitude of these and similar seat suspensions can be classified into three groups, subject to intensity of the vibration inputs and vibration protection performance, including the following:
1. The suspensions operate under small-amplitude vibrations in cruising speed motion of the vehicles on a high-quality road that can result in either weak or no vibration protection. The reason is low mechanical Q-factor of the FGMs, which may exhibit a certain lock-up behavior due to Coulomb friction in the movable joints of the FGMs and other structural members of the suspensions.
2. The suspensions within the second group are the most common. They operate under relatively higher amplitudes of the vibrations in the vehicle motion on a relatively rough road or a “smooth” off-road. The performance reaches a certain quality if there is no bottoming and topping in the vibration motion of the FGM structural elements. The amplification of output vibration changes into an attenuation of input vibration depending on the workmanship of the mechanisms and predominant parameters of the input vibration.
3. The performance in the third group of suspensions relates to higher displacement amplitudes mostly in vertical direction of vibration motion of the object that is to be protected. These displacements may exceed the travel of structural elements of the FGMs and result in shock with either the bottom or top end-stops of the FGMs. Then such suspensions are the vibration amplificators rather than vibration isolators.
Suspensions of the first and second groups provide the working travel around . Such suspensions predominate of total base of the VPSs designed and used for land vehicles and construction equipment. The quality of vibration protection recommended by the standards is formulated from evaluation of the suspensions of the second group mainly. The suspensions of the third group are used mostly to provide the travel
, and elastic end-stops are used in addition so as to limit the motion beyond this displacement range [Reference McManus, Clair, Boileau, Boutin and Rakheja53].
However, this prediction does not come true because the experimental data inform us the opposite. Thus, the problem of vibration protection of operators and passengers in most land transport vehicles and construction equipment cannot be considered as satisfied in the infra-low-frequency range. Figure 1.13 demonstrates, for greater persuasiveness, that seat suspensions are not a panacea to provide high-quality vibration protection in the infra-low-frequency range. Perhaps, this is a maximum effectiveness of the best widespread types of mechanical seat suspensions with passive control (see Figure 1.13a) and pneumatic and combined ones with active control (see Figure 1.13b) [Reference Lee and Goverdovskiy3].


Figure 1.13 Efficiency of the third cascade (seat suspensions) of the VPS in a land vehicle or construction equipment under infra-low-frequency vibrations: (a) mechanical suspensions with passive control and (b) pneumatic suspensions with active control. All suspensions are equipped with external fluid dampers of different levels of the damping force.
Experimental data in Figure 1.13 were obtained in the process of testing seat suspensions on vibration exciters under identical conditions and then subsequent selection of quality data for many dozens of samples. Total number of tested seat suspensions is more than 130 types. As seen, all seat suspensions reveal deficient efficiency in the infra-low-frequency range of . Moreover, they operate as vibration amplifiers rather than vibration isolators in some bandwidths of this range. The suspensions differ in efficiency slightly despite significant differences in design. Obviously, the main reason for this is that the suspensions with passive and active parameter control are structured of identical parametric (elastic and damping) elements. The active parameter control using the positive or negative feedbacks weakens the resonances at several frequencies (see for more detail the works [Reference Sun, Jolly and Norris56‒Reference Sun, Gao and Kaynak61]). However, this is not enough to improve the quality of vibration protection of people inside and outside transport vehicles, especially in the infra-low-frequency range, at least to the level required by general and industrial safety standards. In subsequent chapters, we formulate rational approaches and demonstrate the possibilities of their practical use to significantly improve the quality of human vibration protection in transport vehicles.
1.5 Conclusions
1. The infra-low-frequency vibrations of
are most dangerous and harmful for human health and work activity. Such vibrations affect a person, as a driver or passenger, inside a moving vehicle a significant part of the day. During the rest of the day and at night, vehicles and other vibrating machines also have a certain devastating effect outside the human environment.
2. The main reason is a dramatic coincidence of the spectra of natural frequencies of the body, internals, and life support system with the spectra of frequencies of forced vibrations generated by moving machines. What are the most effective methods of vibration protection to break this “vicious circle,” destructive for both the person and the machine? Apparently this is the method of vibration isolation. Finally, what should be the mechanisms of vibration isolation to separate the natural frequencies of a human VPS and the frequencies of forced vibrations inside and outside a “human–machine” system so that, metaphorically speaking, “to part them to different corners of a vibro-boxing ring”?
3. We have focused on the infra-low-frequency vibrations from a few types of transport vehicles affecting humans inside and outside. These are high-speed trains, helicopters, and land vehicles and construction equipment. First, just these machines are the most common among those that people use most often. Second, these extremely painful and long-time affects since the human natural frequencies dramatically coincide with forced vibrations of the machines in the same frequency spectra. This puts body, internal, nervous, cardiovascular, and other life support systems in a permanent broadband resonance. Third, the above types of vehicles of next generation are in a stage of intensive development. However, a possible harmful effect of the vibrations of new machines on humans is not known yet.
4. The tires, body and/or cabin suspensions of land vehicles and construction equipment, or bogie suspensions of high-speed trains, are inefficient in the infra-low-frequency range, not to mention helicopters, in which there is practically no means of vibration protection of crew and passengers. Furthermore, these suspensions operate as vibration amplifiers rather than vibration isolators in this frequency range. Besides, such harmful and dangerous vibration impacts on humans increase as the machine speed and exposure time increase.
5. Personal protective equipment, like seat suspensions, could improve the vibration protection of humans inside and outside moving transport vehicles. However, this is a last frontier to protect humans and does not meet the requirements on safety and comfort, despite significant progress in development. Pneumatic suspensions are prevalent. In active control, they are the best among the other types of existing suspensions. However, pneumatic suspensions are effective insufficiently in the range
regardless of control method.
6. Fundamentally other design methodology and proper technology are required to provide the infra-low-frequency vibration protection for humans inside and/or outside transport vehicles, construction equipment, and other machines. Since a gap between the efficiency of existing suspensions and the vibration limits required for humans safety and comfort may increase at least three times and more, especially in the range
. Besides, new potentially hazardous vibrations appear with the advent of next-generation machines, for example, in the near-zero frequency range of 0.05–5 Hz, which are critical for human health and activity.
7. The following chapters dedicate a theory and design methodology for new vibration protection systems with mechanisms of negative and quasi-zero stiffness. The design methods we formulate and describe in detail will help designers to avoid harmful effects of critical infra-low-frequency vibrations in harmony with existing machines and the human environment. Besides, the methodology could be helpful, to a certain extent, for predicting how much more progress could be made in designing next-generation vibrating machines.