Introduction
Investigations of the structural form of ice sublimed from water vapor onto a cold substrate have been carried out by many investigators using different methods, i.e. X-ray diffraction, electron diffraction, calorimetry, and electron microscopy using the selected-area diffraction method.
When water vapor sublimed on a base at a temperature above −85° C, the X-ray pattern of the ice was found to be normal hexagonal by Reference Burton and OliverBurton and Oliver (1935). At temperatures below −110° C, the pattern consisted of two diffuse lines; ice demonstrating such characteristics was described as vitreous ice.
A determination of the lattice constants of hexagonal ice at −66° C was made using the X-ray diffraction method by Reference MegawMegaw (1934) with the results:

From these measurements the ratio c/a is 1.628, which does not agree with the value for close packing (1.633).
An investigation of the cubic and hexagonal forms of ice was carried out by Reference KönigKönig (1943) using the electron diffraction method. Ice specimens were formed on a thin collodion film in a low-temperature unit designed for transmission experiments with an electron-diffraction camera. The temperature of the specimen was controlled by a heater in the apparatus. The ice specimen formed at −170° C gave a diffraction pattern of diffused rings. When the specimen was heated to about −140° C, ring patterns characteristic of the diamond-type cubic structure were obtained. The specimen formed at about −80° C produced the electron diffraction ring pattern of hexagonal ice. The lattice constant of the cubic ice was calculated to be 6.36±0.01 Å.
Reference HonjoHonjo and others (1956) designed a cold stage for electron diffraction analysis of the structure of ice formed at temperatures from −70° to −170° C. The ice specimens were formed on a cooled collodion film on a grid in the cold stage by injecting water vapor from a glass tube containing moisture-laden silica gel. The transmission diffraction patterns of hexagonal ice were obtained above −80° C. Ice specimens formed at −150° C showed the ring patterns of cubic structure. Ice formed below −160° C was found to give diffuse halo patterns. The (222) ring from cubic ice and the corresponding (0004) ring from hexagonal ice were observed. It is suggested that when the scattering by hydrogen atoms in Pauling’s position is taken into account, the observed intensity of the rings agrees well with the calculated value.
Reference Blackman and LisgartenBlackman and Lisgarten (1957), using both transmission and reflection electron diffraction, observed three types of diffraction patterns, the type depending on the temperature of the substrate, a cooled collodion film or silver film. Below −140° C diffused rings were obtained. Between −140° and −120° C a sharp ring pattern characteristic of the diamond-type cubic pattern was observed. Between −120° and −100° C a sharp ring pattern of both the hexagonal and cubic ice types was found, and above −100° C only the hexagonal pattern was produced. The lattice constants of the hexagonal ice were calculated to be

and those of the cubic ice were

Reference BertieBertie, Calvert and Whalley (1963), examined the transformation of cubic ice to hexagonal ice using X-ray diffraction. When a fresh sample of cubic ice was kept at −103° C, no change was observed within 2 h, but hexagonal ice was observed on the following day. When the cubic ice was kept at −140° C, no change was observed for 6 d, but hexagonal ice was detectable at −120° C in 2 or 3 d. When the sample temperature was increased to −103° C, a great amount of hexagonal ice was detected in 15 min, and was completely changed to hexagonal ice in 75 min. The difference in rate of transformation at −103° C in the above experiments is very striking, and shows that the rate depends on the thermal history and probably on the nucleation of hexagonal ice at the sample temperature.
Reference Defrain and Trong LinhDefrain and Nguyen Trong Linh (1966) obtained diffused rings from X-ray diffraction of ice at temperatures below −140° C, diffraction rings of cubic ice at temperatures between −140° C and −80° C, and rings of hexagonal ice at temperatures warmer than −80° C. Reference McMillan and LosMcMillan and Los (1965) observed exothermic reactions of ice crystallization in a vacuum chamber at low temperatures, and discussed the recrystallization from cubic to hexagonal at a temperature of −87° C.
Ice crystals of sub-micron diameter were first observed by Reference HallHall (1950) using the replica method, and then by Reference Hibi and YadaHibi and Yada (1959) using an electron microscope. The first direct observation of ice crystals formed on a cooled Formvar film at temperatures from −70° to −120° C was made by Reference Fernández-MoránFernández-Morán (1960), who was also the first to obtain the Moiré pattern exhibited by hexagonal ice crystals grown on a single-crystal film of mica at −80° C. Reference Vertsner and ZhdanovVertsner and Zhdanov (1965) also made direct observations of ice crystals and the electron diffraction ring pattern of hexagonal, cubic and amorphous forms of ice at temperatures from −70° to −160° C.
This paper presents the results of measurements of the lattice constants and coefficients of thermal expansion of ice crystals, and reports on the temperature dependence and the transformation of the structural form of ice.
Experimental Procedure and Specimen Preparation
The heat exchange for the cooling system was built into the specimen chamber of the electron microscope. The specimen was cooled to −170° C by the use of liquid nitrogen. Intermediate specimen temperatures were provided by the cold-stage heater. The temperature was measured at the specimen by means of a ring-shaped thermocouple consisting of a copper-stainless steel junction formed during construction of the bi-metal specimen holder. The specimen rested against the thermal junction. A microvolt meter, calibrated at 0° C and at −196° C, the boiling point of liquid nitrogen at 1 bar pressure, was used to measure the temperature. The vacuum of the electron microscope was measured by an ionization gauge.
The ice crystal specimens were formed on the surface of a collodion film, a thin foil of gold, a thin cleavage surface of mica, or a grid of copper on the specimen holder of the cold stage. The surface to be used for ice crystal formation was cooled to the desired temperature of less than −90° C in pure nitrogen gas at atmospheric pressure. A steep temperature gradient existed on the surface of the specimen holder. A small amount of water vapor was introduced into the specimen holder, causing minute droplets to form above its surface. The ice crystal specimens grew on the surface of the cold substrates by sublimation of water vapor from the minute droplets. Ice crystal photomicrographs and their electron diffraction patterns were obtained with the electron microscope in the temperature range −90° to −180° C with a vacuum of 10−4 to 10−5 mm Hg.
Measurement of Lattice Constants
The Iattice constants of the hexagonal and cubic ice crystals were determined by comparing their patterns with those from a standard substance, sodium chloride or gold, which was shadowed in a vacuum chamber on one part of a thin collodion film on the electron microscope grid. At room temperature the electron-diffraction pattern of this substance was recorded on a photographic plate. Ice crystals were then formed on the filmed grid at temperatures from −90° to −180° C. An ice pattern and a combined pattern of ice and standard substance were made on photographic plates by a slight shift of the grid.
The camera constant λL can be obtained for the standard substance by the relation of a Bragg reflection,

where d is the spacing of the (h, k, l) plane, r is the radius of the ring pattern from the (h, k, l) plane, λ is the electron wave-length, and L is the camera length.
The d-spacings can be obtained from the accurately known value of the lattice constant of sodium chloride, a = 5.601±0.003 Å at −130° C (Reference Blackman and LisgartenBlackman and Lisgarten, 1957). This value was in good agreement with that obtained by estimation based on thermal data using the Grüneisen formula.
Once λL is determined for the standard ring pattern, the d-spacing of the specimen can be obtained by dividing λL by the measured radius r of the ring patterns for the specimen. The radius values are based on eight determinations of each ring diameter, each being taken twice at 45° intervals. The observed value of λL for the standard substance, the average measured values of 2r for each ring pattern from the ice, and the calculated values of the lattice constants for hexagonal and cubic ice are tabulated in Table I.
Table I. Determination of Lattice Constants

The values of the lattice constants were:

In the case of the hexagonal ice crystals, the a spacing was measured from four patterns of {10.0}, {11.0}, {12.0} and {30.0} rings which did not involve the c spacing. The c spacing was determined from the measurement of the combined patterns of the {00.6} and {30.2} rings, and the {00.2} ring (Table I). The temperature of the specimens was measured within an error of about 5 deg by the calibrated copper-stainless steel thermocouple.
In this experiment lattice spacings of hexagonal ice for high indices such as {10.6}, {11.6}, {31.2} and {31.3} were observed for the first time (Table II).
Table II. Interplanar Distances for Hexagonal and Cubic Ice*

Most of the observed ring patterns of the diamond-type cubic ice coincide within the error of observation with the ring patterns of the hexagonal ice. In the examination of many diffraction patterns, very weak traces of the {400} and {222} ring patterns from cubic ice, and the {00.4} ring pattern from hexagonal ice, were observed.
Diffraction Pattern and Grain-Size of Ice
A large number of hexagonal crystals with diameters of 0.1 to 2.0 μm, with the most frequently occurring diameter 0.3 μm, were formed on copper grids previously cooled to −100° C. The basal and prismatic planes were revealed upon examination (Fig. 1a). The average height of the hexagonal crystals was about 0.7 of the diameter of the hexagonal base. This is close to the value 0.8 obtained by Reference KrastanovKrastanov (1943) and Reference Wolff, Weickmann and SmithWolff (1957) for an equilibrium form of hexagonal ice when only the interaction between the nearest oxygen neighbors was taken into consideration.
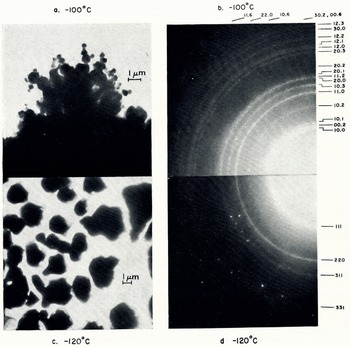
Fig. 1. (a) Hexagonal ice crystals formed on a copper grid at −100° C, (b) their hexagonal ring patterns, (c) hexagonal and cubic ice crystals formed on a collodion film at −120° C, (d) spot patterns from the hexagonal ice and sharp rings from the cubic ice shown in (c)
Transmission electron diffraction patterns for ice crystals of known size distribution (Fig. 2) were observed. The width of the diffraction ring depends on the grain size of the ice crystals, the camera length, the diameter of the electron beam, the amount of electron beam absorption by ice, etc. Using a beam diameter of about 20 μm and a selected area of 50 μm2, the transmission electron diffraction pattern of hexagonal ice in the diameter range 0.1 to 2.0 μm, with a most frequently occurring diameter of 0.3 μm (Fig. 1a), showed ring patterns with some spots in the ring (Fig. 1b). Ice crystals larger than 1 μm in diameter showed spot patterns. When the most frequently occurring diameter was larger than 0.3 μm, the spot pattern was more prominent and the ring patterns became faint (Fig. 3a, b). With further growth of the 0.3 μm crystals in the direction of the electron beam, the characteristic needle-like patterns of electron diffraction were observed (Fig. 3c, d). Minute crystals, possibly around 500 Å in diameter, gave sharp ring patterns, (Fig. 4a) and even more minute crystals less than 100 Å in diameter gave broad diffraction rings (Fig. 4b) as was observed by Reference Vertsner and ZhdanovVertsner and Zhdanov (1965).

Fig. 2. The size distribution of ice crvslals shown in Figure 1a

Fig. 3. (a) Hexagonal ice crystals grown on a copper grid at −110° C, (b) electron diffraction pattern of (a) showing prominent spots and faint rings. (c) hexagonal crystals grown further in the direction of the electron beam, (d) the characteristic needle-like patterns of the electron diffraction of (c)

Fig. 4. Electron diffraction patterns of ice crystals
In an examination of many diffraction plates, faint traces of the {00.4} ring of hexagonal ice were observed in some plates, and very weak traces of {400} and {222} ring patterns from cubic ice were present. The observed lattice spacings of hexagonal ice (Fig. 1a) are shown in Table II.
Temperature Dependence of the Structural Form of Ice
A series of experiments was carried out on crystals formed at temperatures from −90° to −180° C to determine the structural forms present at these temperatures. At temperatures above −100° C hexagonal crystals formed (Fig. 1a, b). At −120° C both hexagonal and cubic ice formed on the collodion film. The cubic crystals were detected by their electron diffraction patterns. The hexagonal ice crystals grew to a diameter of 1 to 3 μm, and the cubic crystals grew to a diameter of less than 0.1 μm (Fig. 1c). The rate of growth of the hexagonal crystals was more than 10 times greater than that of the cubic crystals at −120° C. The cubic ring patterns from {111}, {220}, {311} and {331} and the spot patterns from the hexagonal crystals are seen in Figure 1d.
The ice crystals formed at −140° C from the residual water vapor in the electron microscope showed {111}, {220}, {311} and {331} cubic diffraction rings (Fig. 4a). At −170° C the cubic ring patterns appeared (Fig. 4b), although the rings were more diffuse than those at −140° C. It is concluded that a very minute form of crystalline cubic ice is present at −170° C. These experiments have shown that the structural form of the ice depends on the temperature of the substrate on which it forms.
The four types of electron diffraction patterns produced by ice at temperatures from −100° to −180° C are shown in Table III, and the results of experiments by many workers (as well as those reported here) on the temperature dependence of the structural form of ice at low temperatures are summarized in Table IV.
Table III. Types of Electron Diffraction Patterns of Ice Crystals Formed at Various Temperatures

Table IV. Temperature Dependence of the Structural Form of Ice Crystals Formed at Low Trmperatures

Transformation of the Structural Form
Transformation of the structural form of the ice by temperature change was examined by comparing the electron diffraction patterns of the ice before and after the temperature change.
When hexagonal ice crystals that were produced at −90° C were cooled to −140° C, the temperature of cubic ice formation, no change in structural form was noted even after about an hour at the lower temperature.
Similarly, the combination of hexagonal and cubic ice which was formed at −120° C did not change structure when cooled to −170° C (Fig. 5a, b).

Fig. 5. (a) Ice crystals formed on a collodion film at −120° C. then lowered to −170° C, (b) electron diffraction pattern of (a) at −170° C
Ice crystals formed at −170° C on collodion film under a poor water vapor supply condition by residual water vapor in the electron microscope showed diffused ring patterns corresponding to the {111}, {220}, {311} and {331} rings of cubic crystals (Fig. 4b). These were microcrystaIline cubic crystals. When these minute crystals were warmed to −140° C the rings appeared sharper because of the growth of the crystals but the same rings were observed, indicating no structural transformation. It was found that cubic ice crystals are formed below −100° C at a lower rate of growth than that of the hexagonal ice; minute cubic ice was detected even at −170° C; and no transformation of the structural form was observed after the specimen temperature was changed.
Cubic ice crystals formed at −140° C were warmed to −100° C. No direct transformation from cubic to hexagonal was observed within two hours during the experiments. However, the transformation may be possible by the occurrence of hexagonal ice nucleation at temperatures above −130° C.
Thermal Expansion of Ice
The coefficient of linear expansion of polycrystalline bulk ice was determined by Reference Jakob and ErkJakob and Erk (1928) in the temperature range 0° to −240° C. The measurements were made on rod-shaped ice frozen slowly in paper tubes from the outside in. The ice showed no indication of any regular orientation of the crystal axes.
The coefficient of expansion for ice can be calculated from the lattice constants obtained at various temperatures (Table V). The lattice constants of hexagonal ice were measured by several workers by diffraction methods at temperatures between 0° and −180° C. The lattice constants measured in the present experiments and by Reference Blackman and LisgartenBlackman and Lisgarten (1957) made use of electron diffraction techniques. Others listed in Table V used the X-ray diffraction method. The discordance of the data in Table V, which may be due to the different forms of the specimens, has been noted by Reference LonsdaleLonsdale (1958), who desired a new determination with the same kind of specimen varied over a wide range of temperatures.
Table V. Lattice Constants of Hexagonal Ice

Comprehensive low-temperature measurements were made by Reference LaPlaca and PostLaPlaca and Post (1960) with a Geiger-counter diffractometer. Specimens were prepared on a cooled glass specimen holder by spraying a fine mist of distilled water. The specimens were warmed up to just below the melting point and then cooled slowly down to a certain temperature to get sharp diffraction for lattice-constant measurements. The c/a ratio of the ice formed from a fine mist was smaller than that of the ideal value of 1.633 for close packing. The ice may have uncquivalent tetrahedral bonds, i.e. the hydrogen bond along the c-axis might be slightly shorter than the centro-symmetric hydrogen bonds or, alternatively, the O-O-O angles may differ slightly from the exact tetrahedral value. However, the c/a ratio was 1.633 in this experiment and for the experiments of Reference Blackman and LisgartenBlackman and Lisgarten (1957) for ice formed from the vapor at an air temperature of approximately −100° C and a low vapor pressure of about 10–5 mm Hg.
The density and the coefficient of expansion of ice can be found from the unit-cell parameters obtained at various temperatures from 0° to −180° C (Table VI). The values given in Table VI were obtained from the curves of unit-cell parameters shown in Figure 6 by taking the molecular weight of ordinary water as 18.016, and Avogadro’s number as 6.02486×1023 mol.−1 (Reference Cohen, DuMond and FlüggeCohen and DuMond, 1957). The coefficients of cubic and linear expansion of hexagonal ice are shown in Figure 7 along with the results of other workers. The coefficient of linear expansion along c-axis is almost the same as that of the a-axis. Therefore it is almost the same as that of polycrystalline ice as determined by Reference Jakob and ErkJakob and Erk (1928).

Fig. 6. Unit cell parameter of hexagonal ice determined by Blackman and Lisgarten (B), Camp (C), Kumai (K), LaPlaca and Post (•), Megaw (M). Truby (7), and Vegard and Hillesund (V)

Fig. 7. The coefficient of thermal expansion of hexagonal ice determined by Kumai (K; Kc, along the c-axis), LaPlaca and Post (Lc, along the c-axis), and Jakob and Erk (J, polycrystalline ice)
Table VI. The Unit-Cell Parameters, the Densities, and the Coefficients of Expansion of Ice at a Pressure of 1 Bar

Summary and Conclusions
The formation and lattice spacings of the hexagonal and cubic forms of ice crystals were observed by the use of a cold stage in an electron microscope within the temperature range −90° to 180° C.
Hexagonal ice formed on the cold substrates at temperatures above −100° C. At temperatures from −100° to −130° C, both hexagonal and cubic forms of ice were detected. Cubic ice was formed on cold substrates from −130° to −160° C. At temperatures below −160° C, a minute form of cubic crystalline ice was detected. It is concluded that ice crystal formation depends on the temperature and the crystal habit of the substrate.
No transformation of the structural form of ice from hexagonal to cubic or cubic to hexagonal was observed when the temperature of the specimens was varied in the range −90° to −180° C. The minute crystalline cubic ice formed below −160° C grew larger at a temperature between −130° and −150° C, displaying the sharp diffraction patterns of cubic ice.
Single ice crystals larger than 0.3 μm in diameter produced diffraction spot patterns. Many ice crystals less than 0.3 μm in diameter produced uniform sharp ring patterns. Ice crystals less than 50 Å in diameter produced halo patterns. The width of the halo pattern increased with the reduction of crystal size.
Cubic ice was formed at temperatures between −130° and −160° C. It is estimated from the width of the diffraction rings that the cubic crystals are from 50 Å to 300 Å in diameter.
The coefficients of linear expansion along the c-axis of the hexagonal ice ranged from 50×10-6 deg−1 to 20×10−6 deg−1, and were almost the same as that of the a-axis in the temperature range from 0° to −180° C. The coefficients of cubical expansion ranged from 140×10−6 deg−1 to 60×10−6 deg−1 at temperatures from 0° to −180° C. The c/a ratio of hexagonal ice sublimed from vapor at temperatures around −100° C was the ideal value of 1.633 for close packing.