Introduction
As one of the leading causes of disability worldwide (Murray et al. Reference Murray, Vos, Lozano, Naghavi, Flaxman, Michaud and Memish2012), depression affects approximately 350 million people each year (Schmaal et al. Reference Schmaal, Hibar, Sämann, Hall, Baune, Jahanshad and Tiemeier2017). Although the neurological substrates of depression are investigated by intensive research studies, the understanding of the pathophysiological mechanism underlying depression remains unclear. As development magnetic resonance imaging (MRI) methods, evidences from structural MRI have consistently pointed to the conclusion that (para-) limbic circuits including dorsomedial prefrontal cortex, orbitofrontal cortex and rostral anterior cingulate cortex (ACC) and the hippocampus presenting structural alteration are involved in the pathophysiological mechanism of depression (Bora, Fornito, Pantelis, & Yücel, Reference Bora, Fornito, Pantelis and Yücel2012; Kempton et al. Reference Kempton, Salvador, Munafò, Geddes, Simmons, Frangou and Williams2011; Schmaal et al. Reference Schmaal, Veltman, van Erp, Sämann, Frodl, Jahanshad and Tiemeier2016). However, these findings are not consistent due to various clinical characteristics such as medicine, recurrence status (first v. recurrent episode), age of onset, especially for illness duration which is thought to be one of the important potential confounders leading to inconsistent findings in depression (Chen et al. Reference Chen, Peng, Sun, Kuang, Li, Jia and Gong2016b). Evidences suggest that depression is a neuroprogressive illness (Morris et al. Reference Morris, Puri, Walker, Maes, Carvalho, Bortolasci and Berk2019; Verduijn et al. Reference Verduijn, Milaneschi, Schoevers, van Hemert, Beekman and Penninx2015) and gray matter volumes (GMVs) are differently altered at different stages according to the length of illness duration (Bora et al. Reference Bora, Fornito, Pantelis and Yücel2012; Serra-Blasco et al. Reference Serra-Blasco, Portella, Gómez-Ansón, de Diego-Adeliño, Vives-Gilabert, Puigdemont and Pérez2013). However, the details about the progressive brain structural alterations as illness advances are still unknown in depression.
Evidences coming from biochemical to brain structural morphological studies convergently indicate that depression is a neuroprogressive illness. Even patients with depression suffering from only one depressive episode also display characteristics of a progressive illness (Moylan, Maes, Wray, & Berk, Reference Moylan, Maes, Wray and Berk2013). Depressive episodes cause brain tissue damage and physiological functioning through a variety of mechanisms underpinning symptomatology and functional decline over time probably resulted from toxic or adaptive effects produced during depressive episodes (Moylan et al. Reference Moylan, Maes, Wray and Berk2013). Patients with longer and more frequent depressive episodes appear to be associated with increased vulnerability to further relapses and functional decline such as increased memory decline and dementia risk (Gorwood, Corruble, Falissard, & Goodwin, Reference Gorwood, Corruble, Falissard and Goodwin2008; Kessing & Andersen, Reference Kessing and Andersen2004). This conclusion is supported from evidences from clinical, biochemical and neuroimaging studies (Moylan et al. Reference Moylan, Maes, Wray and Berk2013; Ruiz, Del Ángel, Olguín, & Silva, Reference Ruiz, Del Ángel, Olguín and Silva2018; Verduijn et al. Reference Verduijn, Milaneschi, Schoevers, van Hemert, Beekman and Penninx2015). In structural neuroimaging studies, gray matter alterations of some crucial regions are found depending on the stage of depression (Alexander-Bloch, Giedd, & Bullmore, Reference Alexander-Bloch, Giedd and Bullmore2013; Frodl et al. Reference Frodl, Meisenzahl, Zetzsche, Born, Jäger, Groll and Möller2003; van Eijndhoven et al. Reference van Eijndhoven, van Wingen, van Oijen, Rijpkema, Goraj, Jan Verkes and Tendolkar2009) and structural changes inconsistently found during the first episode appear more commonly in severe protracted illness (Lorenzetti, Allen, Fornito, & Yücel, Reference Lorenzetti, Allen, Fornito and Yücel2009). One of the most representative brain regions is the hippocampus whose structural alteration is inconsistently found in first-episode patients (Caetano et al. Reference Caetano, Hatch, Brambilla, Sassi, Nicoletti, Mallinger and Soares2004; Campbell, Marriott, Nahmias, & MacQueen, Reference Campbell, Marriott, Nahmias and MacQueen2004; Eker & Gonul, Reference Eker and Gonul2010; Malykhin, Carter, Seres, & Coupland, Reference Malykhin, Carter, Seres and Coupland2010; McKinnon, Yucel, Nazarov, & MacQueen, Reference McKinnon, Yucel, Nazarov and MacQueen2009) but consistently presents in patients with longer illness duration or more frequent episode (McKinnon et al. Reference McKinnon, Yucel, Nazarov and MacQueen2009). Moreover, a relationship between the hippocampal volume decline and longer cumulative illness duration has been described (Sheline, Gado, & Kraemer, Reference Sheline, Gado and Kraemer2003). The same goes for gray matter alterations in rostral ACC and dorsomedial frontal cortex supported from longitudinal work (Frodl et al. Reference Frodl, Koutsouleris, Bottlender, Born, Jäger, Scupin and Meisenzahl2008). Even though many studies attempt to find correlation between illness progression information (such as illness duration) and morphometric alteration (Chen et al. Reference Chen, Shen, Liang, Li, Tyan, Liao and Weng2016a; McKinnon et al. Reference McKinnon, Yucel, Nazarov and MacQueen2009), the progressive structural alteration pattern and details about how initial structural alterations extend to other brain regions remain unknown in depression. Exploring initial structural alterations and the causality between these regions is of great significance for revealing neuroprocessive pathogenesis of depression.
A newly proposed method named causal structural covariance network (CaSCN) analysis (Zhang et al. Reference Zhang, Liao, Xu, Wei, Zhou, Sun and Lu2017) to elucidate the causal relationships of brain structural alterations as illness advances. This method is inspired by Granger causality (GC) analysis commonly used in functional MRI data delineating information flow between time series of two brain regions (Jiang et al. Reference Jiang, Luo, Li, Duan, He, Chen and Yao2018) and conducted by applying the signed path coefficient GC analysis to structural images. In the method, structural images of patients are sequenced in ascending order of illness duration and treated as pseudo-time series describing progressive property of disease (Zhang et al. Reference Zhang, Liao, Xu, Wei, Zhou, Sun and Lu2017). With the help of the CaSCN researchers find original GMV reduction in the hippocampus and the thalamus expert causal effects on prefrontal cortex and other brain regions in epilepsy (Zhang et al. Reference Zhang, Liao, Xu, Wei, Zhou, Sun and Lu2017), important role of the thalamus and frontal lobe in the progression of schizophrenia (Jiang et al. Reference Jiang, Luo, Li, Duan, He, Chen and Yao2018) and progressive pathway originally decreased GMV in the subgenual anterior cortex then propagating to other brain regions such as ventromedial prefrontal cortex and insula in generalized anxiety disorder (Chen et al. Reference Chen, Cui, Fan, Guo, Tang, Sheng and Chen2020). However, the progressive morphological alterations as illness advances and the causal relationship between these structural alterations remain unclear in depression.
In this study, we aimed to investigate GMV alterations at each stage according to illness duration and explore how did initial structural alterations expand to other regions. One hundred ninety-five never-treated first-episode patients with depression and 130 healthy controls (HCs) undergoing T1-weighted MRI scans were included in this study where GMV was quantified using voxel-based morphometry (VBM). We first explored GMV alterations at different stages to assess the gradual changing pattern in depression. This procedure was done to clarify initial and stage-specific structural alterations in depression. Then, regions with GMV alterations at the first stage were treated as seeds for the following CaSCN analysis. This analysis would illustrate how initial alterations spread to other brain regions in depression.
Methods
Participants
Patients with depression were recruited from out-patient/inpatient services of Department of Psychiatry, the First Affiliated Hospital of Zhengzhou University, Zhengzhou, China. One chief physician and one well-trained psychiatrist diagnosed patients according to Diagnostic and Statistical Manual of Mental Disorders, Fourth Edition (DSM-IV) for depression. Inclusion criteria for patients included: (a) First episode and never taking any antidepressants/antipsychotics and any other antidepressant treatment such as psychological therapy and electric shock treatment; (b) No comorbidity of other mental/psychotic disorders; (c) Did not have previous episodes of manic symptoms. All patients were Han Chinese and right handedness. The severity of depression was evaluated using the 24/17-items Hamilton Depression scale (HAMD).
HCs were recruited from the community through poster advertisement. None of them presented a history of serious medical or neuropsychiatric illness or a family history of major psychiatric or neurological illness in their first-degree relatives. All HCs were Han Chinese and right handedness.
In addition, participants (both HCs and patients) in the current study would be excluded it they meet one of the following exclusion criteria: (1) Taking drugs such as anesthesia, sleeping and analgesia in the past 1 month. (2) Substance abuse; (3) A history of brain tumor, trauma, surgery, or other organic body disease; (4) Suffering from cardiovascular diseases, diabetes, hypertension; (5) Contraindications for MRI scanning including fixed dentures, metal braces, artificial heart valves and other metal foreign bodies in the body. (6) Other structural brain abnormalities revealed by MRI scan.
Written informed consents were obtained from all participants before experiment. The study was approved by the research ethical committee of the First Affiliated Hospital of Zhengzhou University.
Data acquisition
T1-weighted anatomical images of participants in dataset 1 were acquired using on 3-Tesla GE Discovery MR750 scanner (General Electric, Fairfield Connecticut, USA). Using an eight-channel prototype quadrature birdcage head coil, we acquired structural T1-weighted images with 3D spoiled gradient echo scan sequence: Repetition time = 8164 ms, echo time = 3.18 ms, inversion time = 900 ms, flip angle = 7 degrees, resolution matrix = 256 × 256, slices =188, thickness = 1.0 mm, and voxel size = 1 × 1 × 1 mm3.
Voxel-based morphometry analysis
All T1 images were processed with stand pipeline steps in the CAT12 toolbox (http://dbm.neuro.uni-jena.de/cat12/). The artifacts of images were checked and the origins of the images were adjusted to the anterior commissure. Images were normalized to Montreal Neurologic Institute space and segmented into gray matter, white matter and cerebrospinal areas. Then images were resampled to a volume image resolution of 1.5 × 1.5 × 1.5 mm3. Finally, the gray matter maps were smoothed using 6 mm full-width at half-maximum Gaussian kernel (FWHM). The total intracranial volume (TIV) of each participant was also calculated for the next comparative analysis.
Overall and stage-specific GMV alteration in depression
First, two sample t test was employed to examine overall GMV aberrance and stage-specific GMV aberrance in depression. Overall GMV aberrance was obtained by comparing GMV of all patients with depression with that in matched HCs. Stage-specific GMV aberrance was obtained by subgroup of patients with depression according to illness duration with that in matched HCs. As there was no acknowledged strategy dividing patients into subgroups, we arbitrarily divided patients into three stages (stage 1: <12 months; stage 2: 12–24 months; stage 3: > = 24 months) according to illness duration distribution of patients in the current study. At each stage, the GMV maps in patients were compared with that in HCs matched for gender, age and education level using two sample t test. Moreover, to explore whether aberrance of GMV occurred at illness onset, we also explored altered GMVs in patients with shorter illness duration (<3/6 months). In this procedure, age, gender and educational level were treated as covariates. Because we had used nonlinearly modulated gray matter maps in the preprocessing, the voxel values were already corrected for individual brain sizes. As done in previous study, we did not include total brain volume as an additional covariate (Bludau et al. Reference Bludau, Bzdok, Gruber, Kohn, Riedl, Sorg and Eickhoff2016).
Results reported were corrected for multiple comparison (voxel-wise p < 0.005, cluster-level p < 0.05; Gaussian random field (GRF) corrected). The mean GMVs of each peak coordinate of statistical results with spherical radius of 4 mm were extracted at each stage/across stages (for overall aberrance) for the following steps. We also compared the TIV of patients with that of HCs at each stage and across stages to explore whether TIV was altered in patients with depression.
Association between GMV alteration and clinical characteristics
Then, we explored correlation between clinical characteristics such as gender, age of onset with the GMV alteration in depression. Specially, to explore the effect of gender on the GMV alteration, we divided patients into male and female groups. Peak coordinates of GMV alteration were identified in previous steps. The mean GMVs of each peak coordinate with spherical radius of 6 mm were calculated and compared between female and male patients. As for the age of onset, because the number of patients whose age of onset were older than 21/25 years, the critical age for distinguishing between adolescent onset patients and adult onset patients in depression (Penttilä et al. Reference Penttilä, Cachia, Martinot, Ringuenet, Wessa, Houenou and Paillère-Martinot2009; Truong et al. Reference Truong, Minuzzi, Soares, Frey, Evans, MacQueen and Hall2013), was only 33/15, we just calculated the Pearson correlation between the mean GMVs and age of onset in patients. In addition, the Pearson correlation between the mean GMVs and illness duration was also calculated. Note the aforementioned steps (statistical and correlation analysis) were done at each stage and across all stages.
Seed-based CaSCN analysis
The CsSCN was performed as done in the previous studies (Jiang et al. Reference Jiang, Luo, Li, Duan, He, Chen and Yao2018; Zhang et al. Reference Zhang, Liao, Xu, Wei, Zhou, Sun and Lu2017), the GMV data of all patients were first ranked in ascending order of illness duration. The data sequencing of GMV was granted as time-series information for characterizing the progressive property of depression. Then, seed-based CsSCN similar to signed-path coefficient GC analysis applied in functional MRI data analysis was constructed. The seed regions were selected from VBM analysis at the beginning of the illness (illness duration <3 months). The signed-path coefficient GC analysis implemented in REST (http://www.restfmri.net) was done to measure directed influences between GMV alteration of the seed regions on that of other voxels in gray matter or the other way around. The positive GC value indicated the same GMV alteration in the voxels lagged behind the seed region or driven by the seed region while the negative GC value denoted an opposite alteration lagged behind the seed region implying a compensatory effect (Jiang et al. Reference Jiang, Luo, Li, Duan, He, Chen and Yao2018; Zhang et al. Reference Zhang, Liao, Xu, Wei, Zhou, Sun and Lu2017). Age and gender were treated as covariates in conducting CsSCN analysis. Based on the presumption that the GMV alteration was the origination of pathological alteration of brain structures in depression, we only adopted GC value of the seed region on the other voxels in gray matter (Zhang et al. Reference Zhang, Liao, Xu, Wei, Zhou, Sun and Lu2017). The GC maps were further transformed into a z score and the results were corrected with Bonferroni correction (where voxel p < 0.05 and corresponding Z > 5.3).
Results
Clinical demographics
The clinical demographics were presented in online Supplementary Table S1. To exclude the effects of covariates such as age and gender on the comparative results, GMV alteration was determined by comparing GMVs between patients at different stages with that in age-, gender-, education level-matched HCs. The clinical demographics of patients at different stages and matched HCs were in online Supplementary Table S2.
Overall GMV alterations in depression
Overall, patients with depression presented decreased GMVs in the bilateral hippocampus extending to the thalamus and cerebellum while increased GMVs in regions including the medial frontal gyrus parahippocampa gyrus, amygdala, ventral portions of the ventral medial prefrontal cortex (vmPFC) and the right precuneus. The results reported were corrected for multiple comparison (voxel-wise p < 0.005, cluster-level p < 0.05; GRF corrected) (Fig. 1 and online Supplementary Table S3).
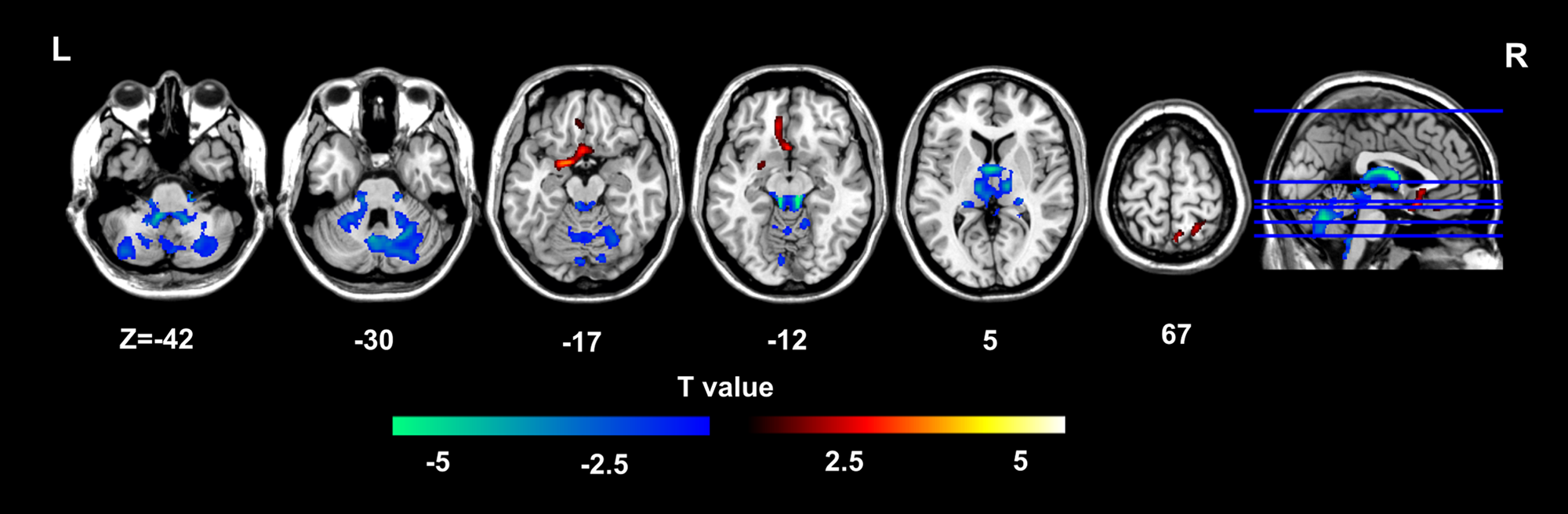
Fig. 1. Overall GMV alterations in depression.
Different GMV alterations at different stages in depression
Whereafter, we investigated gradual structural alterations at different stages according to illness duration in depression. Patients with depression were divided into three stages (stage 1: 0–12 months; stage 2: 12–24 months; stage 3: > = 24 months) according to illness duration. By comparing GMVs in patients with that in matched HCs, we found stage-specific structural alteration in depression. Specifically, patients presented decreased GMVs in the bilateral hippocampus extending to the thalamus and cerebellum, while increased GMVs in the sgACC/vmPFC at the first stage (< 12 months). At the second stage, GMVs of the thalamus were found decreased while that in regions such as right insula extending to the right amygdala and the right putamen, orbital frontal gyrus and dorsal medial frontal gyrus extending to ACC were found increased in patients with depression. At the third stage, patients presented overall decreased GMV in regions including the thalamus, the hippocampus and the cerebellum (Fig. 2, online Supplementary Table S4). Considering that whether structural alterations in critical regions such as medial frontal gyrus, the hippocampus and the amygdala occurred at the onset of illness was still controversial, we also investigated GMV alteration in patients shorter illness duration (<3/6 months) (Belleau, Treadway, & Pizzagalli, Reference Belleau, Treadway and Pizzagalli2019; Bremner et al. Reference Bremner, Narayan, Anderson, Staib, Miller and Charney2000; Mervaala et al. Reference Mervaala, Föhr, Könönen, Valkonen-Korhonen, Vainio, Partanen and Lehtonen2000; Sheline, Gado, & Price, Reference Sheline, Gado and Price1998; Treadway et al. Reference Treadway, Waskom, Dillon, Holmes, Park, Chakravarty and Pizzagalli2015). As a result, GMV reduction was found in the hippocampus and the thalamus and GMV elevation in the sgACC/vmPFC were found in patients with illness less than 3 months. Additional GMV reduction in the cerebellum was found in patients with illness less than 6 months (Fig. 3 and online Supplementary Table S5). We did not observe significant difference of TIV between patients and HCs at each stage and across stages.

Fig. 2. Different GMV alterations at different stages in depression.

Fig. 3. Altered GMVs in patients with shorter illness duration (< 3/6 months).
Association with clinical characteristics
To explore the effect of gender on the GMV alteration, we compared mean GMVs of each peak coordinate with spherical radius of 4 mm between female and male patients using two sample t test at each stage and across stages. There was no significant difference in GMVs between male and female patients with depression. Then, we explored the Pearson correlation between the mean GMVs of each peak coordinate with spherical radius of 4 mm and illness duration/age of onset at each stage and across stages. Overall, the mean GMVs of the thalamus was significantly correlated with age of onset (R = −0.208, p = 0.004) and the mean GMVs of the vmPFC was correlated with illness duration (R = −0.188, p = 0.008) across stage. At the first stage, the mean GMVs of left hippocampus was correlated with illness duration (R = −0.271, p = 0.011) (online Supplementary Fig. S1).
Causal effects of GMV alteration pattern in depression
To map the causal effects of progressive GMV alteration in patients with depression, the CsSCN was constructed with seminal structural alterations at the onset of the illness (illness duration <3 months). The bilateral hippocampus (MNI: 30, −35, 9 for the right, −32, −33, −9 for the left) and the sgACC/vmPFC (MNI: −2, 18, −12) were selected as seeds for CsSCN analysis. The positive/negative GC values represented same/opposite GMV alterations in the regions occurring behind the seed region (Jiang et al. Reference Jiang, Luo, Li, Duan, He, Chen and Yao2018; Zhang et al. Reference Zhang, Liao, Xu, Wei, Zhou, Sun and Lu2017). As a result, the sgACC/vmPFC presented positive GC values on the bilateral nucleus accumbens (NAc), the right ACC, the inferior frontal gyrus and the cerebellum and while negative GC values on the left precuneus extending to the calcarine, and the left rolandic operculum (Fig. 4, online Supplementary Table S6). Positive GC values were found from the left hippocampus to the right precuneus extending to calcarine, the bilateral NAc and the bilateral middle occipital gyrus and negative GC values to the left inferior parietal lobe and right inferior frontal gyrus (Fig. 4). As for the right hippocampus, only positive GC values were found in the bilateral dorsolateral frontal gyrus (DLPFC), right ACC, bilateral precuneus and the cerebellum (Fig. 4). The summary of the CsSCN analysis was presented in Fig. 5, the GC values were included in online Supplementary Table S7.
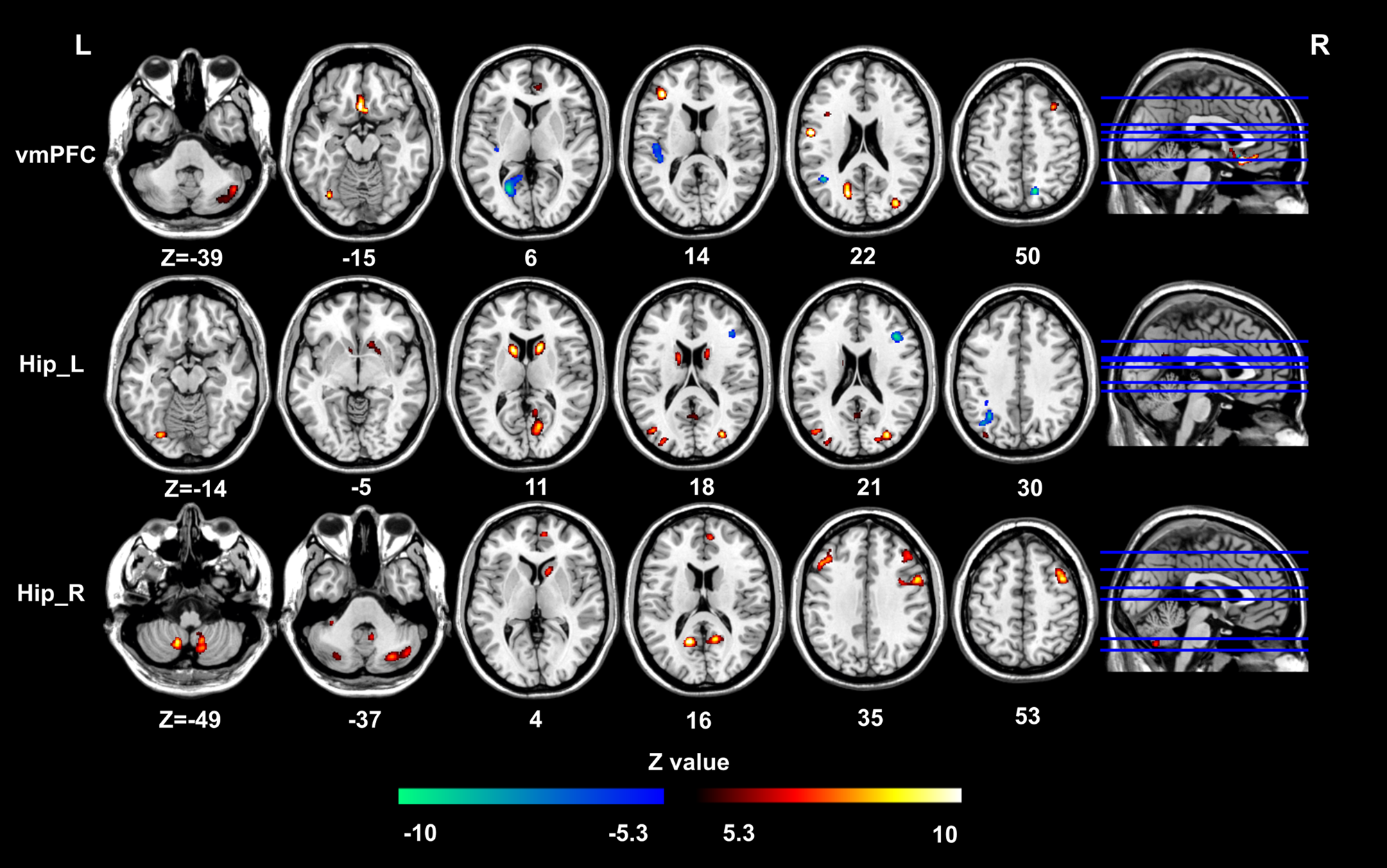
Fig. 4. Causal effects of GMV alterations pattern in depression.

Fig. 5. Summary of the CsSCN analysis. The red arrow represented positive GC values and the negative ones represented negative GC values.
Discussion
In this study, we investigated stage-specific morphometric alterations measured with VBM and how these alterations extended to other brain regions as illness advanced. At the first stage (illness duration <12 months), patients with depression were companied with GMV increase in vmPFC while GMV reduction in the bilateral hippocampus and the thalamus. Then as the illness duration was prolonged, GMV alterations spread to other brain regions including the NAc, orbital (and medial) frontal gyrus, the amygdala, the putamen, precuneus and the cerebellum. These results elaborated stage-specific GMV alterations pattern in depression. The CaSCN results revealed causal effect of the bilateral hippocampus and the vmPFC on other brain regions. Specifically, the bilateral hippocampus and the vmPFC corporately exerted causal effect on NAc, the precuneus and the cerebellum. GMV alterations in the hippocampus were also potentially causally related to that in the DLPFC. Consistent with aforesaid stage-specific GMV alteration pattern, these results revealed expanding pattern of GMV alterations from the hippocampus and the vmPFC to other brain regions. In general, our results revealed progressive morphological alteration stemming from and further elucidated possible details about disease progression in depression.
The stage-specific morphological alterations revealed the progressive alteration pattern spreading from original alterations in regions including the hippocampus, the thalamus and the sgACC/vmPFC to other regions such as amygdala, dorsal medial frontal gyrus and NAc. Converging evidences suggested that depression was a neuroprogressive illness (Kendler, Thornton, & Gardner, Reference Kendler, Thornton and Gardner2001; Moylan et al. Reference Moylan, Maes, Wray and Berk2013), the morphometric alteration of critical brain regions was related to illness progression information (Alexander-Bloch et al. Reference Alexander-Bloch, Giedd and Bullmore2013; Chen et al. Reference Chen, Shen, Liang, Li, Tyan, Liao and Weng2016a; Frodl et al. Reference Frodl, Meisenzahl, Zetzsche, Born, Jäger, Groll and Möller2003; McKinnon et al. Reference McKinnon, Yucel, Nazarov and MacQueen2009; van Eijndhoven et al. Reference van Eijndhoven, van Wingen, van Oijen, Rijpkema, Goraj, Jan Verkes and Tendolkar2009). Consistent with these studies, we observed stage-specific GMV alterations in never-treated first-episode patients with depression. Specially, the GMVs of the hippocampus and the vmPFC were found altered at the onset of illness. Whether GMV alterations in critical brain regions such as the hippocampus, the vmPFC and the amygdala was still in dispute (Schmaal et al. Reference Schmaal, Veltman, van Erp, Sämann, Frodl, Jahanshad and Tiemeier2016, Reference Schmaal, Hibar, Sämann, Hall, Baune, Jahanshad and Tiemeier2017) that might be resulted from illness duration (Chen et al. Reference Chen, Peng, Sun, Kuang, Li, Jia and Gong2016b). Progressive GMV alterations of the hippocampus, the vmPFC and the amygdala (Belleau et al. Reference Belleau, Treadway and Pizzagalli2019; Bora et al. Reference Bora, Fornito, Pantelis and Yücel2012) were related to chronic stress and toxic effects of recurrent depressive episodes (Treadway et al. Reference Treadway, Waskom, Dillon, Holmes, Park, Chakravarty and Pizzagalli2015). Our results suggested that structural alterations of these regions occurred at the onset of the illness. In fact, patients with longer (than the current study) illness duration presented decreased GMVs (Coryell, Nopoulos, Drevets, Wilson, & Andreasen, Reference Coryell, Nopoulos, Drevets, Wilson and Andreasen2005; Klauser et al. Reference Klauser, Fornito, Lorenzetti, Davey, Dwyer, Allen and Yücel2015; Yucel et al. Reference Yucel, McKinnon, Chahal, Taylor, Macdonald, Joffe and MacQueen2008) suggesting illness duration burden-mediated alterations in this region. In all, these results confirmed the notion that depression was a neuroprogressive illness and structural alterations of the hippocampus and the vmPFC occurred at the onset of the illness.
At the second stage, we found transient increase of GMVs in regions such as DLPFC, insula, and dorsal medial frontal gyrus. At the third stage, GMVs of these regions presented insignificant reduction. The possible explanation might be pre-apoptotic osmotic changes (Adler, Levine, DelBello, & Strakowski, Reference Adler, Levine, DelBello and Strakowski2005; Yucel et al. Reference Yucel, McKinnon, Chahal, Taylor, Macdonald, Joffe and Macqueen2009) in depression. Both decreased (Kandilarova, Stoyanov, Sirakov, & Maes, Reference Kandilarova, Stoyanov, Sirakov and Maes2019; Vasic, Walter, Höse, & Wolf, Reference Vasic, Walter, Höse and Wolf2008; Wise, Radua, Via, Cardoner, & Abe, Reference Wise, Radua, Via, Cardoner and Abe2017) and increased (Adler et al. Reference Adler, Levine, DelBello and Strakowski2005; Brambilla et al. Reference Brambilla, Nicoletti, Harenski, Sassi, Mallinger, Frank and Soares2002; Straub et al. Reference Straub, Brown, Malejko, Bonenberger, Grön, Plener and Abler2019; van Eijndhoven et al. Reference van Eijndhoven, van Wingen, Katzenbauer, Groen, Tepest, Fernández and Tendolkar2013; Yucel et al. Reference Yucel, McKinnon, Chahal, Taylor, Macdonald, Joffe and Macqueen2009) GMVs were found in these regions, we noticed that reduced GMVs of these regions were found in patients with much longer illness duration than the current study. Combining the findings that stress-mediated neurotoxic processes resulted in progressive volumetric reductions in these regions (Ansell, Rando, Tuit, Guarnaccia, & Sinha, Reference Ansell, Rando, Tuit, Guarnaccia and Sinha2012; Bora et al. Reference Bora, Fornito, Pantelis and Yücel2012; Hatton et al. Reference Hatton, Franz, Elman, Panizzon, Hagler, Fennema-Notestine and Kremen2018), GMV reductions would be found in patients with longer illness duration. However, this conclusion might be treated carefully, as there was no study investigating structural abnormality in depression with the same specific interval of illness duration. At the third stage, the GMV of these regions began to exhibit decreasing trend in patients with longer illness duration (online Supplementary Fig. S2). However, the limited sample size and the not enough length of illness duration might restrict the statistical power to detect GMV reductions in these regions. Combined with GMV alterations at the first stage, these results elucidated the stage-specific GMV alterations pattern in depression.
The CaSCN analysis revealed causal relationship between initial GMV alterations in the hippocampus and the vmPFC and that in other brain regions. The vmPPFC was implicated in a variety of social, cognitive and affective functions that were disrupted in depression (Hiser & Koenigs, Reference Hiser and Koenigs2018). Specially, the vmPFC was in charge of the generation and regulation for negative emotions by inhibition of the amygdala (Likhtik, Pelletier, Paz, & Paré, Reference Likhtik, Pelletier, Paz and Paré2005; Quirk, Likhtik, Pelletier, & Paré, Reference Quirk, Likhtik, Pelletier and Paré2003; Rosenkranz, Moore, & Grace, Reference Rosenkranz, Moore and Grace2003), representation of reward/value-based decision making through its interaction with the ventral striatum (Christakou, Robbins, & Everitt, Reference Christakou, Robbins and Everitt2004; Richard & Berridge, Reference Richard and Berridge2013), processing of self-relevant information and social cognition by interacting with default mode network (DMN) and temporoparietal cortex (Hiser & Koenigs, Reference Hiser and Koenigs2018; Raichle, Reference Raichle2015). Sustained negative affect and impairment of emotional regulation were hallmark features in depression (Joormann & Stanton, Reference Joormann and Stanton2016). Prior GMV alterations in the vmPPFC then followed by GMV alterations in the amygdala might be associated with emotional regulation dysfunctions in depression. Although we did not find direct linear causal effect from GMVs alterations in the vmPPFC on that in the amygdala, the reason might be that the causal relationship between the two regions was nonlinear or independent. The altered prediction error with reinforcement learning theories was hypothesized to underlie the anhedonia in depression (Gradin et al. Reference Gradin, Kumar, Waiter, Ahearn, Stickle, Milders and Steele2011). NAc and its connected regions including vmPPFC played an important role in motivated decision making and encoding of prediction errors in depression (Christoffel et al. Reference Christoffel, Golden, Walsh, Guise, Mitra, Friedman and Madeline2015; Han et al. Reference Han, Cui, Wang, Chen, Li, Li and Chen2020a; Stuber et al. Reference Stuber, Sparta, Stamatakis, van Leeuwen, Hardjoprajitno, Cho and Bonci2011). Consistent with studies, we observed significant causal effect of the GMV alterations in the vmPPFC on the that in the NAc and middle temporal cortex. In addition, we also found causal relationship between the GMV alterations in the vmPPFC on that in the precuneus. As an important part of DMN, dysfunctions of the precuneus were related to the rumination about low self-esteem in depression (Cheng et al. Reference Cheng, Rolls, Qiu, Yang, Ruan, Wei and Feng2018; Han et al. Reference Han, Cui, Wang, Li, Li, He and Guo2020b). Taken together, these results suggested that clinical symptoms including emotional regulation especially for sustained negative affect, aberrant reward processing and rumination related GMV aberrance might originate from structural aberrance in the vmPPFC in depression.
The causal effects of the GMVs of the bilateral hippocampus on that of the DLPFC, the precuneus, NAc and the cerebellum were identified in the current study. Receiving dense glutamatergic projections from the hippocampus and the medial PFC (Friedman, Aggleton, & Saunders, Reference Friedman, Aggleton and Saunders2002; Phillipson & Griffiths, Reference Phillipson and Griffiths1985), NAc and its structural covariance network were found to be responsible for the anhedonia in depression (Han et al. Reference Han, Cui, Wang, Chen, Li, Li and Chen2020a). Especially, the hippocampus input was particularly capable of stably depolarizing NAc neurons, allowing prefrontal cortex input to generate spike firing in these cells (Friedman et al. Reference Friedman, Aggleton and Saunders2002) and contributing to the proper regulation of goal-directed behaviors (LeGates et al. Reference LeGates, Kvarta, Tooley, Francis, Lobo, Creed and Thompson2018). In addition to vmPFC, the GMV alterations of the bilateral hippocampus also exerted causal effect on that in the NAc, these results suggested the vmPFC and the hippocampus jointly facilitated the GMV alterations in the NAc. The conclusion held true for the GMV alterations in the precuneus. Recent studies suggested that DLPFC receiving monosynaptic projections from the hippocampus (Barbas & Blatt, Reference Barbas and Blatt1995; Hoover & Vertes, Reference Hoover and Vertes2007), was recruited during the regulation of negative emotion through reappraisal/suppression strategies (Eippert et al. Reference Eippert, Veit, Weiskopf, Erb, Birbaumer and Anders2007; Lévesque et al. Reference Lévesque, Eugène, Joanette, Paquette, Mensour, Beaudoin and Beauregard2003; Ochsner et al. Reference Ochsner, Ray, Cooper, Robertson, Chopra, Gabrieli and Gross2004; Ochsner, Bunge, Gross, & Gabrieli, Reference Ochsner, Bunge, Gross and Gabrieli2002; Phan et al. Reference Phan, Fitzgerald, Nathan, Moore, Uhde and Tancer2005). Its (both anatomical and functional) interactions with the hippocampus played a central role in various behavioral and cognitive functions and were disturbed in psychiatric disease (Sigurdsson & Duvarci, Reference Sigurdsson and Duvarci2015). Negative stimuli, such as stress and cognitive impairment resulted in dysfunction of neural plasticity in the hippocampus then that of the DPLFC in depression (Kuhn et al. Reference Kuhn, Höger, Feige, Blechert, Normann and Nissen2014). Recent years, the cerebellum draw researcher's attention for its anatomical connections with the limbic regions involved in mood regulation (Turner et al. Reference Turner, Paradiso, Marvel, Pierson, Boles Ponto, Hichwa and Robinson2007) and important role in emotion and cognitive processing (Moulton et al. Reference Moulton, Elman, Pendse, Schmahmann, Becerra and Borsook2011; O'Reilly, Beckmann, Tomassini, Ramnani, & Johansen-Berg, Reference O'Reilly, Beckmann, Tomassini, Ramnani and Johansen-Berg2010). The interaction between the cerebellum and regions especially the hippocampus was suggested to be central to depression (Steinke et al. Reference Steinke, Gaser, Langbein, Dietzek, Gussew, Reichenbach and Nenadić2017). The hippocampal glutamate/glutamine related to cognitive functions (Ende, Reference Ende2015), was found linked to the cerebellar function (Steinke et al. Reference Steinke, Gaser, Langbein, Dietzek, Gussew, Reichenbach and Nenadić2017). Our results suggested that the decreased GMVs in the cerebellum might be originated from GMV alterations in the hippocampus.
We also explored the association between the GMV alterations with clinical characteristics. Consistent with hypothesis that depression was a neuroprogressive illness, GMVs in the vmPFC were positively correlated with illness duration across stages, reflecting the effect of the cumulative adversity and stress on this region (Ansell et al. Reference Ansell, Rando, Tuit, Guarnaccia and Sinha2012). As for the hippocampus, we observed negative correlation between the GMVs with illness duration at first stage. The negative correlation between GMVs in the hippocampus and the thalamus with age of onset embodied effect of illness onset on the functional and structural alterations in depression (Ancelin et al. Reference Ancelin, Carrière, Artero, Maller, Meslin, Ritchie and Chaudieu2019; Brown, Clark, Hassel, MacQueen, & Ramasubbu, Reference Brown, Clark, Hassel, MacQueen and Ramasubbu2019). In the current study, we observed the negative correlation between GMV in the hippocampus and the thalamus and age of onset. Taken together, our results elaborated that GMVs of regions like the thalamus were related to various clinical factors, explaining the possible reason for the inconsonant GMVs results in depression.
Several limitations must be considered in the current study. First, conclusions drawn in this study come from a single dataset, another independent dataset was needed to validate our results in the future. Second, the age of patients enrolled was less than 36 years, whether conclusions held are true for late-onset depression need to be explored (Penttilä et al. Reference Penttilä, Cachia, Martinot, Ringuenet, Wessa, Houenou and Paillère-Martinot2009; Truong et al. Reference Truong, Minuzzi, Soares, Frey, Evans, MacQueen and Hall2013). Third, the CaSCN results could not directly reflect the real sequence of morphological changes, longitudinal studies were needed to further clarify the causal relationship between morphological changes in depression. Fourth, other clinical variables such as severity of depression that might also be related to brain structural alterations could be considered in future. Fifth, we did not consider the causal effect between the GMV alteration in the following brain regions since the second stage.
Conclusion
We explored initial GMV alterations, progressive structural alteration pattern and causal relationships of them using CaSCN analysis in never-treated first-episode patients with depression in this study. Our results revealed progressive structural alteration pattern in depression. Specially, patients with depression were accompanied with GMV increase in vmPFC while GMV reduction in the bilateral hippocampus and the thalamus at the first stage. Then GMV alterations spread to regions such as NAc, orbital (and medial) frontal gyrus, amygdala, the putamen, the precuneus and the cerebellum. The GMV alterations in the bilateral hippocampus and the vmPFC jointly or separately exerted causal effects on that in the following brain regions, hinting their crucial roles in the disease progression. Consistent with the neuroprogressive hypothesis, our results revealed progressive morphological alterations originating from the vmPFC and the hippocampus and elucidated possible details about disease progression of depression.
Supplementary material
The supplementary material for this article can be found at https://doi.org/10.1017/S0033291721003986.
Acknowledgements
The authors thank all subjects who participated in this study.
Financial support
This research study was supported by the Natural Science Foundation of China (81601467, 81871327, 6210020612) and Medical Science and Technology research project of Henan province (201701011).
Conflict of interest
All authors declared no conflict of interest.