Assessment of the left ventricle, regarding both size and function, was one of the earliest targets of clinical echocardiographyReference Picard, Popp and Weyman1 as it has always been important for evaluating patients with suspected or proven cardiac disease, as well as guiding their management and anticipating their prognosis.Reference Luis, Chan and Pellikka2 Recent guidelines no longer recommend using linear ventricular dimensions to measure the left ventricular ejection fraction as it can easily miss regional dysfunction,Reference Lang, Badano and Mor-Avi3 in addition to the inter- and intra-observer variability and inaccuracies encountered when meticulous delineation of the endocardial border is difficult and also in cases with hypertrophied ventricles.Reference Mirea, Pagourelias and Duchenne4 Limitations of the other methodologies used for the evaluation of ventricular function such as Doppler assessment of the stroke volume and modified Simpson biplane method had also been reported.Reference Luis, Chan and Pellikka2
Imaging of myocardial deformation with the measurement of the strain and strain rate has been introduced as reliable methods to detect regional left ventricular contractility.Reference Pellerin, Sharma and Elliott5 Strain is a dimensionless quantity of myocardial deformation expressed in percent (%). It represents the change in the myocardial fibre length during stress (end systole) compared to its original length in the relaxed state (end diastole), whereas the strain rate is the change of strain per unit time and it represents the speed of deformation of the myocardial fibre. Ventricular fibres shorten in the longitudinal direction from base to apex and in the circumferential direction perpendicular to the radial and longitudinal axes, whereas it thickens in the radial direction perpendicular to the epicardium and to the longitudinal axis. Negative strain values denote shortening or thinning, whereas positive values indicate lengthening or thickening.Reference Pavlopoulos and Nihoyannopoulos6
The clinical use of tissue Doppler-derived strain measurements was limited by the angle dependency, long exhausting analysis time, inter- and intra-observer variability,Reference Geyer, Caracciolo and Abe7 and the presence of noise artifacts.Reference Urheim, Edvardsen and Torp8 Two-dimensional speckle tracking echocardiography is a relatively new non-invasive imaging modality that has emerged recently for more accurate assessment of myocardial deformation.Reference Leitman, Lysyansky and Sidenko9 It is a non-Doppler, largely angle-independent modality that is capable of objective evaluation of both global and regional ventricular function.Reference Blessberger and Binder10 It is technically based on the analysis of the motion of speckles (spots generated from the interaction between the myocardial fibres and the ultrasonic beam) on routine B-mode images. By tracking the displacement of these speckles, the strain and strain rate can be measured during the cardiac cycle.Reference Mondillo, Galderisi and Mele11
The clinical importance of evaluating the deformation parameters using two-dimensional speckle tracking echocardiography, in addition to the easy mode of assessment, has sparked great passion among the echocardiographic community towards exploring this modality, which is reflected by the increasing number of publications that focus on all aspects of speckle tracking echocardiography and test its potential clinical utility in the evaluation of clinical and subclinical cardiovascular diseases.Reference Blessberger and Binder10
The definition of normal values for two-dimensional speckle tracking echocardiography derived left ventricular systolic global strain is crucial for proper clinical application of this relatively new modality.Reference Yingchoncharoen, Agarwal and Popovic12 Marking an abnormal strain value is not as easy as it might seem as a variety of demographic, haemodynamic, and anthropometric parameters might potentially affect the measurements of the strain.Reference Marwick, Leano and Brown13 Unfortunately, reference values for these measurements are still relatively scarce in the paediatric population.Reference Marcus, Mavinkurve-Groothuis and Barends14 Also, inter-vendor variability of the two-dimensional strain assessment do exist when using different softwares.Reference Nagata, Takeuchi and Mizukoshi15 We believe that more studies are needed to be done on larger scales in children that should include different paediatric populations with different ethnicities using different machine vendors and softwares that helps in future comparability between them. Hence, the aim of this study was to set reference values for both global and regional two-dimensional speckle tracking echocardiography-derived peak longitudinal systolic strain in healthy Egyptian children, who belong to the North African ethnicity, using automated functional imaging and to study the correlation between the global peak longitudinal left ventricular systolic strain and age, anthropometric measurements, vital data, and some echocardiographic parameters.
Patients and methods
This is a cross-sectional study that was conducted at the Echocardiography lab, paediatric cardiology unit, Children Hospital, Ain Shams University. It included 250 healthy children (141 males and 109 females) who were recruited in two ways: one hundred and ninety three children were referred to the Echo lab for innocent murmurs and 57 children were the healthy siblings of cardiac patients following up in the paediatric cardiology clinic. Their ages ranged from 1 to 16 years with a mean and SD of 9.24 ± 4.33 years. Based on their ages, the study population was categorised into four groups of nearly equal sizes. Detailed medical history was taken as well as thorough clinical examination to ensure that all children were free from any cardiovascular diseases or any other medical illnesses of clinical significance. Vital data were taken (heart rate and blood pressure), and anthropometric measurements were assessed (weight, height, body mass index, and the body surface area using the Costeff formula).Reference Costeff16
Transthoracic echocardiography
Echocardiographic examinations were done using the vivid E9 machine (GE Vingmed ultrasound N-3191, Horton, Norway) and the M5S-D Probe (S/N 00000 2891, Portugal). Conventional transthoracic echocardiography using two-dimensional, colour flow, continuous, and pulsed wave Doppler modalities was performed in accordance to the American Society of Echocardiography pediatric guidelinesReference Lai, Geva and Shirali17 to rule out any clinically undiagnosed congenital or acquired heart disease. M-mode echocardiographic assessment was done in accordance to the American Society of Echocardiography adult guidelinesReference Lang, Bierig and Devereux18 using the parasternal short-axis view to measure the left ventricular dimensions and function at the mid-papillary level and to measure the aortic and left atrial dimensions at their corresponding levels.
Two-dimensional speckle tracking echocardiography
Acquisition of the two-dimensional speckle images was obtained via an automated functional imaging software (GE health care) integrated into the Echo machine. The left ventricular longitudinal strain was assessed by obtaining electrocardiogram-gated high-quality images for the left ventricle at frame rates of 60 to 90 per second in the standard three views (four chamber, apical long-axis three chamber, and apical two chamber). Tracking of the endocardial border of the myocardium was done automatically to delineate a region of interest that was discarded automatically by the software when inadequately tracked. The left ventricular longitudinal strain was assessed in 18 segments (six segments for each view). The apical four-chamber view assessed the septal and the lateral walls. Each wall was divided into apical, mid, and basal (basal septal, mid septal, apical septal, basal lateral, mid lateral, and apical lateral). The apical two-chamber view assessed the inferior and the anterior walls. Each wall was divided into apical, mid, and basal (basal inferior, mid inferior, apical inferior, basal anterior, mid anterior, and apical anterior). The apical long-axis view assessed the posterior and the anteroseptal walls. Each wall was divided into apical, mid, and basal (basal posterior, mid posterior, apical posterior, basal anteroseptal, mid anteroseptal, and apical anteroseptal).
For each of the standard views, the global peak systolic longitudinal strain was obtained that represented the average strain results of the six segments, then the averaged global peak longitudinal strain was automatically displayed which represented the average strain of the three previous averages as well as the colour-coded representation of the longitudinal stain (Bull’s eye map).
The analysis was done on-line by a single experienced echocardiographer (Al-Fahham MM).
Statistical analysis
Statistical analysis was performed using IBM SPSS version 20.0, Armonk, NY: IBM Corp. Quantitative data were expressed as mean ± standard deviation. Qualitative data were expressed as frequency and percentage. Independent-samples t-test was used to compare between two means and ANOVA test (one-way analysis of variance) to compare more than two means. Post hoc test was used for comparison of all possible pairs of group means. Chi square test (X2) was used to detect the relationship between two qualitative variables. Correlation between variables was done using Pearson correlation coefficient (r). P-value of ≤ 0.05 was considered significant and ≤ 0.01 was considered highly significant.
The intra- and inter-observer variability was determined by re-assessment of the left ventricular longitudinal strain in 25 randomly recruited children. To measure the intra-observer variability, the same echocardiographer (Al-Fahham MM) assessed the myocardial strain after 1 month to avoid possible recall bias. To assess for the inter-observer variability, the strain measurements were analysed by another observer who was completely blinded to the results of the first observer.
The degree of intra- and inter-observer variability was defined based on the coefficient of variation and Alpha Cronbach’s. The reliability was scaled as follows: values < 0.25 indicates weak reliability, 0.25 – 0.75 indicates moderate reliability, and 0.75 – < 1 indicates strong reliability and 1 is optimum.
Results
Demographic characteristics and vital data of the studied population are shown in Table 1. There were no statistically significant differences between the studied groups as regard to gender distribution (X2 = 3.771, p = 0.287). Left ventricular dimensions and functions of the studied population are shown in Table 2.
Table 1. Demographic and vital data of the studied population
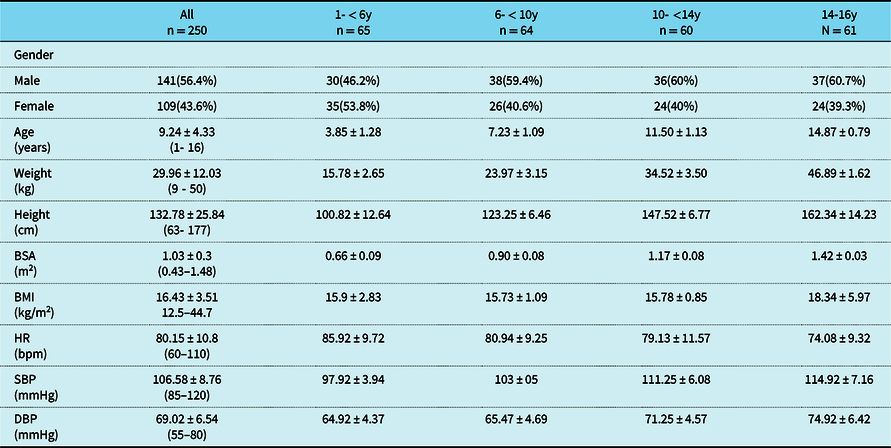
BSA: body surface area; BMI: body mass index; DBP: diastolic blood pressure; HR: heart rate; SBP: systolic blood pressure
Table 2. Left ventricular dimensions and function of the studied population
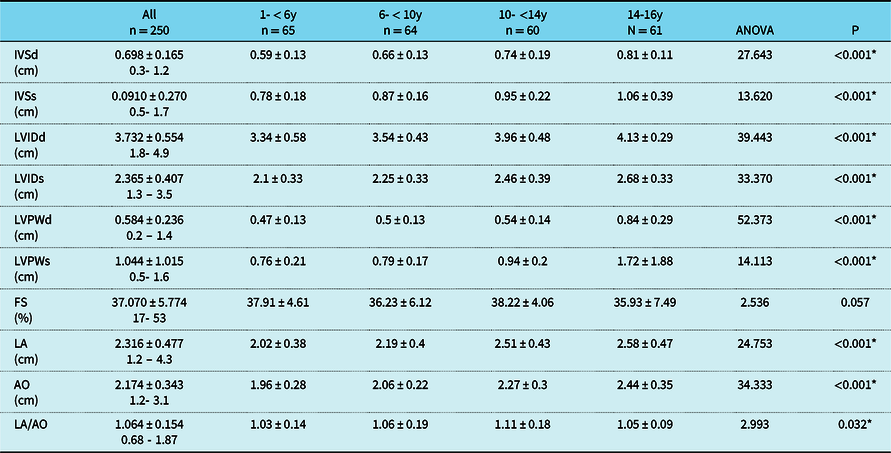
Ao: aorta; FS: fractional shortening; IVSd: interventricular septum at diastole; IVSs: interventricular septum at systole; LVIDd: left ventricular internal diameter at diastole; LVIDs: left ventricular internal diameter at systole; LVPWd: left ventricular posterior wall at diastole; LVPWs: left ventricular posterior wall at systole; LA: left atrium
Among the studied population, the global peak longitudinal systolic strain at the apical long-axis view ranged from −28% to −17% with a mean and standard deviation of −20.592 ± 2.437%, the global peak longitudinal systolic strain at the apical four-chamber view ranged from −28% to −15% with a mean and standard deviation of −20.952 ± 2.254%, the global peak longitudinal systolic strain at the apical two-chamber view ranged from −32 % to −16% with a mean and standard deviation of −22.232 ± 2.468%, and the averaged global peak longitudinal systolic strain ranged from −27% to −17% with a mean and SD of −21.224 ± 1.862%.
The mean values of the systolic longitudinal strain become significantly more negative from base to apex (Table 3).
Table 3. Comparison between the averaged LV systolic longitudinal strain of the three LV segments (apical, mid-segment, and basal)
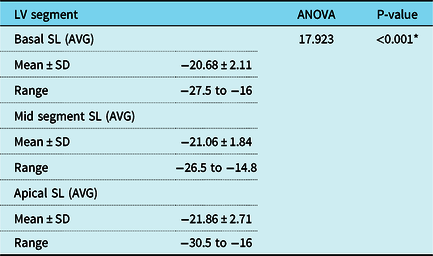
LV: left ventricle; SD: standard deviation; SL: strain longitudinal
Averaged basal longitudinal strain and averaged mid segment longitudinal strain 0.134
Averaged basal longitudinal strain and averaged apical longitudinal strain 0.001*
Averaged mid segment longitudinal strain and averaged apical longitudinal strain 0.001*
There were significant differences between the studied age groups as regard to the regional and global longitudinal systolic strain parameters (Table 4).
Table 4. Normal values of 2D –STE-derived regional and global systolic longitudinal strain categorised by age
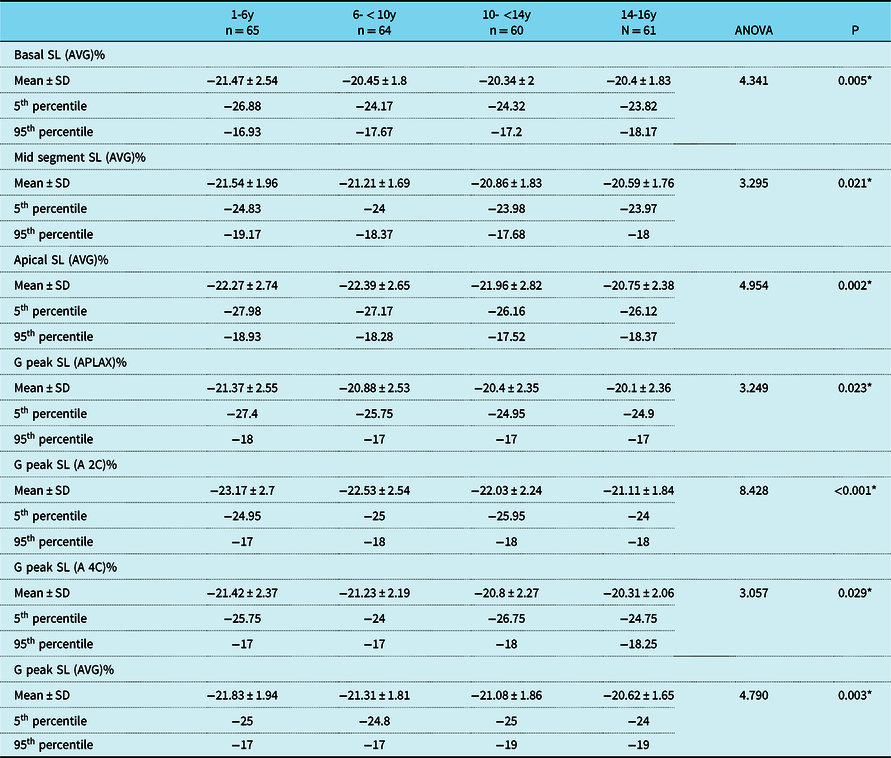
G peak SL (APLAX): global peak longitudinal strain at the apical long-axis view; G peak SL (A4C): global peak longitudinal strain at the four-chamber view; G peak SL (A2C): global peak longitudinal strain at the apical two-chamber view; G peak SL(Avg): averaged global peak longitudinal strain; SD: standard deviation; SL: strain longitudinal
The global peak longitudinal systolic strain at the apical long-axis view, apical four-chamber view, and apical two-chamber view, the averaged global peak longitudinal systolic strain, the averaged basal longitudinal strain, the averaged mid segment longitudinal strain, and averaged apical longitudinal strain were −20.7 ± 2.46%, −20.85 ± 2.18%, −22.15 ± 2.48%, −21.14 ± 1.92%,−20.71 ± 1.92%,−21.01 ± 1.94%, and −21.64 ± 2.82%, respectively, in males. They were –20.71 ± 2.53%, −21.13 ± 2.37%, −22.34 ± 2.46%, −21.33 ± 1.78%, −20.64 ± 2.34%, −21.13 ± 1.7%, and −22.13 ± 2.55%, respectively, in females with no statistically significant differences between both sexes (p > 0.05). There were no statistical significant differences between both sexes in each age subgroup as regard to the regional and global longitudinal left ventricular systolic strain parameters (Table 5).
Table 5. Gender comparison in the studied groups
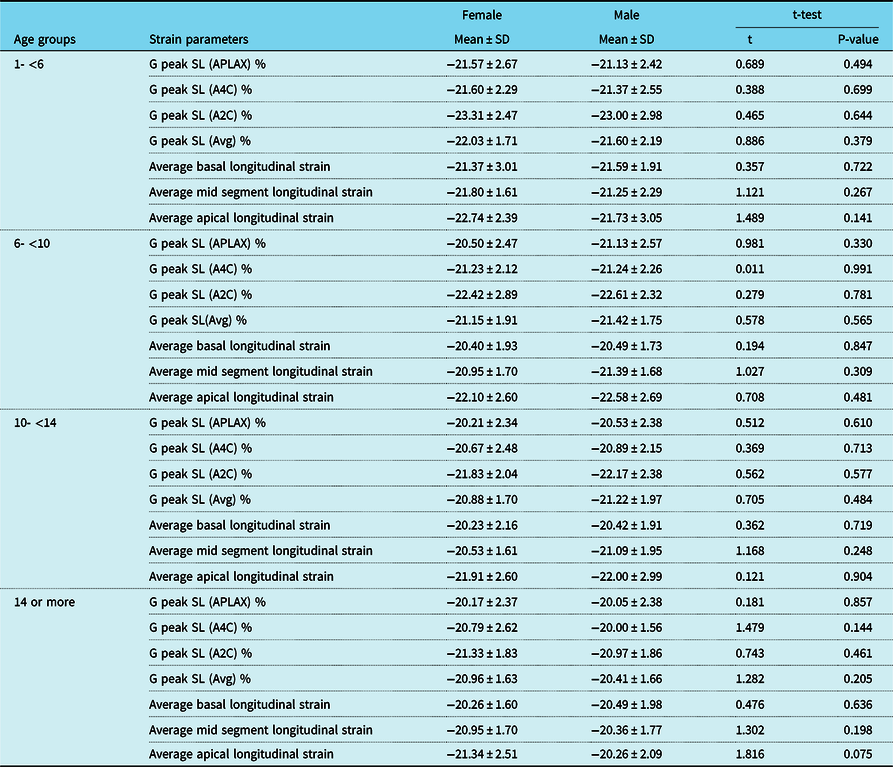
G peak SL (APLAX): global peak longitudinal strain at the apical long-axis view; G peak SL (A4C): global peak longitudinal strain at the four-chamber view; G peak SL (A2C): global peak longitudinal strain at the apical two-chamber view; G peak SL (Avg): averaged global peak longitudinal strain; SD: standard deviation
The averaged global peak longitudinal systolic strain correlated positively with age (r = 0.221, p < 0.001) and negatively with the fractional shortening (r = −0.168, p = 0.008). No correlations were found between the averaged global peak longitudinal systolic strain and the left ventricular internal diameter in diastole (r = −0.037, p = 0.559) or body mass index (r = 0107, p = 0.092) or body surface area (−0.034, p = 0589) or heart rate (r = 0.102, p = 0.109) or systolic and diastolic blood pressure (r = −0.054, p = 0.398) and (r = 0.053, p = 0.354), respectively.
Intra- and inter-observer reliability for the speckle tracking echocardiography-derived longitudinal strain parameters is shown in Table 6.
Table 6. Intra-observer and inter-observer variability
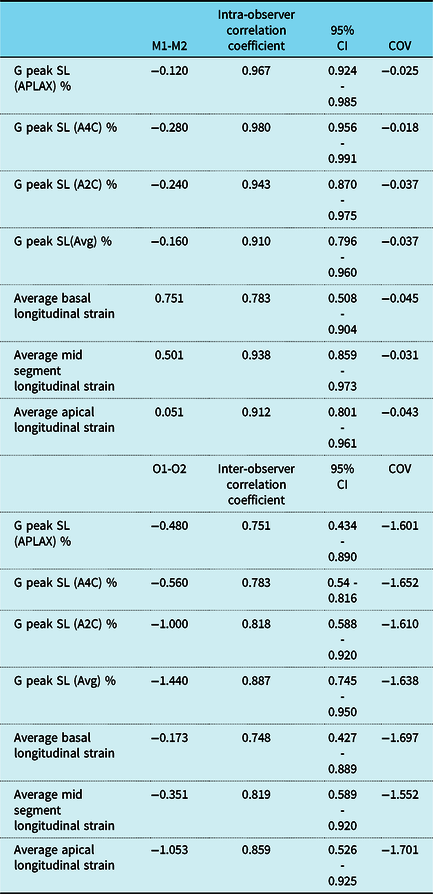
CI: confidence interval; COV: coefficient of variance; G peak SL (APLAX): global peak longitudinal strain at the apical long-axis view; G peak SL (A4C): global peak longitudinal strain at the four-chamber view; G peak SL (A2C): global peak longitudinal strain at the apical two-chamber view; G peak SL (Avg): averaged global peak longitudinal strain; M1: first measurement; M2: second measurement; O1: first observer; O2: second observer; SD: standard deviation
Discussion
The accuracy of evaluation of both global and regional systolic left ventricular function has improved with the advances of novel technologies as each new echocardiographic modality has overcome the limitations of the preceding one resulting in improved accuracy and better reproducibility. Reference Picard, Popp and Weyman1 Speckle tracking echocardiography is a relatively novel modality in the field of clinical and investigational echocardiography. It can easily derive the strain measurements from two-dimensional images without the limitations of tissue Doppler imaging, which renders it an easy appealing tool for echocardiographic assessment. Not only can speckle tracking echocardiography assesses the ventricular function, but it is also able to detect subclinical impairment. This opens the field for a wide variety of new clinical applications including different types of cardiomyopathies, valvular heart disease, and systemic illnesses with cardiac complications. It can also help in the assessment for dyssynchrony and diastolic function, in addition to quantification of right ventricular function. Reference Blessberger and Binder10 Moreover, assessment of the regional longitudinal strain is useful for accurate evaluation of segmental wall motion abnormalities, Reference Amzulescu, Langet and Saloux19,Reference Cimino, Canali and Petronilli20 which provides additional data in a wide range of cardiac pathologies such as cardiac amyloidosis, Reference Phelan, Collier and Thavendiranathan21 chronic ischaemic cardiomyopathy, Reference Bertini, Ng and Antoni22 and myocardial infarction. Reference Voigt, Arnold and Karlsson23
Recently, assessment of the systolic strain using two-dimensional speckle tracking echocardiography had been evaluated in different cardiovascular diseases including myocardial infarction, hypertrophic cardiomyopathy, metabolic diseases (amyloidosis, Fabry disease), Duchenne muscular dystrophy, cardiotoxcicity, valvular heart disease, and even in Atheletic hearts. Reference Zito, Longobardo and Citro24 Currently, applications of speckle tracking echocardiography are increasingly being studied in the research work for the evaluation of myocardial strain in the field of paediatric cardiology, Reference Forsey, Friedberg and Mertens25 and major endeavours are being performed on a large scale to test for the validity of this relatively new methodology and to relate the obtained two-dimensional speckle tracking echocardiographic measurements to hard end points. Reference Blessberger and Binder10
Up to the best of our knowledge, very few studies were concerned to set up reference values for the left ventricular strain in children using two-dimensional speckle tracking echocardiography. Reference Dallaire, Slorach and Bradley26–Reference Koopman, Rebel and Gnanam28 The values of the strain measurements are affected by the type of the software and the ultrasound machine used. Reference Koopman, Slorach and Hui29 Though the assessment of the global longitudinal strain revealed excellent reproducibility, a significant bias between different vendors was reported. Reference Plana, Galderisi and Barac30 Previous studies included a Japanese multi-centric study that included both adults and children and used different ultrasound vendors, but unfortunately data on the encountered children were incomplete, Reference Takigiku, Takeuchi and Izumi31 an Italian study in children using the Philips E33 ultrasound machine and QLAB 9 software which reported normal values for the global and regional circumferential left ventricular strain as well as the global longitudinal left ventricular strain but was lacking the regional longitudinal strain analysis. Reference Cantinotti, Scalese and Giordano32 A recent study done in Netherlands had assessed the global and regional longitudinal left ventricular strain as well as the circumferential strain in healthy children, but it also used the Philips platform. Reference Koopman, Rebel and Gnanam28 In this study, we provide normal reference values for the global and regional longitudinal left ventricular systolic strain in terms of centiles for healthy Egyptian children, who belong to the North African ethnicity, using the GE healthcare system through an automated functional imaging software integrated into the ultrasound machine.
In this study, the mean values of the global peak longitudinal systolic strain at the apical long-axis view, apical four-chamber view, and apical two-chamber view and the averaged global peak longitudinal systolic strain were −20.592 ± 2.437%,−20.952 ± 2.254%, −22.232 ± 2.468%, and −21.224 ± 1.862%, respectively. Takigiku et al Reference Takigiku, Takeuchi and Izumi31 detected a mean global longitudinal strain of −21.3 ± 2.1 using the GE Healthcare ultrasound machine (Vivid 7 or Vivid E9), −18.9 ± 2.5 using the Philips Medical System, and −19.9 ± 2.4% using Toshiba Medical System, but they included a more wider range of age (0–88 years). Using a Philips platform, Koopman et al Reference Koopman, Rebel and Gnanam28 detected mean values of global peak longitudinal systolic strain at the apical long-axis view, apical four-chamber view, and apical two-chamber view and the averaged global peak longitudinal systolic strain of −20.8 ± 2.8, −20.6 ± 2.6, −20.9 ± 2.7, and −21.1 ± 2.2, respectively, in healthy children between the age of 1 and 18 years. Our results were comparable to the published standard range from the meta-analysis done by Levy et al. Reference Levy, Machefsky and Sanchez33 This matches with the European Association of Cardiovascular Imaging/American Society of Echocardiography Industry Task Force that demonstrated acceptable differences among different vendors in relation to the global systolic longitudinal strain measurements. Reference Mirea, Pagourelias and Duchenne34
Though the clinical use of the global longitudinal left ventricular strain is expanding, the evaluation of the regional longitudinal strain has not been widely introduced into the clinical practice Reference Delgado and Marsan35 due to the higher variability of the obtained measurements when compared to the global strain systolic strain values. Reference Amzulescu, Langet and Saloux19 To limit this variability, the European Association of Cardiovascular Imaging/American Society of Echocardiography Industry Task Force reported that the segmental longitudinal strain should be assessed and compared with data obtained using the same ultrasound machine and the same vendor-specific software package. Reference Mirea, Pagourelias and Duchenne34 This study provides reference values for both global and regional longitudinal strain measurements using automated functional imaging and GE health care ultrasound platform that allows for more accurate interpretation of the strain values when using the same vendor and software package.
The human myocardium reveals growth- and age-related striking changes in its mechanics and trophic capacity, which is manifested by increase in the afterload and decrease in the myocardial contractility and reduction in the systolic function as the age increases. These developmental changes are more predominant during the first 4 years of life that become less prominent thereafter. Reference Colan, Parness and Spevak36 In this study, there were statistically significant differences between the studied age groups as regard to both global and regional longitudinal systolic strain; moreover; the averaged global peak systolic longitudinal strain correlated positively with age. This means that as the age increases, the averaged global peak systolic longitudinal strain increases (becomes less negative) and vice versa. This is in agreement with previous studies Reference Marcus, Mavinkurve-Groothuis and Barends14,Reference Dallaire, Slorach and Bradley26,Reference Koopman, Rebel and Gnanam28 and emphasises the practical need for age-specific reference ranges for more accurate interpretation of the two-dimensional speckle tracking echocardiographic-derived strain measurements. Though the small maturational changes in the global longitudinal strain based on age in this study may be statistically significant, but probably clinically irrelevant as most of the mean values varied between −20.62 and −21.83. However, having a standardised mean and range for all ages will improve the applicability of strain imaging when translated to clinical scenarios.
In this study, we did not find any correlation between the averaged global peak systolic longitudinal strain and any of the anthropometric or vital parameters. This indicates that no specific haemodynamic or anthropometric variable can explain the differences in the longitudinal strain values encountered between each of the studied age groups. This matches with Marcus et al Reference Marcus, Mavinkurve-Groothuis and Barends14 who suggested that it is the individualistic alterations in the morphometric variable of each subject, due its growth and developmental cardiac changes, that contribute to the overall differences in the systolic strain values observed among the different age groups.
In this study, there were no gender differences regarding both global and regional systolic strain parameters. This is in agreement with previous studies. Reference Marcus, Mavinkurve-Groothuis and Barends14,Reference Koopman, Rebel and Gnanam28
The non-uniformity of the left ventricular myocardial strain had been documented in multiple previous studies and was attributed to the irregular shape of the left ventricle in addition to the complexity of its architecture, regional variations in its morphology as regard to the wall thickness, and the radii of curvature. Reference Freeman, Le Winter and Engler37–Reference Buchalter, Rademakers and Weiss41 The myocardial deformation of the different left ventricular segments varies at the transmural level, around the left ventricular circumference and from base to apex. Reference Bu¨chi, Hess and Murakami42 Using myocardial magnetic resonance tagging, Bogaert and Rademakers Reference Bogaert and Rademakers43 found that the left ventricle showed marked variations in its wall thickness as well as in the longitudinal and circumferential radii of curvature in different segmental regions (the wall thickness and the circumferential radii of curvature decrease from base to apex, on the contrary, the longitudinal radii of curvature increase from base to apex). They also found that in the systolic phase, the wall stress becomes attenuated towards the apical segment due its small circumferential radius of curvature resulting in increase in its longitudinal strain when compared to the basal segment in addition to different myocardial architecture at the base with more cross-fibre shortening compared to the base. Moreover, they reported that this variation in the regional architecture resulted in obvious functional non-uniformity as the regional contribution of the left ventricular myocardium to the pumping of blood was highly variable (the ejection fraction increased from base to apex, the posterior wall of the left ventricle showed a comparatively higher ejection fraction, whereas the anterior part of the left ventricle showed the lowest ejection fraction). In this study, the mean values of the systolic longitudinal strain were significantly lower in the basal segments and become significantly more negative from base to apex. This is in agreement with previous studies Reference Marwick, Leano and Brown13,Reference Marcus, Mavinkurve-Groothuis and Barends14,Reference Levy, Machefsky and Sanchez33,Reference Kocabay, Muraru and Peluso44,Reference Menting, McGhie and Koopman45 that reported a base to apex gradient with the highest longitudinal strain values detected at the apical segment and the lowest at the basal segment. On the other side, Jashari et al Reference Jashari, Rydberg and Ibrahimi46 detected no significant base to apex differences in their total studied population though the increasing base to apex longitudinal strain values were observed in two of his studied age groups, whereas Koopman et al Reference Koopman, Rebel and Gnanam28 found that the mid-ventricular segments had the highest longitudinal strain values.
Though the assessment of the left ventricular dimensions and functions measured by m-mode echocardiography was beyond the scope of this study, we reported statistically significant differences in the left ventricular, left atrial, and aortic dimensions between different age groups. On the contrary, no significant differences were found as regard to the fractional shortening. This had been proved earlier by Gutgesell et al Reference Gutgesell, Paquet and Duff47 who found that the fractional shortening remains constant in healthy hearts from birth onwards. In this study, the averaged global peak systolic longitudinal strain; expectedly, correlated negatively with the fractional shortening, this means the higher the value of the fractional shortening, the lower the value of the averaged global peak systolic longitudinal strain (becomes more negative). In this study, no correlations were found between the averaged global peak systolic longitudinal strain and the left ventricular internal diameter in diastole. Similarly, Marcus et al Reference Marcus, Mavinkurve-Groothuis and Barends14 did not find a strong relation between the cardiac size and the peak systolic strain measurements.
Automated functional imaging is an easy, less time consuming, feasible, effective, and accurate modality for the assessment of the two-dimensional speckle tracking echocardiographic-derived strain values that shows a very satisfactory reproducibility independent of the operator’s experience. Reference Belghitia, Brettea and Lafittea48 In this study, we detected good intra- and inter-observer reliability scores. High intra- and inter-observer reliability scores for almost all of the left ventricular strain parameters using two-dimensional speckle tracking echocardiography were also documented in previous studies. Reference Mavinkurve-Groothuis, Weijers and Groot-Loonen49,Reference Abou, Leung and Khidir50 The higher reliability in our study could be explained by the automated functional imaging modality we used in our assessment.
Study limitations
The data included in our study are representative of children who belong only to the North African ethnicity; hence; data from other ethnic backgrounds are missing. However, this will eliminate the bias of using reference values of different racial compositions when assessing children belonging to the North African ethnicity. Moreover; this work will allow for future comparative studies between children of different races and ethnicities.
As maturational and developmental changes in the myocardial contractility were proved to occur in the newborn babies at birth and to continue throughout infancy, Reference Rowland and Gutgesell51 we did not include infants below the age of 1 year as we believe they have different haemodynamics that we shall consider in our future research. Also, we did not assess for neither the circumferential nor the radial LV strain due to non-availability of the software in our ultrasound machine.
Although we were aware that the Z-scoring approach is the widely used scoring method in the field of the paediatric echocardiography, previous studies had documented that the effect of the body size on the deformation parameters is much weaker when compared to its effect on the size of the cardiac structures as well as the values of the Doppler measurements. Reference Lai, Geva and Shirali17,Reference Dallaire, Slorach and Bradley26,Reference Cantinotti, Scalese and Franchi52 For this reason, we chose not to apply it in our study as we also did not find a correlation between the strain parameters and the anthropometric measurements of the studied individuals.
Conclusion
We believe that this study adds to the growing literature that used the GE healthcare vendors to set normal values for both global and regional longitudinal left ventricular systolic strain in Egyptian children who belong to the North African ethnicity, using automated functional imaging, which is important for the evaluation of ventricular function in relevant clinical scenarios.
Acknowledgements
We thank the children and their caregivers for their participation in the study. We also thank the second observer who performed the echocardiographic examinations for the twenty-five children.
Financial support
This research received no specific grant from any funding agency, commercial, or not-for-profit sectors.
Conflicts of interest
None.
Ethical standards
This study had been carried out in accordance with the Code of Ethics of the World Medical Association (Declaration of Helsinki) for experiments in humans and was approved by the Ethics Committee of the Pediatric department, Ain Shams University.