There have been many discoveries of Roman lead (Pb) palaeo-contamination in urban sedimentary archives throughout the Mediterranean basin.Footnote 1 Indeed, Pb resistance to corrosion, its malleability, and the low melting temperature made it the raw material of choice during antiquity for, inter alia, piping, architecture, glassware, crockery, and paint.Footnote 2 The use of plumbum was so prevalent during Roman antiquity that Pb-Ag ore mining was the main source of Pb contamination detected in Greenland ice cores.Footnote 3 The Roman atmospheric Pb peak has therefore been considered a possible chronostratigraphic marker.Footnote 4 Moreover, Pb has four stable isotopes of masses 204, 206, 207, and 208, the last three of which are the end products of the Uranium (238U and 235U) and Thorium (232Th) radioactive decay chain. The relative quantity of 238U, 235U, and 232Th in Pb ores varies according to the age of the source rock and its initial U and Th concentrations.Footnote 5 As such, isotopic imprints coupled with concentration analyses provide a marker of anthropogenic activities, as well as of the geographical origin of the ores used in the manufacture of urban artifacts, when compared to the Pb isotopic signature database of known deposits. In that respect, anthropogenic lead in sediments constitutes a proxy for the expansion and contraction of ancient urban development.Footnote 6 Here, we used this geochemical indicator in sediments of the Vistre de la Fontaine palaeochannel to document the environmental footprint of Nemausus (Nîmes, southeastern France) during antiquity.
Archaeological assessment carried out as part of a project to restore the meandering trajectory of the Vistre de la Fontaine stream on the outskirts of rural Nîmes led to the excavation of a palaeochannel in the watercourse and of a bridge dating back to the Early Roman Empire (Fig. 1). The excavation area opened a 2,500-year time window on the evolution of a section of the palaeo-Vistre de la Fontaine. Archaeology, stratigraphy, sedimentology, and malacology of this alluvial sequence together show the migration of the minor bed and its convex bank over an approximate length of 30 m.Footnote 7 The accretion of this bank therefore featured a sequence of sedimentary archives that was investigated following a multiproxy approach. Granulometry, sedimentology, and analyses of major and trace elements and Pb isotopic signatures made it possible to test for the presence and origin of metallic palaeo-pollutants recorded in the alluvial sediments during the historical period, in connection with the development of the city located approximately 3 km upstream from the site. Additional data include anthracological, archaeozoological, and carpological remains found within the Roman-period minor bed of the river. Originating from a sacred karstic spring that became a central node in the development of the Nîmes agglomeration from the end of the 6th c. BCE, the Vistre de la Fontaine River displays today physicochemical indications of domestic and agricultural contaminants (sewage waters and high nitrate levels, respectively).Footnote 8 This study explores for the first time the deep history of anthropogenic contamination of this urban river.

Fig. 1. Aerial view looking northwards from the Vistre de la Fontaine 2-2 site under excavation, in the Vistrenque plain, at the foot of the Nîmes limestone hills, northwest of the Rhône delta. The inset map top-left shows the geographical context of the Vistre de la Fontaine, a tributary of karstic origin of the Vistre coastal river, itself a tributary of the Rhône-Sète canal. (Photo © Globdrone.)
Methods
Chronostratigraphy
The Vistre de la Fontaine is a karstic river flowing from a perennial spring which constituted the heart of Nîmes from its foundation in the 6th c. BCE. The Vistre de la Fontaine 2-2 site, located ca. 3 km downstream from the city, allowed the excavation of a Roman bridge that used to cross the river and opened a window onto the evolution of its palaeochannel over the last 2,500 years. A stratigraphic section of about 40 m in length with a deep dig and three auger cores intersected the accreting palaeo-bank over a length of about 30 m (see stratigraphic transect in Fig. 2), producing a temporal sedimentary series that was horizontally structured. This section underwent a detailed stratigraphic field survey and an orthorectified and georeferenced photogrammetric survey. Thirty-three samples of raw sediment were taken horizontally along the convex bank's lateral accretion. The granulometry of the deposits was approximated using wet sieving and fraction quantification of gravel (> 2mm), coarse sand (> 0.5 mm), fine sand (> 0.063 mm), and silt and clay (< 0.063 mm) (Table 1). The composition of sand and gravel fractions were observed using binocular loupes. The chronology of this sequence was established on the basis of stratigraphic relationships, archaeological structures in situ, and archaeological artifacts in secondary positions, as well as by three radiocarbon dates (Table 2).
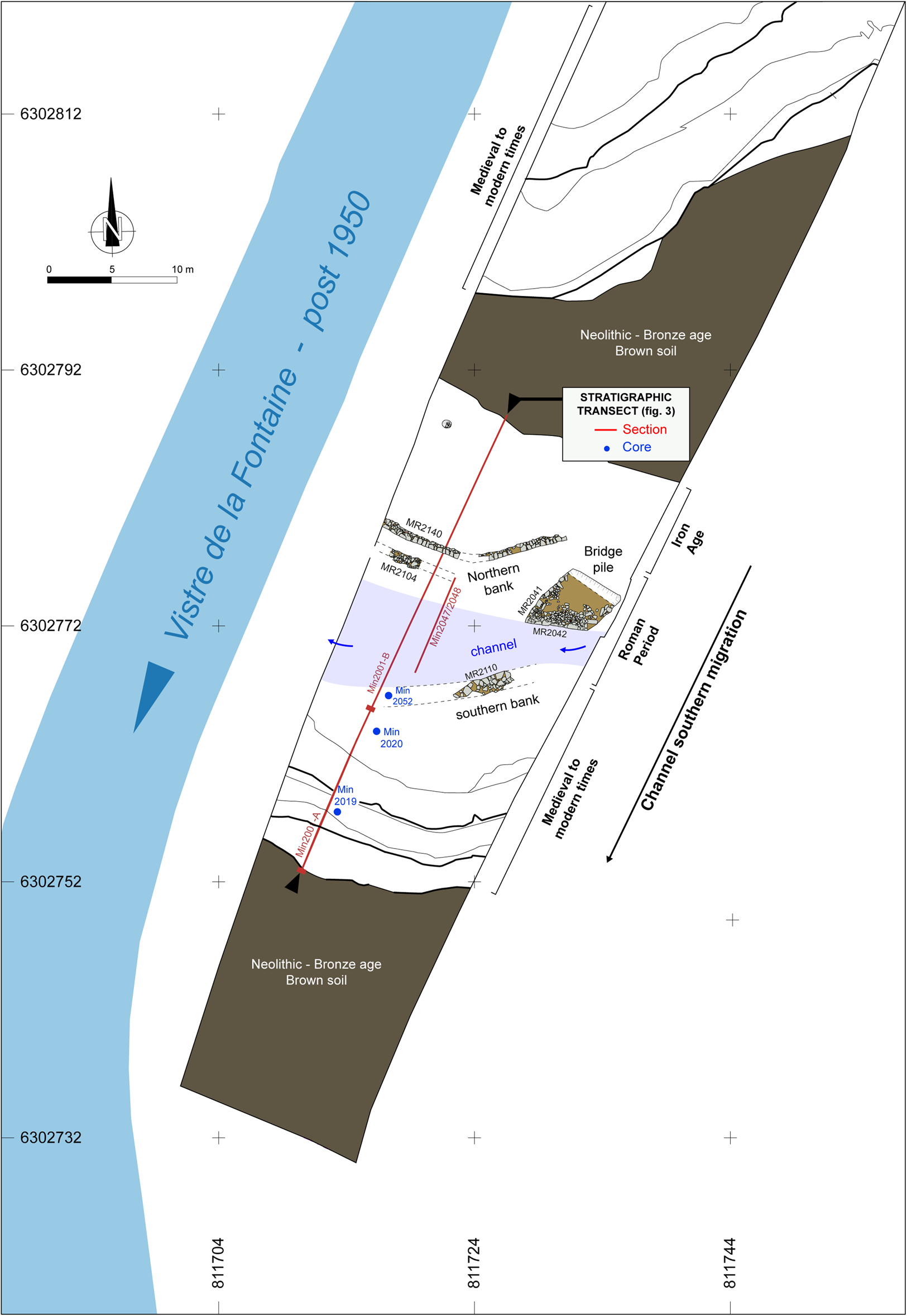
Fig. 2. General Holocene phasing of the stripped surface, map of the Early Roman Empire remains, and location of the stratigraphic sections. Lambert 93 coordinate system. (Map by C. Flaux.)
Table 1. Granulometric data and XRF data applied along the accretionary profile of the convex bank of the Vistre de la Fontaine palaeochannel (samples 1–33; Fig. 3). Measurements were made on two certified sediment standards (NIST Standard 2710a and NIST Standard 2711a) at the beginning and end of the analysis, enabling the error bar to be calculated for each element (**).

Table 2. Samples selected for radiocarbon dating (calibration carried out according to Reimer et al. Reference Reimer, Austin, Bard, Bayliss, Blackwell, Bronk-Ramsey, Butzin, Cheng, Edwards, Friedrich, Grootes, Guilderson, Hajdas, Heaton, Hogg, Hughen, Kromer, Manning, Muscheler, Palmer, Pearson, Van der Plicht, Reimer, Richards, Scott, Southon, Turney, Wacker, Adolphi, Büntgen, Capano, Fahrni, Fogtmann-Schulz, Friedrich, Köhler, Kudsk, Miyake, Olsen, Reinig, Sakamoto, Sookdeo and Talamo2020).

Bio-archaeology
Fluvial deposits that filled the Roman palaeochannel as a result of stable hydromorphic conditions provided a sequence of carpological, anthracological, and zoological data from the Roman period. The samples were prepared and studied at the Mosaïques Archéologie laboratory. Details of the bioarchaeological methods and studies employed on the Vistre de la Fontaine 2-2 site can be found in the excavation report.Footnote 9
Nine samples of approximately 10 L were selected for anthracology, from which 360 charcoals were extracted after wet sieving of bulk sediments through mesh of 4, 1, and 0.5 mm. Four samples of approximately 10 L were selected for carpology, from which ca. 8,000 remains were extracted after wet sieving of bulk sediments through mesh of 4, 1, and 0.35 mm. One thousand and forty-nine archaeozoological fragments were collected during the excavation and after wet sieving of bulk sediment sampled from the fluvial context (both minor bed and bank) of the Roman era.
Geochemistry
XRF geochemical analysis was performed upon the 33 samples taken along the convex bank's lateral accretion. The raw sediment samples were dried in a heating cabinet at 35°C for ten days, then ground in an agate mortar and compacted into a dish covered by a 4μm-thick polypropylene film used specifically for XRF analysis. Measurements were taken using an InnovX Delta Premium spectrometer equipped with a silicon drift detector (SDD) and a 4W X-ray tube with a rhodium target operating between 10 and 40 kV for a maximum amperage of 200μA. Soil mode was selected with a long exposure time for better accuracy.Footnote 10 The raw data from the analysis are presented in Table 1.
Stable lead isotope ratios were measured using a Neptune+ MC-ICPMS (Multi-Collector Inductively Coupled Plasma Mass Spectrometer) at CEREGE (CNRS, France), after microwave-associated digestion of sediments and Pb extraction and purification on AG1X8 resins.Footnote 11 The isotope ratios provided in Table 3 were normalized by multiple analyses of the NBS981 standard.Footnote 12 Analytical uncertainties (standard deviation) for the measured Pb isotope ratios presented in figures and tables was lower than 0.012%.
Table 3. Lead isotope ratios. Preparation and analysis of samples took place at CEREGE (CNRS, UMR7730), on a Neptune+ MC-ICPMS (Multi-Collector Inductively Coupled Plasma Mass Spectrometer). The reproducibility of these measurements is associated with an error bar lower than 0.012% for all reported lead isotope ratios.

Sedimentary archives
General stratigraphy of the Vistrenque
The archaeological site is located on the lower Vistre de la Fontaine River, a tributary of the Vistre coastal river. The alluvial plain of the Vistrenque forms a depression 5 to 7 km wide, extending in a northeast–southwest direction and separating the Garrigues area in the north from the Costières de Nîmes in the south (Fig. 1). The hills and plateaus of the Garrigues region are formed of marly limestone from the Lower Cretaceous, and their piedmont plain is commensurate with a detrital glacis formed during the Pleistocene and Holocene.Footnote 13 The Costières to the south comprise series of alluvial terraces formed by the Rhône during the Lower and Middle Pleistocene. These terraces also form the bedrock of the Vistrenque, which would have subsided through synsedimentary tectonic processes.Footnote 14
The main lines of the surface formations of the Vistre plain are now well understood, thanks to the numerous geoarchaeological and palaeoenvironmental studies carried out in this area.Footnote 15 The ante-Holocene substratum of the sedimentary sequence consists of the detrital formation of the Costières, the roof of which lies around 3.5 m below the surface, covered by a “loessic complex,” deposited over a thickness of 2 to 2.5 m and dated to the recent Pleistocene, with a roof that is capped by a palaeosoil formed during the Tardiglacial period. The Holocene is represented by a pedosedimentary sequence of fine alluvial deposits, 0.8 to 1.25 m thick, characterized by a shelly brown palaeosoil, which, in the Vistrenque, is generally defined by occupations from the Neolithic and the Bronze Age.
The construction of the meander
The palaeochannel of the Vistre de la Fontaine was created in a silty alluvial plain. The stripped surface of the site, cleared to about 50 cm below the present soil, reveals this dichotomy in the form of a winding pathway nested in the shelly brown palaeosoil (Fig. 2). Stratigraphic transect 1 (Fig. 3) intersects this formation in a north-northeast–south-southwest direction, showing the truncation of the Neolithic brown, shelly palaeosoilFootnote 16 and the underlying loess complex in the form of a 39 m-long basin with a maximum depth of 2 m below the stripped surface. Overall, the basin contains two main sedimentary facies. At the bottom, deposits are mostly coarse, lenticular conglomerates of rolled and joined gravels interspersed with more sandy or even muddy lenticular bodies, which are consistent with the filling of an active minor bed. The upper part is much more homogeneous, composed of massive and monotonous yellowish-brown to greyish-brown sandy silts over a length of more than 30m, intersected by oxidized rhizoconcretions. The homogeneity of this facies is also evident in its texture. Granulometry is mainly fine, with 53 to 77% of silt and clay, 24 to 36% fine sands (Table 1). The sand fraction is invariably composed of abundant, poorly rolled carbonate rhizoconcretions (interpreted as detrital travertines), calcareous and quartz minerals, and malacofaunal elements (shells, shell fragments, and operculum in variable proportions). However, the apparent homogeneity of this layer is structured in multi-metric sedimentary units with oblique interfaces with a 15 to 20° southward inclination, marked by strandlines composed of charcoals or shells left by a high-water level (Fig. 3). This geometry evokes the slope of a fluvial channel bank, and the horizontal succession of deposits provides evidence for the southward accretion of this bank, burying the deposits of the Vistre de la Fontaine palaeochannel, jointly migrating towards the south through the erosion of the concave bank. This mechanism reflects the construction of a meander. The malacofauna studied along this sequence shows mixed assemblages composed of aquatic and terrestrial fauna in variable proportions, consistent with the transitional space constituted by these alluvial palaeobanks.Footnote 17 The geoarchaeological study of the site, via analysis of anthracological, carpological, palynological, malacological, and archaeozoological alluvial assemblages, has revealed that the Vistrenque formed a diversified mosaic landscape, an open and cultivated alluvial plain where wooded areas nevertheless remained.Footnote 18
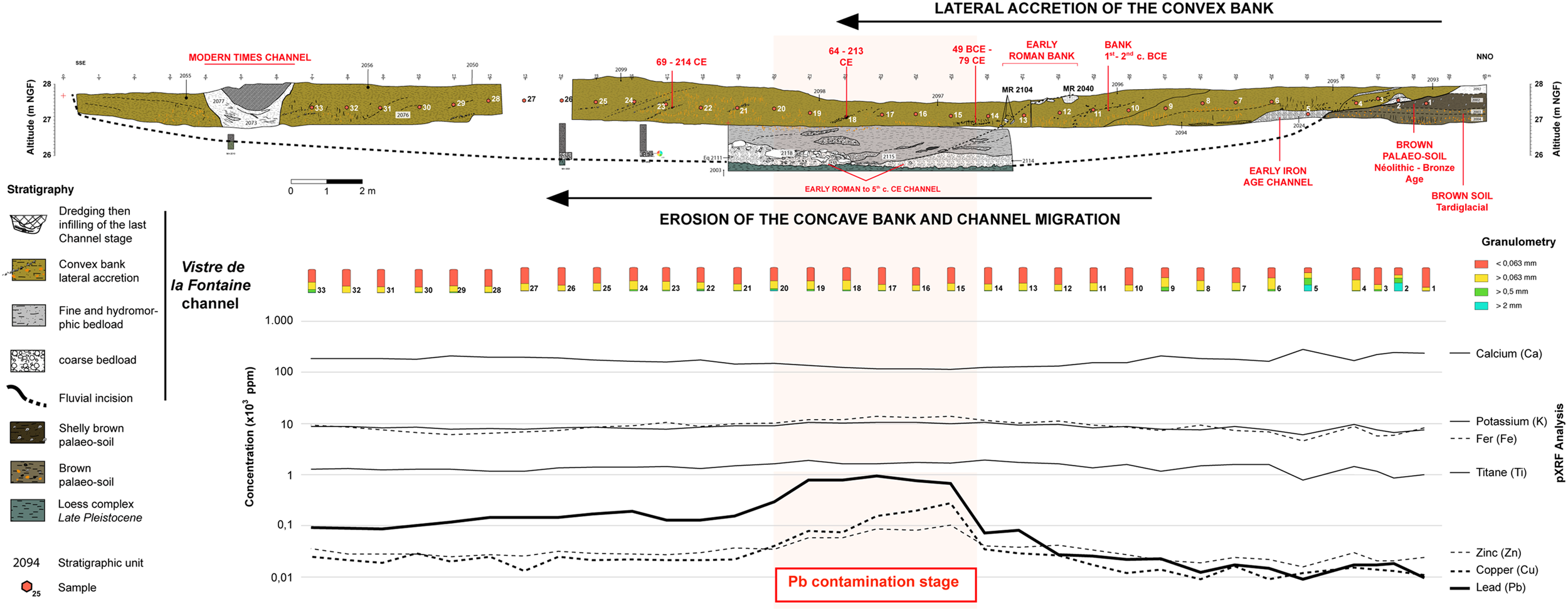
Fig. 3. South-southeast–north-northwest stratigraphic transect intersecting the palaeochannel of the Vistre de la Fontaine and the southward migration of its convex bank. Location of discrete samples and chronological, granulometric, and geochemical data (XRF) are also shown. (C. Flaux.)
Chronology of the meander
The stratigraphy of this homogeneous sedimentary series, structured in multi-metric units with oblique interfaces and a southward inclination of 15 to 20°, and marked by strandlines composed of charcoals or shells left by a high-water level, implies the chronological succession of the deposits of this bank in a southward accretion, burying the laterally and jointly migrating channel deposits. It was possible to date three phases of deposition for these successive channels (Fig. 3). The first, on the north side, is coarsely lenticular and yielded a series of non-rolled ceramic fragments in a good state of preservation, favoring the hypothesis of a rubbish tip in the palaeochannel of the Vistre de la Fontaine. The assemblage yielded hand-thrown pottery, fragments of Etruscan amphorae, and Eastern Greek ceramics dated to the 6th c. BCE.Footnote 19 The second phase of channel deposits corresponds to the fluvial activity contemporary with the construction of the bridge and dated to the Early Roman Empire (1st–2nd c. CE).Footnote 20 The ceramic assemblage associated with the deposit of this ancient palaeochannel reveals a wider time period, between the 2nd c. BCE and the 5th c. CE, although the overwhelming majority of the ceramics are dated to the Early Roman Empire. Finally, the third phase corresponds to the final sealing of the palaeochannel, traces of which are still visible on contemporary aerial images. The final phase of the filling is anthropic, incorporating fragments of contemporary ceramics and iron elements (nails, rods, barbed wire), providing a terminus post quem of the first half of the 20th c., consistent with the mechanical rectification of the channel carried out between 1945 and 1953.Footnote 21
The accretion of the convex bank of the Vistre de la Fontaine palaeochannel thus took place between the First Iron Age and the contemporary period. One of the oblique interfaces marking the slope of this palaeobank is highlighted in sections by walls MR2040 and, about 1 m below and 2 m farther south, MR2104 (Fig. 3). This construction of the bank of the ancient palaeochannel is associated with the addition of the bridge during the Early Roman Empire, consistent with a ceramic assemblage dated to the 2nd–1st c. BCE and discovered within the bank sediments underlying these two walls.Footnote 22 Finally, three radiocarbon dates were ascertained from organic material (Table 2) taken from oblique strandlines composed of charcoals left by a high-water level made after these two walls. From north to south, these three charcoal-bed dates are 49 BCE–79 CE, 64–213 CE, and 69–214 CE, respectively. These bank deposits bury the minor-bed deposits of the ancient palaeochannel, dated by the ceramic assemblages to between the 2nd c. BCE and the 5th c. CE, and are therefore stratigraphically subsequent. The radiocarbon dates appear to contradict the chronological model based on the ceramic material. However, it must be considered that in a fluvial environment, sedimentary stocks can be reformed when transiting along the longitudinal profile of the river. For example, during the accretion of the convex bank of the Vistre de la Fontaine, the sedimentary material is likely a result of an eroding concave bank upstream. This mechanism would explain why the radiocarbon ages of the two southernmost radiocarbon samples cover the same period (64–213 versus 69–214 CE; Fig. 4 and Table 2): they probably derived from the same sedimentary stock further upstream.
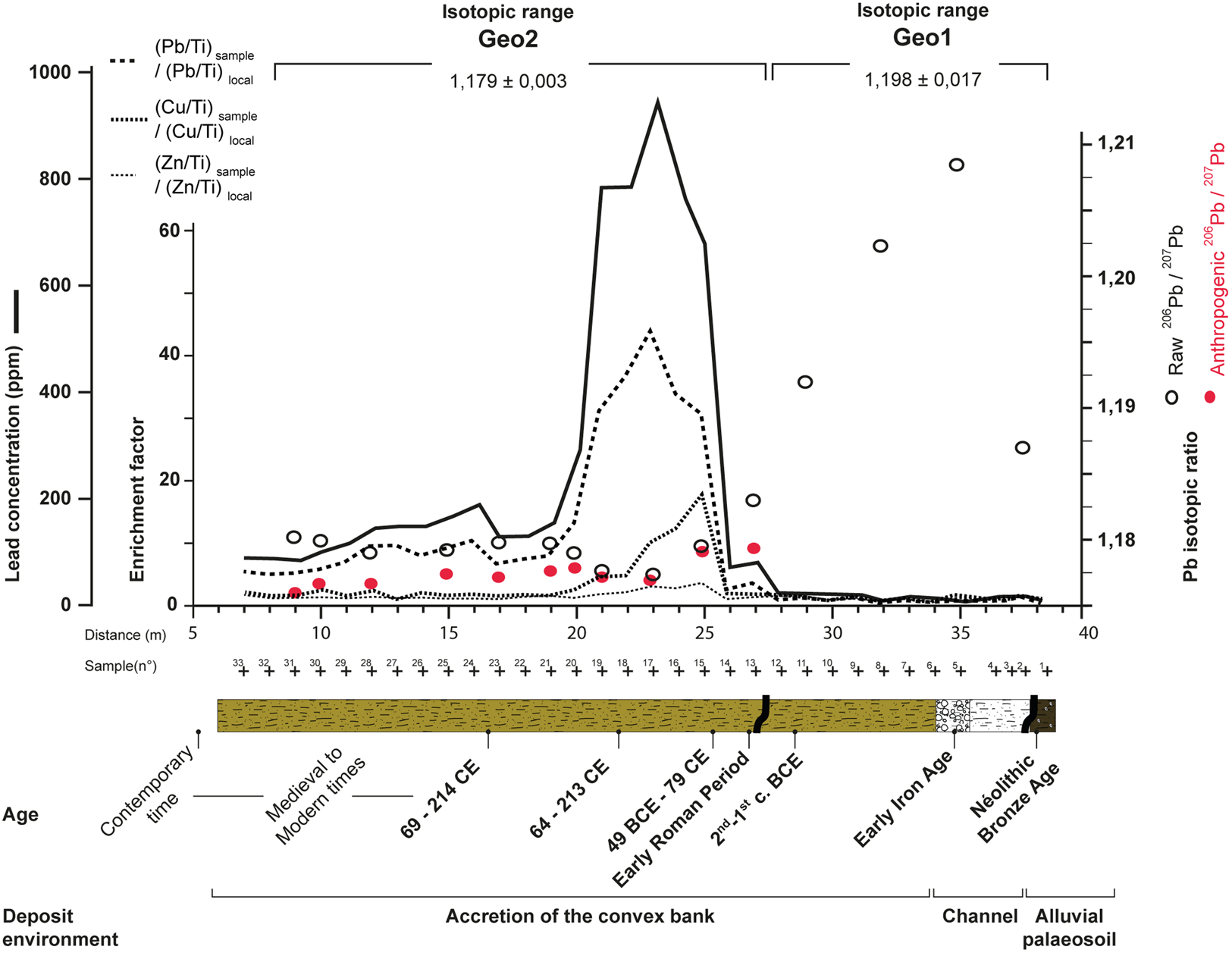
Fig. 4. Synthetic stratigraphic log of the site showing chronology, Pb concentration (ppm), Pb, Cu, and Zn enrichment factors (calculated according to Barbieri Reference Barbieri2016), and 206Pb/207Pb isotopic ratios, raw and in excess (Pb of anthropic origin). Lead isotope ratios are associated with an error bar lower than 0.012%. (C. Flaux.)
Waste deposition in the palaeochannel
The ancient palaeochannel and the way it developed allowed for the accumulation of a fluvial sedimentary stock at a depth of 2 to 3.5 m. This remained below the water table in apparently stable hydromorphic conditions and so enabled the good preservation of carpological and palynological material that complements the archaeozoological and anthracological assemblages.Footnote 23
The archaeozoological assemblage is dominated by the remains of livestock (cattle, pigs, sheep, and goats) as well as other domestic species (chickens, equines, and dogs). Traces of carcass cuttings, particularly the chopping of animal legs into pieces, are suggestive of butchery and consumption of meat. The assemblage also includes some bone remains that show no traces of human intervention, likely from wild species (lagomorphs, murids) and species whose domestic or wild status remains undetermined (cats, small canids, Columbidae). Most of the fragments have been rolled and the bone surfaces are disintegrating (desquamation), indicating their displacement by the watercourse before their final deposition. Traces of trimming indicate the intervention of detrivores on some bones. The archaeozoological assemblage is therefore not in a primary position and demonstrates diversified inputs via the Vistre de la Fontaine River.
The carpological assemblage is diversified, composed of cultivated taxa, crop weeds, woodland, forest, and forest borders. The anthropic contribution can be seen in the high proportion of fruit and seed remains that may have been of nutritional benefit (figs, grapes, cherries/wild cherries, olives, possibly pomegranates, melons/cucumbers, gourds, parsnip, and coriander seeds), as well as in the high level of fragmentation of some remains, indicating hulling or grinding. The discovery of some 40 rolled and highly eroded remains that resemble either shell (nuts?) or pit (olives, Prunus family?) fragments is suggestive of a contribution of allochthonous material, probably from the ancient city of Nîmes. Roman-period sites inside and outside the city walls yielded similar “economic” taxa to those found on the Vistre de la Fontaine 2-2 site, and these are common in urban and peri-urban settlements and in funerary environments.Footnote 24 The Vistre de la Fontaine exported some of its organic waste outside of the town. On the other hand, the discovery of pressed grape residues (highly fragmented pips, pedicels, skin, stalk, and aborted berries) indicates there was no displacement in the watercourse or local discharge either from the banks of the channel or directly from the bridge that overhangs the deposition area. In an urban or rural context, the Vistre de la Fontaine resembles a waste disposal area.
The anthracological and xylological samples collected in the Vistre de la Fontaine palaeochannel are also very diverse, including soaked fragments from timber, charcoal of fruit and ornamental taxa, and fuelwood. The high proportion of heather within the anthracological spectrum, which has been shown to be a fuel of choice of the ancient city,Footnote 25 indicates that the assemblage is, at least in part, representative of the range of waste that passed from the city of Nîmes into the riverbed. Charcoal and soaked-wood analysis shows that the zones used for gathering firewood or wood for the manufacture of shaped objects include all the wooded environments present around Nîmes: the mixed oak forest of the Garrigues and Costières, the alluvial forest of the plain, the riparian forest, the possible relict woodlands on nearby mountains, and also cultivated trees. This diversity reflects the wider environment of Nîmes rather than just the immediate riparian setting of the site being studied.
In antiquity, the demographic and urban expansion of Nemausus, as well as the development of cottage industries and commercial trade, led to a proliferation of waste and various kinds of pollution. Recent studies of ancient rubbish tips indicate a concern to preserve the cleanliness of public areas and efforts to relocate rubbish dumps to the periphery of the Augustan enclosure and to roadside locations.Footnote 26 Bioarchaeological remains found in the Roman channel of the Vistre de la Fontaine underline the fact that the river also transported urban waste outside the city of Nîmes, suggesting that the river acted as a sewer. Archaeological research in Nîmes has shown that the Vistre de la Fontaine was indeed largely integrated into extended and complex wastewater drainage networks.Footnote 27 The Arles harbor dump in the Rhône is representative in this respect, since it gathered thousands of waste items from the city's domestic, cottage-industry, and harbor activities.Footnote 28 In Nîmes, the urban river also carried invisible waste such as metals.
Chemical imprint
Lead contamination of the banks
The overall macroscopic homogeneity of the sedimentary matrix of the accretionary sequence of the Vistre de la Fontaine's convex palaeobank, as expressed by grain size data, is confirmed by the weak variation of the calcium, potassium, and iron content (Fig. 3 and Table 1) that dominate the elemental composition of the detrital load. This homogeneity contrasts with the variability of Pb content (Fig. 3). In the north–south direction of the lateral accretion of the palaeo-Vistre de la Fontaine's convex bank, the Pb profile shows an initially low content (10–20 ppm), followed by a sharp rise to nearly 1,000 ppm, and a fall to rather steady concentrations (100–200 ppm) that remain about ten times higher than the geochemical background recorded at the beginning of the sequence, that is, the Neolithic soil. Copper (Cu) and zinc (Zn) levels broadly follow a similar pattern (Fig. 3).
Metal enrichment factors (EFm with m = Pb, Cu, or Zn) were calculated to estimate the metal enrichment in relation to the local geochemical background:

The metal concentration is normalized to titanium (Ti), which is a conservative terrigenous element in soils,Footnote 30 that is, not prone to mobility, with a variability linked to that of the natural source from which the sediments are derived. The local geochemical background corresponds to the metal content measured within pre-anthropic sediments from samples 3, 4, and 6 to 11 (Fig. 3) because these sediments have a silicoclastic and silty matrix like contaminated sediments. An EFm >3 is considered enough to suggest excess accumulated metal that may be of anthropogenic origin.Footnote 31 EF values above 40 are considered extremely high.Footnote 32 These enrichment factors are shown in Figure 4, where a significant enrichment is evidenced during the Early Roman Empire (20–25m in the sequence) for Pb (EFPb max = 44), to a lesser extent for Cu (EFCu max = 18), and slightly above the materiality threshold for Zn (EFZn max = 3.5). EFPb indicates a massive and sudden source of lead input into the alluvial sedimentary system of the Vistre de la Fontaine. For comparison, an environmental lead contamination during the Roman Empire was also observed in the Étang de Thau by elemental and isotopic analyses of mussel shell.Footnote 33
Possible source of Pb contamination
Although ceramic or wooden pipes existed during antiquity, the use of Pb pipes was very common in Roman times,Footnote 34 this material being known for its elasticity and resistance to pressure.Footnote 35 In Nîmes, A. Pelet described in 1863 “an infinite number of lead pipes criss-crossing our city [Nîmes] which we discover every day buried under our soil.”Footnote 36 In particular, the sector of the Fontaine spring has yielded Pb pipes in abundance, as has the Castellum sector, the sewer and distributor of water from the Nîmes aqueduct. The geography of the lead pipe discoveries (40 in Alain Veyrac's catalogue),Footnote 37 the ten leakage pipes from the Castellum, each about 35 cm in diameter, and the distribution of the thermal baths in the city provide, inter alia, evidence that almost the entire extent of the Roman city was supplied with water by two distinct networks independently of each other (the aqueduct of Nîmes and the conveyance of water from the Fontaine source), composed mainly of Pb pipes. The ancient main sewers were drained away in the main water flow, downstream of the Vistre de la Fontaine.Footnote 38 Therefore, the discharge of bioarchaeological remains (partly resulting from domestic and cottage-industry discharges from Nîmes) and Pb contaminants downstream of the Roman city is no wonder. The Pb anomaly starts abruptly after the construction of the bridge during the Early Roman Empire. The archaeological data from Nîmes show that the upkeep of the Roman and public water management system declined during the 2nd c. CE and ceased during the 4th c. CE.Footnote 39 Our hypothesis is that the Pb peak identified in the sediments of the convex bank of a meander of the Vistre de la Fontaine evidences the installation, use, and then abandonment of Nîmes's lead pipe network during the Early Roman Empire. Comparing the isotopic signature of these remains with that of the contamination generated in the river will allow the validity of this hypothesis to be examined.
Origin of Pb ore
Alain Veyrac assumed that Pb pipe manufacturing workshops must have existed in Nîmes, at least to satisfy local demand.Footnote 40 This hypothesis was confirmed by the discovery of two Pb pipes in the vicinity of Nîmes, at Balaruc-les-Bains (Hérault), each bearing an inscription referring to the Nîmes colony.Footnote 41 Likewise, in the Ambrussum way station in Villetelle (Hérault), a stamp on a Pb pipe showed similarities to the onomastics of the city of Nîmes, suggesting a plumbing company based in Nîmes may have been involved.Footnote 42 Using stable Pb isotopes, we may infer the geographic origin of the Pb ores that contaminated the palaeochannel during the Roman Empire.
The excess Pb measured in the sediments of the banks of the Vistre de la Fontaine provides evidence of an anthropogenic contribution superimposed on the local geochemical background. Both may be identified by their isotopic signatures. The horizontal profile of the 206Pb/207Pb ratio of the alluvial sequence is characterized by two main isotopic fields, Geo1 and Geo2 (Fig. 4). The Geo1 field, associated with relatively low Pb content (9–25 ppm), has a variable and more radiogenic isotopic signature (206Pb/207Pb = 1.198 ± 0.017) as compared to the Geo2 field, which is characterized by higher Pb content (100–1,000 ppm) and a more homogeneous and less radiogenic isotopic signature (206Pb/207Pb = 1.179 ± 0.003). The most radiogenic values are consistent with those of uncontaminated sediments deposited in the western Mediterranean basinFootnote 43 ([Pb] = 13–20 ppm; 206Pb/207Pb = 1.195–1.233); in the ancient harbor of FréjusFootnote 44 ([Pb] = 14–20 ppm; 206Pb/207Pb = 1.202 ± 0.002); and in the uncontaminated sediments of the Étang de Thau, southwest of MontpellierFootnote 45 (206Pb/207Pb = 1.200 ± 0.003). Within the Geo1 field, samples 2 and 11 show a slightly less radiogenic isotope ratio (206Pb/207Pb around 1.19), which could indicate differentiated (anthropogenic?) Pb contributions prior to the Roman-period contamination. We believe that the Geo1 isotopic field constitutes, if not the natural geochemical background given an unstable isotopic signature from possible low amplitude proto-contamination sources, at least the local geochemical background before the advent of the Roman Empire. Given the relative uniformity of the measured 206Pb/207Pb ratios from each Geo field, the mean isotopic signature of the excess Pb in the Roman palaeo-bank can be assessed from the 206Pb/207Pb (y) vs. 1/Pb (x) relationship (Fig. 5C). The latter shows a significant strong correlation (r2 = 0.97, p < 0.01) with y = 1.178 + 0.3x. Therefore, one can calculate that the mean isotopic imprint of Pb in excess into the fluvial sedimentary system of the Vistre de la Fontaine during the Roman Empire is 206Pb/207Pb = 1.178 ± 0.002, much less radiogenic than the local geochemical background (student t-test, p < 0.05). The Pb isotope imprint introduced in excess (RXs) into the environment from this period can also be calculated by the following isotopic mixing equation (with R: isotopic ratio and Pb: lead content):

Fig. 5. A- and B- 206Pb/204Pb vs. 207Pb/204Pb of known lead ores that can fit the Geo2 isotopic imprint (Arribas and Tosdal Reference Arribas and Tosdal1994; Barnes et al. Reference Barnes, Shields, Murphy, Brill and Beck1974; Brevart et al. Reference Brevart, Dupre and Allegre1982; Pomiès et al. Reference Pomiès, Cocherie, Guerrot, Marcoux and Lancelot1988; Ruiz et al. Reference Ruiz, Arribas and Arribas2002; Craddock et al. Reference Craddock, Freestone, Gale, Meeks, Rothenberg, Tite, Craddock and Hughes1985; Hunt-Ortiz Reference Hunt-Ortiz2003; Krahn and Baumann Reference Krahn and Baumann1996; Le Guen and Lancelot Reference Le Guen and Lancelot1989; Le Guen et al. Reference Le Guen, Orgeval and Lancelot1991; Leach et al. Reference Leach, Premo, Lewchuk, Henry, Le Goff, Rouvier, Macquar, Thibieroz and Piestrzynski2001; Leach et al. Reference Leach, Macquar, Lagneau, Leventhal, Emsbo and Premo2006; Marcoux Reference Marcoux1986; Marcoux and Brill Reference Marcoux and Brill1986; Niederschlag et al. Reference Niederschlag, Pernicka, Seifert and Bartel-Heim2003; Rohl Reference Rohl1996; Rosman et al. Reference Rosman, Chisholm, Hong, Candelone and Boutron1997; Sangster et al. Reference Sangster, Outridge and Davis2000; Stos-Gale et al. Reference Stos-Gale, Gale, Houghton and Speakman1995; Stos-Gale et al. Reference Stos-Gale, Gale and Annetts1996; Stos-Gale and Gale Reference Stos-Gale and Gale2009; Swainbank et al. Reference Swainbank, Shepherd, Caboi and Masssoli-Novelli1982; Wagner et al. Reference Wagner, Gentner, Muller and Gale1979). Geo2 is the mean calculated isotopic signature of lead in excess during the Roman Empire sequence (“SD” = standard deviation). C- Determination of the excess Pb isotopic imprint from the linearity between Geo1 and Geo2 fields. (C. Flaux.)

The term “raw” is measured in the sample. The term “local” corresponds to the five samples of the sequence with the lowest lead content (considered as carrying the local geochemical background; samples 2, 5, 7, 8, and 11). PbXs (Pb in excess) = Pbraw - Pblocal. The mean calculated isotope signature of excess Pb (1.179 ± 0.002) is similar to that determined from Figure 5, as expected from the remarkably constant PbXs isotope imprint during the Roman sequence and beyond. This isotopic composition has been compared to a compilation of the isotopic signatures of lead deposits located in massifs where ancient works have been identified. The 206Pb/204Pb and 207Pb/204Pb PbXs signatures of the Geo2 field are used owing to the discrimination ability of the normalization to the non-radiogenic 204Pb isotope.Footnote 46 These PbXs isotopic ratios are calculated using the mixing equation. The different geographical provenances compose broad, sometimes scattered, and frequently overlapping isotopic domains, indicating isotopic heterogeneity of the ores (Fig. 5A). A closer analysis of 206Pb/204Pb vs. 207Pb/204Pb ratios of excess Pb reveals two possible regional sources within the uncertainty range of the Nîmes footprint (Fig. 5B): Great Britain (Northumberland, Devon, Staffordshire, and Gwynedd) and the southern Massif Central (Mont Lozère, Ségalas-Montagne Noire, southern Cévennes). The latter signature highlights the systematic prospecting of Cu-Pb-Ag deposits in the Montagne Noire during antiquityFootnote 47 and could confirm the southern Massif Central as a potential Pb-Ag mining region during the Roman period,Footnote 48 more firmly evidenced for the Middle Ages in metallurgical works and environmental deposits.Footnote 49 Alternatively, practices of salvaging and recycling implemented by the RomansFootnote 50 may have produced a mixture from several original sources that would be precluded from identification. Interestingly, the PbXs isotopic imprint recorded in Vistre de la Fontaine 2-2 Roman sediments is similar to that of ancient Roman lead pipes from Rome (Fig. 5A).Footnote 51
Lead isotope analysis also makes clear that this Roman-period contamination has been perpetuated until the modern era, most probably due to a remobilization of the “ancient reservoir,” which would have been redistributed by the alluvial dynamics along the meandering longitudinal profile of the Vistre de la Fontaine. As such, the excess Pb introduced at a given time into a fluvial environment could remain a passive source of contamination of local sediments for several thousand years, Pb being relatively stable in soils due to its insolubility in clean waterFootnote 52 and its resistance to leaching.Footnote 53 Such pervasive Roman Pb pollution in modern fluvial sediments has also been identified in the Tiber delta.Footnote 54
Conclusion
This study illustrates the riverine transport of diversified anthropogenic waste from the city of Nîmes towards its rural periphery since the Early Roman Empire, at a location 3 km downstream of the urban center, including remains from butchery, consumption of fruit, and combustion. These results confirm the role played by watercourses in evacuation of urban waste from ancient cities.Footnote 55 The human imprint is also characterized by heavy metal (Pb, Cu, and Zn) accumulation onto deposited sediment particles. It is likely that the Pb peak mirrors the expansion and retraction of the Nemausus water supply system during the Early Roman Empire. The Vistre de la Fontaine, originating from a sacred spring dedicated to the eponymous god of the city, Nemausus, became a sewer downstream and a vector, from the Roman city to the countryside, of visible and invisible waste. It has generated significant metallic palaeocontamination in the sediments of the convex bank of a meander of the river.
The perception of lead contamination remains topical: in the Nîmes area, little is known of the concentration of heavy metals in present-day watercourses.Footnote 56 The Vistre de la Fontaine 2-2 site shows that their presence may be a distant legacy, with local levels of long-lasting ancient Pb higher than the values for an impact on human health (sensitive Pb environmental impact value VCI of 400 ppm)Footnote 57 and well above the WHO/FAO permissible limit of 50 ppm.Footnote 58 Stable Pb isotopes show not only the possible geographic origin of Pb ores imported into the city of Nîmes during the Roman Empire (from the southern French Massif Central and/or Great Britain) but also the reworking of this ancient contamination into posterior alluvial deposits. This site shows that local present-day heavy metal anomalies may be inherited from industrial activities that date back to Roman Antiquity.
Acknowledgments
We thank three anonymous reviewers and the editor whose insightful comments helped improve the manuscript. We are grateful to Mélanie Errera, Loriane Besombes, and Florient Ortis for their great help during fieldwork. We also thank Christophe Pellecuer, chief curator of heritage of the French ministry of culture and communication, who fully promoted this study beyond its initial archaeological prescription. The study was supported by APIC 2021 funding from CEREGE. We thank Hélène Mariot for their careful maintenance of CEREGE's clean laboratory. The CEREGE group was supported by the French Investissement d'Avenir program through the Equipex ASTER-CEREGE.
Competing interests
The authors declare none.