Seafood has been a key component of human diets for thousands of years, and is rich in long chain (LC) n-3 PUFA, EPA (20 : 5n-3) and DHA (22 : 6n-3). Endogenous synthesis of EPA can occur in the human body to a limited extent when there is a dietary supply of the shorter chain, plant-derived n-3 PUFA, α-linolenic acid (ALA, 18 : 3n-3), but further conversion to DHA appears to occur only on a very small scale( Reference Burdge 1 ). Microscopic, single-celled marine organisms carry out de novo synthesis of LC n-3 PUFA resulting in concentrated amounts of EPA and DHA in the seafood that is commonly consumed by human subjects further up the marine food chain. In fact, the role of microalgae as primary producers of EPA and DHA may have been a key driver that enabled the evolutionary adaptation response of early Homo sapiens to environmental pressures. A plausible theory posits that an increased reliance on marine resources by early human subjects began in Africa about 140 000 years ago and allowed the modern human brain to grow rapidly due to a ready supply of dietary DHA( Reference Bradbury 2 – Reference Broadhurst, Cunnane and Crawford 4 ). DHA comprises approximately 40 % of total PUFA in the mammalian central nervous system( Reference Broadhurst, Cunnane and Crawford 4 ) and proponents of the marine/brain theory postulate that rapid encephalisation and increasing neural plasticity opened the way to behavioural innovations that enabled population expansion along the African coast and beyond( Reference Crawford, Bloom and Broadhurst 5 ).
An adequate supply of LC n-3 PUFA is vital for development and maintenance of the nervous system, a topic that has been comprehensively reviewed elsewhere( Reference Michael-Titus and Priestley 6 – Reference Dyall 9 ). A functioning central and peripheral nervous system, particularly the autonomic nervous system comprising sympathetic and parasympathetic nerves, is also essential for cardiovascular health, including homeostasis of blood pressure and heart rate (HR) by innervation of blood vessels and the pacemaker respectively. Marine LC n-3 fatty acids profoundly influence cardiovascular function (HR, blood pressure), by influencing neuronal function in the hypothalamus, sympathetic and parasympathetic neurons and the intrinsic cardiac nervous system by neurotrophic and neuroprotective mechanisms( Reference Robson, Dyall and Sidloff 10 ), including anti-inflammatory mechanisms( Reference Calder 11 , Reference Bazan, Molina and Gordon 12 ). Accumulated evidence to date suggests that dietary fish consumption is protective against cardiac mortality( Reference Zheng, Huang and Yu 13 ) and the potentially anti-arrhythmic effects of LC n-3 PUFA at relatively low doses are likely to be a significant contributor to these observed effects( Reference Albert, Campos and Stampfer 14 , Reference Marchioli, Barzi and Bomba 15 ).
Organisations with the authority to set dietary guidelines across the world are in agreement that inclusion of fish in the diet, particularly oily fish, or supplemental fatty acids derived from fish, is likely to be protective against CVD( 16 ). The link between marine fatty acids and CVD has been intensively investigated since the earliest reports appeared in the latter half of the last century of associations between high LC n-3 PUFA intakes and low rates of CVD in Canadian and Greenland Inuits( Reference Sinclair 17 – Reference Bang, Dyerberg and Nielsen 19 ). Epidemiological studies support the theory that fish consumption reduces the risk of CHD mortality( Reference Zheng, Huang and Yu 13 , Reference Mozaffarian and Wu 20 ) and meta-analyses of studies measuring blood or tissue levels of EPA + DHA at baseline have shown inverse associations with risk of coronary events( Reference Chowdhury, Warnakula and Kunutsor 21 ). The lack of effect in more recent primary and secondary prevention randomised controlled trials of fish oil supplementation( Reference Rizos, Ntzani and Bika 22 ), in contrast to earlier well-known trials( Reference Marchioli, Barzi and Bomba 15 , Reference Burr, Fehily and Gilbert 23 , Reference Tavazzi, Maggioni and Marchioli 24 ) has been debated at length( Reference von Schacky 25 – Reference Rice, Bernasconi and Maki 28 ). Factors such as reduced bioavailability of supplemental oils if consumed with low-fat meals or no food at all, concomitant medications obscuring therapeutic benefit (e.g. statins) and the amount of EPA and DHA already present in the body tissues, may have been critical determinants of clinical efficacy. A critical question is whether individuals with a risk profile indicating increased cardiovascular risk due to a low EPA and DHA tissue status, might benefit more from dietary LC n-3 PUFA supplementation than those whose tissue fatty acid profile is relatively enriched with EPA and DHA. Only 23 % of UK adults aged 19–64 report consuming oily fish and if this is accurate, it presents the possibility that a large proportion of the UK population may have sub-optimal concentrations of LC n-3 PUFA in their tissues and may be at increased risk of CHD mortality( Reference Zheng, Huang and Yu 13 ). Dietary intakes of fish and fish oil fatty acids vary widely for a variety of reasons, including lifestyle choices, for example vegetarianism, access to food/food security, food preferences and religious dietary restrictions. Furthermore, individual dietary requirements for fish oil fatty acids are likely to vary due to genetic variation (for example polymorphisms in the desaturase and elongase genes( Reference Smith, Follis and Nettleton 29 – Reference AlSaleh, Maniou and Lewis 31 )) and possibly background inflammatory burden, which may also be partly genetically determined( Reference Grenon, Conte and Nosova 32 ). The aim of this review is to examine what we know about the consequence of low dietary EPA and DHA intakes and to consider what the cardiovascular health impact might be in otherwise healthy populations.
ω-3 Index and cardiovascular health
A widely accepted method to assess an individual's medium-term dietary intakes of LC n-3 PUFA, is by measuring the percentage of erythrocyte membrane fatty acids (erythrocyte phospholipids) that are EPA and DHA, which correlates closely with the EPA + DHA content in cardiac tissue( Reference Harris, Sands and Windsor 33 ). Proportions of EPA + DHA in erythrocyte phospholipids have been identified as an independent predictor of CVD risk( Reference Harris and Von Schacky 34 , Reference Kleber, Delgado and Lorkowski 35 ). Erythrocyte EPA + DHA as a % of total erythrocyte fatty acids is termed the ω-3 index, with ≥8 % considered to be associated with the maximum protective effect( Reference Harris and Von Schacky 34 ). Incorporation of supplemented EPA + DHA into erythrocyte membranes occurs in a dose–response fashion and significant increases can be measured over a supplementation period of 2–12 months at relatively low doses akin to intake of 1–4 portions of oily fish per week( Reference Browning, Walker and Mander 36 ). The effects of age and sex on the efficiency of incorporation of supplemental EPA and DHA in blood cells and plasma fractions seem to be relatively minor( Reference Walker, Browning and Mander 37 ), but there may be an increased efficiency of uptake in individuals with lower baseline intakes of LC n-3 PUFA( Reference Cao, Schwichtenberg and Hanson 38 ) and potentially at times of greater need such as during pregnancy( Reference Dunstan, Mori and Barden 39 ). Reported average ω-3 indices vary widely in human populations that are identified as being omnivorous, most likely representing the fact that meat-consumers who eat very little fish will have much lower proportions of EPA and DHA in their membrane lipids than meat-consumers who also regularly consume fish, whereas reported ω-3 indices in vegans are distinctly lower compared with meat and fish-eaters (Table 1). Observational evidence suggests that low whole blood or erythrocyte membrane levels of EPA and DHA are associated with a greater risk of sudden cardiac death, primary cardiac arrest, acute coronary syndrome( Reference Albert, Campos and Stampfer 14 , Reference Siscovick, Raghunathan and King 40 , Reference Block, Harris and Reid 41 ) and a meta-analysis of prospective cohort studies that measured circulating fatty acids showed associations between higher EPA and DHA and lower relative risks of coronary outcomes( Reference Chowdhury, Warnakula and Kunutsor 21 ).
Table 1. Erythrocyte EPA and DHA (% or absolute concentrations) of meat- and fish-consumers (omnivores), vegetarians and vegans

Wt%, weight percentage of the sum of fatty acids; mol%, mole percentage of the sum of fatty acids.
* A vegetarian was defined as someone who ate no meat and not more than one fish meal a month.
Cardiovascular health of populations with low ω-3 index: vegetarians and vegans
Evidence from prospective cohort studies with a high proportion of vegetarians and vegans suggests that they are less likely to develop CHD than meat- and fish-consumers. For example, a 1999 meta-analysis of five prospective studies (USA, UK, Germany) reported that vegetarians had 24 % lower mortality from CHD (specific to those who followed diet for >5 years)( Reference Key, Fraser and Thorogood 42 ). Additional prospective cohorts included in more recent meta-analyses agreed with earlier findings( Reference Huang, Yang and Zheng 43 , Reference Kwok, Umar and Myint 44 ). Reduced risk of CHD appears to be driven by lower blood LDL cholesterol, blood pressure and possibly lower BMI in vegans/vegetarians( Reference Key, Appleby and Rosell 45 – Reference Appleby, Davey and Key 47 ). Taking this evidence at face value it appears that a vegetarian/vegan diet may be a cardio-protective diet. It has been suggested that there seems to be a minimum basal rate of conversion of ALA to DHA, which is sufficient to maintain cardiovascular health where dietary sources of pre-formed LC n-3 PUFA are lacking( Reference Sanders 48 , Reference Sanders 49 ). Furthermore, authors of an observational study, using statistical estimates of ALA to longer chain n-3 PUFA conversion rates, suggested that conversion might actually be increased in non-fish eaters compared with fish-eaters. This would account for the relatively low margin of difference in ω-3 indices of fish-eaters compared with non-fish eaters( Reference Welch, Shakya-Shrestha and Lentjes 50 ), although conversion rates to DHA specifically were not clear.
Closer inspection of the observational evidence for coronary risk in vegetarians/vegans, raises the question of whether it is valid to translate these findings to the wider population. Vegetarian/vegan cohorts vary in their motivations for dietary choice (religious, health, animal welfare), which may converge with other distinct lifestyle behaviours that may affect cardiovascular health (physical exercise, practising mindfulness/meditation/prayer, prevalence of alcohol and drug use). This concern seems particularly pertinent to the Seventh Day Adventist cohorts. Kwok et al. applied sub-group testing in their meta-analysis of seven prospective cohort studies that evaluated mortality and clinical cardiovascular outcomes in vegetarian populations compared with non-vegetarian controls and revealed that the overall pooled difference in risk of cardiac events was mainly driven by the Seventh Day Adventist cohorts( Reference Kwok, Umar and Myint 44 ), suggesting that the results from this particular population might not be generalisable to other populations. Furthermore, the definition of vegetarian in Seventh Day Adventist cohorts tends to be inconsistent with the generally accepted definition of eating no meat or fish. Seventh Day Adventist cohorts incorporated into the pooled analyses( Reference Key, Fraser and Thorogood 42 , Reference Kwok, Umar and Myint 44 ) included those who ate meat <1/week or less with no mention of what their fish intakes were( Reference Snowdon 51 , Reference Beeson, Mills and Phillips 52 ), or were otherwise not clearly defined( Reference Berkel and de Waard 53 ). It is therefore possible that the Adventist cohorts were also fish-consumers and may have had higher ω-3 indices than ‘true’ vegetarians/vegans who do not eat any fish. The more recent Adventist Health Study 2 clearly defined participants as vegans, lacto-ovo vegetarians, pesco-vegetarians, semi-vegetarians and non-vegetarians( Reference Orlich, Singh and Sabaté 54 ). The results of Adventist Health Study 2 showed that the reduced risk in CHD mortality was not quite statistically significant (hazard ratio 0·81, 95% CI 0·64, 1·02) for all vegetarians combined. Further analysis of dietary sub-groups revealed that the reduced risk was significant in pesco-vegetarians when both sexes were combined (hazard ratio 0·65, 95% CI 0·43, 0·97) but not vegans (hazard ratio 0·90, 95% CI 0·60, 1·33) or lacto-ovo vegetarians (hazard ratio 0·90, 95% CI 0·76, 1·06).
The UK vegetarian/vegan cohorts might be considered more generalisable to the broader population since they are not affiliated with a religious faith and may be more diverse in terms of demographics and lifestyle behaviours. Furthermore, vegetarians and vegans were clearly defined as not eating meat or fish( Reference Appleby, Davey and Key 47 , Reference Appleby, Crowe and Bradbury 55 , Reference Crowe, Appleby and Travis 56 ) and the vegetarian and vegan men from the EPIC-Oxford cohort were reported to have lower EPA and DHA proportions of total plasma fatty acids compared with omnivores, providing an objective biomarker of low fish intake( Reference Rosell, Lloyd-Wright and Appleby 57 ). Although vegetarians/vegans had a lower incidence of CHD in the UK EPIC-Oxford cohort( Reference Crowe, Appleby and Travis 56 ), there was no difference in CHD mortality compared with controls who ate meat and fish (death rate ratio 0·83, 95% CI 0·59, 1·18)( Reference Key, Appleby and Spencer 58 ). The latter study may have been statistically underpowered, but a more recent combined analysis of mortality rates (total 644 CHD deaths) reported that there was no significant difference in CHD mortality in UK vegans/vegetarians up to 90 year (nor 75 year) compared with comparable regular meat-eaters (hazard ratio 0·99, 95% CI 0·79, 1·23)( Reference Appleby, Crowe and Bradbury 55 ), who consumed meat ≥5 times/week as well as fish.
In translating these observations to the question of the cardiovascular health impact of low EPA + DHA tissue status, it is impossible to cleanly dissect the influence of dietary LC n-3 fatty acid intake from other dietary influences. For example, vegetarian/vegan diets are lower in SFA, higher in fibre, but can also be deficient in bioavailable iron, vitamin B12 and vitamin D. Low serum 25-hydroxyvitamin D concentrations are also associated with increased CVD mortality( Reference Schöttker, Jorde and Peasey 59 ). Furthermore, the nature of the comparator group is an important consideration, particularly as health-conscious participants were recruited to UK cohorts who tended to have lower intakes of dietary animal protein compared with Adventist non-vegetarian/non-vegans( Reference Crowe, Appleby and Travis 56 ). However, equivalent CHD mortality rates could alternatively signify that the vegetarian/vegan diet is also associated with other raised CHD risk factors (e.g. low ω-3 index) that might negate the otherwise cardio-protective effects of a vegetarian/vegan diet. It is hypothesised here that long-term low tissue LC n-3 PUFA levels may counterbalance the athero-protective qualities of vegetarian/vegan diets in the sum effect on overall CHD mortality risk. The implications of this for the sizeable non-fish-eating, non-vegetarian/non-vegan population are more serious. Low tissue EPA and DHA levels in a less health-conscious meat-eating population might have a more deleterious effect on cardiovascular health, such as increasing risk of cardiac arrhythmia, against the background of higher LDL-cholesterol, blood pressure and BMI. Fig. 1 summarises the counterbalance of dietary factors that may positively or negatively influence risk of CHD mortality in non-fish eaters. The next sections will address the cardiovascular risk factors that may mediate increased risk of CHD mortality in populations with very low LC n-3 PUFA intakes, including low HR variability (HRV) and a reduced capacity to regulate inflammatory responses.

Fig. 1. Theoretical schematic showing how low long chain n-3 PUFA intakes may oppose the cardioprotective effects of vegetarian/vegan diets, resulting in an equivalent risk of CHD mortality (A). The majority of the UK population eats little or no fish and may be at risk of low ω-3 status. Without the counterbalancing cardio-protective qualities of a vegetarian/vegan diet, this could lead to an increased risk of CHD mortality mediated by arrhythmia (B). Picture of artery attributed to ‘Blausen gallery 2014’( 136 ).
Heart rate variability and risk of arrhythmia
Low HRV indicates a reduced capacity to regulate HR in response to internal and external stressors and demands, and is associated with mortality after a myocardial infarction( Reference Vaishnav, Stevenson and Marchant 60 – 62 ) and risk of cardiac events in the general population( Reference Tsuji, Larson and Venditti 63 ). Low HRV is considered to be a predictor of sudden cardiac death( Reference Algra, Tijssen and Roelandt 64 ). Therefore, measurement of parameters of short- and long-term variability in heart beat intervals (RR intervals, or interbeat intervals) using Holter monitors or less intrusive HRV/accelerometry integrated chest-worn monitors (e.g. Actiheart, eMotion Faros), can be a useful non-invasive method to assess the adaptability/resilience of the heart. HRV is partly under the control of the autonomic nervous system, which receives afferent impulses from, and exerts efferent activity to, the intrinsic cardiac nervous system, which interacts with the sino-atrial node. Consequently, variability in HR reflects the sum effect of sympathetic and parasympathetic outflow. Low HRV is associated with a high degree of sympathetic activity (which raises HR thus reducing the capacity to self-regulate in response to demand) and suppressed parasympathetic activity (vagal activity slows HR). Higher LC n-3 PUFA tissue status or fish consumption has been positively associated with HRV( Reference Christensen 65 , Reference Mozaffarian, Stein and Prineas 66 ). Mixed results have been obtained in previous investigations into the effect of n-3 PUFA on HRV in healthy subjects( Reference Mozaffarian, Stein and Prineas 66 – Reference Christensen, Christensen and Dyerberg 69 ), haemodialysis patients( Reference Svensson, Schmidt and Jorgensen 70 ), subjects with previous myocardial infarction( Reference Christensen, Korup and Aaroe 71 – Reference Hamaad, Kaeng Lee and Lip 74 ) and patients with epilepsy( Reference DeGiorgio, Miller and Meymandi 75 ). These studies were typically only 12 weeks long, the durations of HR monitoring differed widely and relatively high doses of n-3 PUFA ranged from 1 to 6·6 g. Furthermore, comparisons between studies are difficult due to different HRV parameters being reported, varying study designs, and methodological inconsistencies. Nevertheless, fish oil supplementation has been shown to increase a parameter of beat-to-beat (vagally regulated) HRV in a meta-analysis of randomised controlled trials( Reference Xin, Wei and Li 76 ), supporting the role of adequate tissue EPA + DHA status in preventing arrhythmic events. Thus autonomic balance may be improved by increased EPA and DHA membrane levels, although a direct effect on pacemaker activity independently of the autonomic nervous system may also occur. Increasing LC n-3 PUFA content in rabbit cardiomyocyte membranes decreases HR in isolated hearts and reduces pacemaker activity and pacemaker current in sinoatrial node cells( Reference Verkerk, den Ruijter and Bourier 77 , Reference Billman 78 ). In accord with its effects on HRV in clinical trials, fish oil supplementation also reduces HR in human studies( Reference Mozaffarian, Geelen and Brouwer 79 ).
In summary, fish consumption and to some extent fish oil supplementation, is associated with reductions in HR and increased HRV, which would be predicted to reduce the risk of arrhythmia. However, the impact on HRV of consuming a LC n-3 PUFA-free diet was unexplored until recently, when we addressed this question in a cross-sectional study in vegans and age- and BMI-matched omnivores, measuring the average duration of interbeat intervals (the reciprocal of HR), HRV, erythrocyte phospholipid fatty acids, plasma fatty acids and oxygenated PUFA metabolites( Reference Pinto, Sanders and Kendall 80 ). Vegans had lower day-time beat-to-beat HRV and shorter day-time interbeat intervals compared with omnivores, adjusted for age, sex, BMI and physical activity levels by accelerometry. Twenty-four hour HRV was higher in vegans due to the greater night–day differences in vegans compared with omnivores, which was mainly due to the relatively lower HRV during the day rather than higher HRV at night in vegans; no differences between groups during nocturnal sleep were observed. This could indicate a greater predominance of sympathetic regulation (associated with reduced parasympathetic activity) during waking hours due to an exaggerated response to stimuli, or a direct effect of low LC n-3 PUFA membrane concentrations in cardiac cells on the pacemaker that manifested in altered cardiac function under conditions of stimulation. There were no differences in serum vitamin D or B12 status between the groups, factors which may also have impacted on HRV. Although these observations only demonstrate an associative link, n-3 PUFA tissue status is implicated as a strong candidate for being the key factor in determining the disparate HRV patterns during waking hours observed in vegans and omnivores.
Dampening and resolving inflammation
Both n-6 and n-3 PUFA in cell membranes can be metabolised into an array of pro- and anti-inflammatory metabolites that are likely to influence prevention of arrhythmia by influencing myocyte cell-signalling and also autonomic function. Chronic inflammation is the key underlying factor in the lifelong accrual of vascular lesions that eventually leads to atherosclerosis and CHD, and LC n-3 PUFA supplementation appears to improve plaque stability( Reference Thies, Garry and Yaqoob 81 ). Although the role of inflammation in initiating and propagating atherosclerosis is at the crux of CHD, chronic inflammation probably also increases coronary risk independently of atherosclerosis by impairing neuronal function( Reference Dyall and Michael-Titus 82 ) and myocyte membrane properties( Reference Papaioannou, Verkerk and Amin 83 ), thereby having a direct influence on cardiac electrophysiology and risk of arrhythmia( Reference Lanza, Sgueglia and Cianflone 84 , Reference Malave, Taylor and Nattama 85 ). LC n-3 PUFA levels in plasma and erythrocytes are inversely associated with circulating markers of inflammation (C-reactive protein, IL-6, IL-1 receptor antagonist, TNF receptor 2, transforming growth factor-β)( Reference Farzaneh-Far, Harris and Garg 86 – Reference Fontes, Rahman and Lacey 88 ). LC n-3 PUFA may lower inflammatory burden in a number of ways: by inhibiting the conversion of LA to more pro-inflammatory eicosanoids by substrate competition, being converted themselves to less potent pro-inflammatory eicosanoids, modulation of transcription factor activation, inhibiting the expression of vascular cell adhesion molecules and cytokines, and through the inflammation-resolving properties of their oxygenated metabolites( Reference Calder 11 ). The lack of sensitivity of measurements of circulating inflammatory biomarkers, and also the large variability due to hyper-responsivity to short-term infections, has up to now hindered efforts to build a body of evidence for a role of LC n-3 PUFA in modulating chronic, low-grade inflammation in the context of CVD( Reference Skulas-Ray 89 ). The relatively recent detection of a diverse array of oxygenated PUFA metabolites presents a whole new line of investigation with respect to the anti-inflammatory effects of marine fatty acids. Some of the lipid mediators derived from LC n-3 PUFA, termed specialised pro-resolving mediators (SPM), act in an autacoid manner to resolve inflammation (Fig. 2). SPM play a functional role in ending acute inflammatory events by inhibition of neutrophil influx to the site of trauma, counter-regulating pro-inflammatory cytokines and stimulating resolving macrophages to clear the products of the inflammatory response, thereby allowing the injured area to heal( Reference Serhan 90 ). Protectin-D1 (originally termed neuroprotectin) is thought to be important in inhibiting proinflammatory gene expression and promoting nerve regeneration in neural tissue, thereby protecting neurons from inflammation-related injury in a DHA-dependent manner( Reference Bazan, Molina and Gordon 12 , Reference Bazan, Musto and Knott 91 ). DHA-derived neuroprostanes, F4 isoprostane-like compounds formed non-enzymatically through free radical-catalysed reactions, are also thought to be significant markers of oxidative stress in neural tissue( Reference Galano, Lee and Gladine 92 ); furthermore, hepatic F4-neuroprostanes are negatively associated with the extent of atherosclerotic plaque( Reference Gladine, Newman and Durand 93 ), inhibit inflammatory cell signalling in macrophages( Reference Gladine, Laurie and Giulia 94 ) and may have cardiac arrhythmic effects( Reference Roy, Oger and Thireau 95 ).
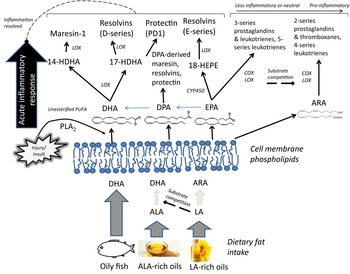
Fig. 2. Overview of the hypothesised role of membrane PUFA profiles in the production of pro-inflammatory and inflammation-resolving oxygenated lipid mediators. In addition to inhibition of arachidonic acid (ARA)-derived pro-inflammatory eicosanoid production, higher proportions of membrane phospholipid long chain (LC) n-3 PUFA may increase availability of LC n-3 PUFA available for enzymic oxygenation to lipid mediators that contribute to the resolution of an acute inflammatory response( Reference Serhan 90 ). ALA, α-linolenic acid; COX, cyclooxygenase; CYP450, cytochrome P450; HDHA, hydroxyl-DHA; HEPE, hydroxyeicosapentaenoic acid; LA, linoleic acid; LOX, lipoxygenase; PLA2, phospholipase A2; SPM, specialised pro-resolving mediators.
SPM, namely E- and D- series resolvins (RvD1 and RvE1), the DHA-derived (neuro)protectin-D1 and maresin (MaR1), are released in nano- and pico-molar concentrations at sites of local inflammation, can be detected in ex vivo cultured mononuclear cells following EPA + DHA supplementation( Reference Wang, Hjorth and Vedin 96 ) and sometimes resolvins can be detected in human plasma although concentrations are not responsive to supplementation( Reference Mas, Croft and Zahra 97 – Reference Mas, Barden and Burke 100 ). Large changes in other postprandial and fasting oxygenated PUFA metabolites have been reported in response to fish oil supplementation( Reference Barden, Mas and Croft 99 , Reference Schuchardt, Schmidt and Kressel 101 ). But the lipid mediator profiles of unsupplemented populations remain undetermined, particularly those who have very low or zero habitual dietary LC n-3 PUFA intakes. We investigated whether the vegans in our cross-sectional study also had lower circulating concentrations of oxygenated EPA and DHA metabolites, since this might have implications for their ability to resolve acute and chronic inflammation. It was observed that vegans had significantly lower plasma concentrations of EPA- and DHA-derived lipid mediators compared with omnivores, including 18-HEPE, 17-HDHA and 14-HDHA, likely precursor markers for RvE1, RvD1 and MaR1 availability, respectively( Reference Pinto, Sanders and Kendall 80 ). Although circulating plasma concentrations of lipid mediators are likely to be an insensitive marker of capacity for autacoid release and activity of SPM in specific inflamed sites in the nervous and cardiovascular systems, higher concentrations of precursor markers of SPM bioavailability (18-HEPE, 17-HDHA and 14-HDHA) may indicate greater capacity for conversion to SPM at times of need, with clear functional implications for populations with low tissue EPA and DHA stores.
The accumulating evidence suggests that tissue LC n-3 PUFA are crucial in moderating inflammatory responses in the cardiovascular system. Consequently individuals with no dietary intake of marine n-3 PUFA may be at risk of incurring inflammatory-related neuronal and cardiovascular damage at a greater rate than fish-consumers. An adverse LC n-3 PUFA-derived lipid mediator profile may also be implicated in impaired haemostatic function in vegetarians( Reference Mezzano, Muñoz and Martínez 102 ). However, presently these ideas remain strictly hypothetical. Health-conscious non-fish-consuming populations may also have a greater bioavailability of ALA- and linoleic acid (LA; 18 : 2n-6)-derived lipid mediators that may have cytoprotective effects( Reference Ramsden, Ringel and Feldstein 103 , Reference Kumar, Gupta and Anilkumar 104 ), although this may not be applicable to the majority of the non-fish-eating population. Few of these lipid mediators have been fully characterised regarding their functional effects, but evidence in animal and cell models to date suggests that plant PUFA-derived oxygenated lipid mediators comprise a complex array of diverse bioactive molecules that may also induce a range of physiological effects in various tissues( Reference Choque, Catheline and Rioux 105 – Reference Nicolaou, Mauro and Urquhart 107 ).
Alternative sources for non-fish consumers?
Soya and rapeseed oils are particularly rich in ALA, with the main dietary sources of ALA being cereal-based products, cooking oils and spreading fats, and vegetables. The high concentrations in oilseed crops presents a potentially easy, sustainable and cheap alternative source of n-3 PUFA, but the evidence for a protective effect against CVD is weaker in comparison with marine n-3 fatty acids( Reference Fleming and Kris-Etherton 108 ). Populations in Europe and the USA consume 3–9 % of energy as LA( 16 , 109 ). Dietary intake of ALA has proven difficult to assess due to methodological limitations associated with accurate fatty acid composition data in food databases, but intakes are estimated to be much lower (0·3–0·8 % energy)( 16 , 109 ) than LA intakes, which would limit the amount of endogenous LC n-3 PUFA production from ALA( Reference Rosell, Lloyd-Wright and Appleby 57 ). Reports are conflicting as to whether vegans/vegetarians consume more ALA than omnivores/fish-eaters( Reference Sanders 49 , Reference Welch, Shakya-Shrestha and Lentjes 50 , Reference Kornsteiner, Singer and Elmadfa 110 ). Dietary LA intakes on the other hand tend to be higher in vegetarians/vegans than omnivores( Reference Sanders 49 , Reference Welch, Shakya-Shrestha and Lentjes 50 ), which may also limit conversion of ALA to EPA through substrate competition( Reference Liou, King and Zibrik 111 ). Furthermore, supplementation with additional ALA in vegans appears to be ineffective in increasing conversion to LC n-3 PUFA( Reference Fokkema, Brouwer and Hasperhoven 112 ) and supplementation with pre-formed EPA and DHA may be necessary for cardioprotective benefit in non-fish-eating populations.
GM crops that can yield EPA and DHA are well-advanced along the experimental process( Reference Napier, Usher and Haslam 113 ), although the first human trial using LC n-3 PUFA-rich Camelina sativa oil has only just commenced at the time of writing. The main impetus for this bioengineering research was to find a way of producing EPA- and DHA-rich oil to replace fish oils to feed farmed fish( Reference Betancor, Sprague and Usher 114 ), but the development of transgenic oilseed crops, using genes from microalgae to synthesise EPA and DHA from shorter chain PUFA, could provide an alternative to fish oil for human supplementation and food fortification. The other, already commercially available, option is DHA-only and DHA + EPA-rich microalgal oil( Reference Sarter, Kelsey and Schwartz 115 ). These LC n-3 PUFA rich algal oils have been demonstrated to increase the DHA content of phospholipids efficiently and to have TAG- and blood pressure-lowering properties( Reference Maki, Yurko-Mauro and Dicklin 116 – Reference Theobald, Goodall and Sattar 120 ). Microalgal oils are also already being used to supplement infant formula and a limited range of adult foods but the limited scale of production and high costs presently precludes any efforts to apply to large populations( Reference Chauton, Reitan and Norsker 121 ). Furthermore, little is known about what dosage and which EPA : DHA ratio is likely to be cardio-protective in disease-free populations with low ω-3 indices( Reference Cottin, Sanders and Hall 122 ). High-DHA purified fish oils are slightly more effective than high-EPA equivalents in lowering blood pressure( Reference Mori, Bao and Burke 123 ). DHA-only algal oil consumed in combination with a fatty meal appears to avoid the less desirable effects of increasing postprandial concentrations of plasma F2 isoprostanes (markers of oxidative stress produced from arachidonic acid following non-enzymatic reaction with reactive oxygen species) compared with EPA + DHA containing fish oil( Reference Purcell, Latham and Botham 124 ). However, although retroconversion of DHA to EPA is estimated at between 8 and 14 %( Reference Conquer and Holub 125 ), the functional consequences of the lack of dietary EPA against the background of plentiful dietary DHA are not known and may be significant if EPA-derived lipid mediators have any tissue specific roles in cytoprotection. It is equally possible that EPA supplementation is less effective and that DHA-only oils are sufficient to reduce risk of lethal arrhythmia. On the whole, fish oil supplementation studies to date have not considered baseline ω-3 status, which in hindsight has hindered progress towards a robust body of evidence that can be used to formulate dietary guidelines( Reference Dwyer, Rubin and Fritsche 26 ) and consequently necessitates a fresh approach to gathering new evidence on whether non-fish-consuming populations would be at reduced risk of CHD if they had access to alternative dietary sources of EPA and DHA.
Conclusion
Observational evidence suggests that non-fish-consumers may be at greater risk of CHD mortality and low EPA + DHA tissue status is associated with increased risk of cardiac events, with arrhythmia being implicated as the most likely common risk factor. Preliminary evidence presented here suggests that vegans, who have an ω-3 index of approximately 2–3 %, may have an impaired capacity to regulate HR in response to physiological demands during the day-time, and they have a distinct lipidomic profile compared with omnivores, with markedly lower circulating concentrations of LC n-3 PUFA-derived lipid mediators. However, there is a large gap in the literature regarding the effects of EPA and DHA supplementation in populations characterised by having a low ω-3 index and the relative roles of EPA and DHA remain to be determined before supplementation can be recommended. The question of determining the safest and most effective EPA : DHA ratio for optimum cardio-protection when delivering these fatty acids outside the matrix of the whole seafood seems to be imperative in the context of the small proportion of the population who are actually meeting the fish intake guidelines. Moreover, this question is even more important when considering the increasingly pressing challenges of sustainability of wild fish stocks, and the huge estimated shortfall in total EPA + DHA presently available for human consumption if global human dietary requirements are to be met( Reference Salem and Eggersdorfer 126 ).
Acknowledgements
The author is grateful to Ana-Margarida Pinto, Tom Sanders, Ana Nicolaou, Alexandra Kendall and Caroline Wheeler-Jones for collaborations and discussions that inspired the content of this review and to Sarah Berry for critical review of the initial manuscript draft.
Financial Support
None.
Conflicts of Interest
None.
Authorship
W. H. wrote the manuscript.