32 results
5 - Quantum Physics with Explicit Time Dependence
-
- Book:
- A Computational Introduction to Quantum Physics
- Published online:
- 24 April 2024
- Print publication:
- 25 April 2024, pp 78-100
-
- Chapter
- Export citation
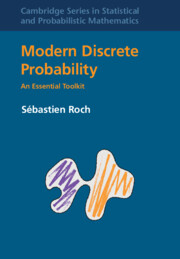
Modern Discrete Probability
- An Essential Toolkit
-
- Published online:
- 14 December 2023
- Print publication:
- 18 January 2024
FULLY 3D FLUID OUTFLOW FROM A SPHERICAL SOURCE
- Part of
-
- Journal:
- The ANZIAM Journal / Volume 64 / Issue 2 / April 2022
- Published online by Cambridge University Press:
- 10 August 2022, pp. 149-182
-
- Article
-
- You have access
- Open access
- HTML
- Export citation
MODELLING OF WITHDRAWAL OF A STRATIFIED FLUID FROM A POROUS MEDIUM
- Part of
-
- Journal:
- Bulletin of the Australian Mathematical Society / Volume 104 / Issue 2 / October 2021
- Published online by Cambridge University Press:
- 12 April 2021, pp. 349-350
- Print publication:
- October 2021
-
- Article
-
- You have access
- Export citation
7 - Numerical Methods for Differential Equations
- from Part II - Numerical Methods
-
- Book:
- Matrix, Numerical, and Optimization Methods in Science and Engineering
- Published online:
- 18 February 2021
- Print publication:
- 04 March 2021, pp 376-406
-
- Chapter
- Export citation
Statistical evaluation of spectral methods for anomaly detection in static networks
-
- Journal:
- Network Science / Volume 7 / Issue 3 / September 2019
- Published online by Cambridge University Press:
- 23 September 2019, pp. 319-352
-
- Article
- Export citation
The Landau-Zener Transition and the Surface Hopping Method for the 2D Dirac Equation for Graphene
- Part of
- Equations of mathematical physics and other areas of application
- Quantum theory
- Applications to specific physical systems
- Approximation methods and numerical treatment of dynamical systems
- General mathematical topics and methods in quantum theory
- Partial differential equations, initial value and time-dependent initial-boundary value problems
-
- Journal:
- Communications in Computational Physics / Volume 21 / Issue 2 / February 2017
- Published online by Cambridge University Press:
- 07 February 2017, pp. 313-357
- Print publication:
- February 2017
-
- Article
- Export citation
THE FORMATION OF LARGE-AMPLITUDE FINGERS IN ATMOSPHERIC VORTICES
- Part of
-
- Journal:
- The ANZIAM Journal / Volume 57 / Issue 4 / April 2016
- Published online by Cambridge University Press:
- 28 March 2016, pp. 395-416
-
- Article
-
- You have access
- Export citation
FRACTIONAL MODELS IN SPACE FOR DIFFUSIVE PROCESSES IN HETEROGENEOUS MEDIA WITH APPLICATIONS IN CELL MOTILITY AND ELECTRICAL SIGNAL PROPAGATION
- Part of
-
- Journal:
- Bulletin of the Australian Mathematical Society / Volume 93 / Issue 3 / June 2016
- Published online by Cambridge University Press:
- 10 February 2016, pp. 518-520
- Print publication:
- June 2016
-
- Article
-
- You have access
- Open access
- Export citation
Spectral methods plasticity modeling for fatigue damageestimation: experimental application – uniaxial case
-
- Journal:
- Mechanics & Industry / Volume 15 / Issue 3 / 2014
- Published online by Cambridge University Press:
- 30 May 2014, pp. 233-242
- Print publication:
- 2014
-
- Article
- Export citation
A Time Splitting Space Spectral Element Method for the Cahn-Hilliard Equation
-
- Journal:
- East Asian Journal on Applied Mathematics / Volume 3 / Issue 4 / November 2013
- Published online by Cambridge University Press:
- 28 May 2015, pp. 333-351
- Print publication:
- November 2013
-
- Article
- Export citation
On the convergence of the stochastic Galerkin method for random elliptic partial differential equations∗
-
- Journal:
- ESAIM: Mathematical Modelling and Numerical Analysis / Volume 47 / Issue 5 / September 2013
- Published online by Cambridge University Press:
- 09 July 2013, pp. 1237-1263
- Print publication:
- September 2013
-
- Article
- Export citation
Finite volume and pseudo-spectral schemes for the fully nonlinear 1D Serre equations
-
- Journal:
- European Journal of Applied Mathematics / Volume 24 / Issue 5 / October 2013
- Published online by Cambridge University Press:
- 24 May 2013, pp. 761-787
-
- Article
- Export citation
Mass and Volume Conservation in Phase Field Models for Binary Fluids
-
- Journal:
- Communications in Computational Physics / Volume 13 / Issue 4 / April 2013
- Published online by Cambridge University Press:
- 03 June 2015, pp. 1045-1065
- Print publication:
- April 2013
-
- Article
- Export citation
Distinguishing and integrating aleatoric and epistemic variation in uncertainty quantification∗
-
- Journal:
- ESAIM: Mathematical Modelling and Numerical Analysis / Volume 47 / Issue 3 / May 2013
- Published online by Cambridge University Press:
- 29 March 2013, pp. 635-662
- Print publication:
- May 2013
-
- Article
- Export citation
SELECTIVE WITHDRAWAL OF A TWO-LAYER VISCOUS FLUID
-
- Journal:
- The ANZIAM Journal / Volume 53 / Issue 4 / April 2012
- Published online by Cambridge University Press:
- 08 February 2013, pp. 253-277
-
- Article
-
- You have access
- Export citation
INVISCID AND VISCOUS MODELS OF AXISYMMETRIC FLUID JETS OR PLUMES
- Part of
-
- Journal:
- The ANZIAM Journal / Volume 53 / Issue 3 / January 2012
- Published online by Cambridge University Press:
- 12 November 2012, pp. 228-250
-
- Article
-
- You have access
- Export citation
Mortar spectral method in axisymmetric domains
-
- Journal:
- ESAIM: Mathematical Modelling and Numerical Analysis / Volume 47 / Issue 1 / January 2013
- Published online by Cambridge University Press:
- 31 July 2012, pp. 33-55
- Print publication:
- January 2013
-
- Article
- Export citation
RAYLEIGH–TAYLOR INSTABILITIES IN AXI-SYMMETRIC OUTFLOW FROM A POINT SOURCE
- Part of
-
- Journal:
- The ANZIAM Journal / Volume 53 / Issue 2 / October 2011
- Published online by Cambridge University Press:
- 05 July 2012, pp. 87-121
-
- Article
-
- You have access
- Export citation
Convergence Analysis of the Spectral Methods for Weakly Singular Volterra Integro-Differential Equations with Smooth Solutions
-
- Journal:
- Advances in Applied Mathematics and Mechanics / Volume 4 / Issue 1 / February 2012
- Published online by Cambridge University Press:
- 03 June 2015, pp. 1-20
- Print publication:
- February 2012
-
- Article
- Export citation