Introduction
Productivity and feed efficiency are the basic prerequisites for profitable swine production. Pigs are often fed nutrient- and energy-dense diets to meet such requirements in a cost-efficient way. The integration of fiber components in complete feeds for pigs may require additional technical effort and lead to faster satiation in the animals. This practice contradicts the natural feeding behavior of pigs, and may cause stress and behavioral problems which are considered important factors affecting welfare and health (Tokach et al., Reference Tokach, Menegat, Gourley and Goodband2019). Therefore, welfare-oriented feeding concepts often include the administration of complete feed rich in dietary fiber (DF) or the additional administration of roughage as a satiation feed. The chemical structure of the various fiber types is crucial for the numerous direct and indirect physiological effects of DF and has been extensively reviewed (Jha and Berrocoso, Reference Jha and Berrocoso2015; Navarro et al., Reference Navarro, Abelilla and Stein2019; Li et al., Reference Li, Yin, Tan, Chen, Zhang, Li and Ma2021), whereas the physical properties have been studied sparsely (Molist et al., Reference Molist, Manzanilla, Perez and Nyachoti2012). Depending on the chemical composition, but in particular on the solubility and the microbial fermentability, DF can have very different effects on the digestive tract and metabolism of pigs. Soluble fiber (SF) sources contain mix-linkage glucans, hemicelluloses, arabinoxylans, xyloglucans, galactomannans, pectins, gums, guar, and agar and non-digestible fructo- and galactooligosaccharides. Partly insoluble fibers (ISF) are composed of cellulose, lignin, and different forms of resistant starches (Gemen et al., Reference Gemen, de Vries and Slavin2011; Williams et al., Reference Williams, Grant, Gidley and Mikkelsen2017). DF occurs naturally in almost all plant-based feedstuffs and can also be obtained from by-products of industrial food and beverage processing, and can therefore be used as a sustainable feed component. Although DF is not considered an essential nutrient, various performance, behavior, and health-related properties are affected, depending on the quantity and quality of the fiber source. Most interestingly, DF has an impact on pigs through specific effects on the digestive tract, intestinal microbiota, and immune functions, and thereby may protect against certain intestinal disorders, contributing to better health (Lindberg, Reference Lindberg2014). In contrast to the situation in rodent animal models, only a few studies in pigs are explicitly devoted to the mechanistic effects of fiber intake. However, the mechanistic aspect of DF is extremely important for explaining its physiologic effects on the digestive tract, microbiota, and immunity.
Here, we summarize the studies on DF in pigs as important food-producing animals, specifically considering pregnant and lactating sows, the sow–piglet couple, weaner piglets, gilts, and fattening pigs and the mode of action of DF.
DF in gestating and lactating sows
Impact of DF on performance of sows
For sows and their offspring, DF has the potential to influence physiology and health (Table 1). Several studies investigated the effect of DF inclusion in the diet on sow performance, including physiological parameters of reproduction and progeny. Particularly interesting are the effects of DF on feed intake, especially during lactation as higher feed intake in this period may promote health and performance in sows and piglets (Farmer et al., Reference Farmer, Robert and Matte1996). An impact of DF on reproductive performance has been observed as early as the onset of puberty, which was demonstrated 15.6 days earlier in gilts fed with diets enriched with soluble DF until mating. Additionally, their litters were characterized by a reduced number of growth-retarded piglets and these litters were more uniform (Zhuo et al., Reference Zhuo, Shi, Lv, Hua, Zhou, Che, Fang, Lin, Xu, Li, Feng and Wu2017). Regarding successful insemination, feeding sows diets rich in DF supplemented with inulin and cellulose, sugar beet pulp (SBP), wheat bran (WB) or lupins demonstrated enhancing effects on oocyte maturity, quality, and ovarian follicle reserve (Ferguson et al., Reference Ferguson, Slevin, Hunter, Edwards and Ashworth2007; Weaver et al., Reference Weaver, Kelly, Kind, Gatford, Kennaway, Herde and van Wettere2013; Cao et al., Reference Cao, Zhuo, Gong, Tang, Li, Li, Yang, Xu, Li, Che, Lin, Feng, Fang and Wu2019). However, no differences were observed in ovulation rate and litter size when gestating sows were fed with diets with 30% oat bran, 12% wheat straw, or 21% soybean hulls (Renteria-Flores et al., Reference Renteria-Flores, Johnston, Shurson, Moser and Webel2008). The number and viability of piglets increased when sows were provided 20–30% hydrolyzed straw meal (Bergner, Reference Bergner1988), 38% SBP and 5.6% soybean hulls (van der Peet-Schwering et al., Reference van der Peet-Schwering, Kemp, Binnendijk, den Hartog, Spoolder and Verstegen2003), or 3% of a mixture of guar gum and cellulose (Wu et al., Reference Wu, Xiong, Zhong, Li, Wan, Wu and Liu2020). Mechanisms for the observed effects could be related to higher short-chain fatty acid (SCFA) production in the gut, enhanced serotonin secretion, and a T helper 2 lymphocyte (Th2)-based immune response in gestating sows with a positive impact on embryo survival. Higher birth weights were also reported when sows were fed with diets with 40% SBP during gestation, which might be related to more favorable microbial activity, as discussed by Rooney et al. (Reference Rooney, O'Driscoll, O'Doherty and Lawlor2019). Ground wheat straw (13%) in gestation diets for sows increased litter size and total litter weight at birth, which might be related to a higher feed intake (Veum et al., Reference Veum, Crenshaw, Crenshaw, Cromwell, Easter, Ewan, Nelssen, Miller, Pettigrew and Ellersieck2009). However, ad libitum feeding of a gestation diet with 23% WB, 20% SBP, and 14% oat bran did not affect the reproductive performance of sows (Peltoniemi et al., Reference Peltoniemi, Tast, Heinonen, Oravainen, Munsterhjelm, Halli, Oliviero, Hameenoja and Virolainen2010). High inclusion rates of WB at 24 and 42% in gestation diets had no enhancing effect on litter size, piglet viability, and body weight gain (BWG) in primiparous sows, but resulted in increased litter performance in the next parity (Che et al., Reference Che, Feng, Wu, Fang, Lin and Yan2011). The inclusion of 8% SBP and 3% cereal straw during gestation and lactation had no impact on reproductive performance (Alvarez-Rodriguez et al., Reference Alvarez-Rodriguez, Mir, Seradj, Morazan, Balcells and Babot2017). Similarly, no impact on performance was observed when breeding sows were fed with a mixture of SBP, alfalfa, soybean hulls, grape pomace, and lignocellulose during gestation (Priester et al., Reference Priester, Visscher, Fels, Rohn and Dusel2020).
Table 1. Effects of DF sources in feed on pregnant and lactating gilts and sows

Table 2. Effects of DF sources in feed on suckling piglets
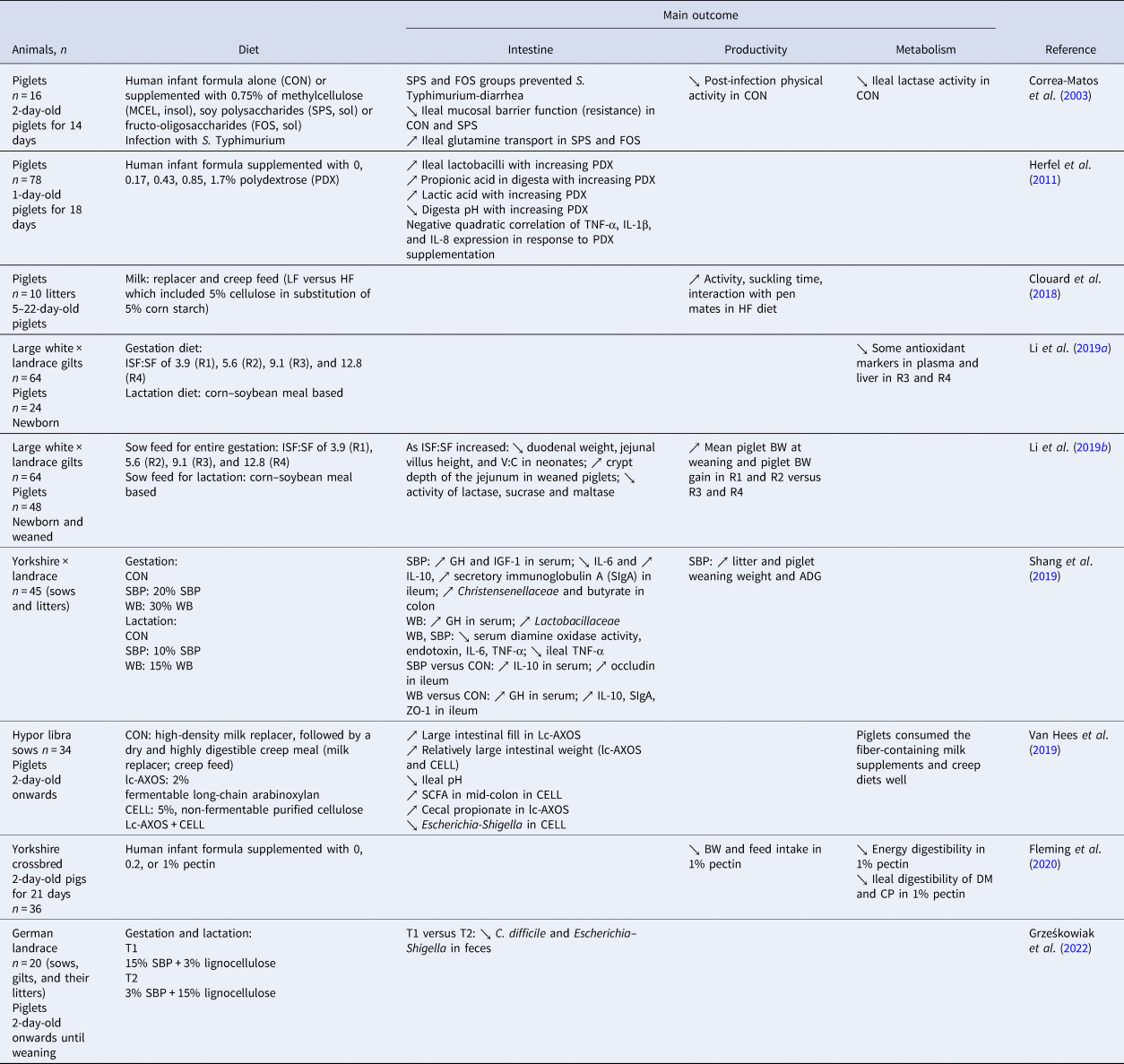
DF, nutrient digestibility, and metabolic effects on sows
Feed intake and nutrient digestibility in sows can be influenced by high-fiber (HF) diets. Specifically, serum levels of vitamin B12 and minerals were lower when sows received diets enriched in fibers such as 53% corn cobs, 43% WB, or 53% oat hulls. The observed effects could be related to the modulation of cecal and colonic vitamin B12-producing microbiota and the mineral-binding capacity of fiber and reduced time for mineral absorption due to increased transit time (Girard et al., Reference Girard, Robert, Matte, Farmer and Martineau1995). A further study showed that diets with 15% SBP resulted in an improved overall N-retention of breeding sows, which could be explained by increased uptake of N from urea and other N-sources by the gut microbiota and therefore a lower N secretion in urine (Patráš et al., Reference Patráš, Nitrayová, Brestenský and Heger2012).
DF often showed a negative impact on total tract organic matter (OM), nutrients, and energy digestibility, but differences were observed depending on fiber sources. SBP and alfalfa meal (12 or 17%) reduced apparent total tract digestibility of energy, crude protein (CP), and in the case of alfalfa meal, non-starch polysaccharides (NSP) (Krogh et al., Reference Krogh, Bruun, Amdi, Flummer, Poulsen and Theil2015). In sows, total tract digestibility of energy, dry matter (DM), OM, CP, and ether extract were moderately reduced by the inclusion of 15% SBP and more distinct with diets containing 23% maize bran and 27% WB (Le Goff et al., Reference Le Goff, Le Groumellec, van Milgen, Dubois and Noblet2002). Diets rich in DF, specifically 9.8% sunflower meal, 9.8% WB, 19.5% SBP, and 9.8% soybean hulls reduced the circulating concentration of leptin and improved the feed intake of sows before farrowing (Quesnel et al., Reference Quesnel, Meunier-Salaun, Hamard, Guillemet, Etienne, Farmer, Dourmad and Pere2009). Either 10% native or heat and pressure processed wheat or oat straw in late gestation diets lowered energy and DM digestibility in gestating sows, especially when included in the native form. Preprandial prolactin concentration was higher with oat straw, whereas postprandial insulin-like growth factor-1 (IGF-1) and prolactin were increased with processed wheat straw. Hence, oat straw was considered more beneficial compared to wheat straw, but underlying mechanisms could not be further elucidated (Agyekum et al., Reference Agyekum, Columbus, Farmer and Beaulieu2019). Surprisingly, one study showed higher postprandial insulin levels in sows fed with HF diets containing each 8% soybean hulls, WB, sunflower meal, and SBP, whereas prolactin was not affected (Loisel et al., Reference Loisel, Farmer, Ramaekers and Quesnel2013).
A recent study in gilts receiving an HF diet based on corn bran (30% of inclusion) also showed a decrease in DM, CP, gross energy, and fiber digestibility accompanied by increased digesta viscosity and reduced cecal fermentation. The observed effects were due to the high amount of neutral-detergent fiber (NDF) in the diets resulting in decreased nutrient and energy digestibility, reduction in relative hindgut fermentation, impairment of pig performance and dilution of dietary energy, as explained by Petry et al. (Reference Petry, Huntley, Bedford and Patience2021).
Effects of DF on reproductive physiology of sows
Colostrum is the first source of energy, nutrients, and bioactive compounds for newborn piglets, being replaced in this role by milk, shortly after farrowing. Therefore, modulation of colostrum, as well as milk yield and composition in lactating sows may offer an opportunity to positively influence the development and health of offspring. A diet with a mixture of soybean hulls, WB, sunflower meal, and SBP, including 8% of each fiber source at late pregnancy (from day 106 until parturition), had no effect on colostrum yield and hormones involved in lactogenesis, but it increased colostrum lipids and decreased immunoglobulin A (IgA) concentration in colostrum and milk compared to a control diet (Loisel et al., Reference Loisel, Farmer, Ramaekers and Quesnel2013). A more recent study compared a diet without added fiber to gestation diets with 20% SBP or 30% WB and lactation diets with 10% SBP or 15% WB as fiber sources. Increases in litter weight at weaning and higher feed intake, IgA and interleukin-10 (IL-10) levels in colostrum and IgA in milk were observed when sows were fed with SBP, whereas IL-10 levels in milk were increased in sows fed with WB when compared to the control group. A higher feed intake was interpreted as related to increased insulin sensitivity in sows fed with SBP (Shang et al., Reference Shang, Liu, Liu, He and Piao2019). The use of HF gestation diets can have an impact on sow performance that would last during lactation, even when using standard lactation diets. Thus, some parameters such as feed intake, body weight loss, or backfat loss during lactation could be ameliorated in sows that receive HF gestation diets (van der Peet-Schwering et al., Reference van der Peet-Schwering, Kemp, Binnendijk, den Hartog, Spoolder and Verstegen2003; Quesnel et al., Reference Quesnel, Meunier-Salaun, Hamard, Guillemet, Etienne, Farmer, Dourmad and Pere2009; Krogh et al., Reference Krogh, Bruun, Amdi, Flummer, Poulsen and Theil2015). However, these effects might depend on the composition of the fiber (Renteria-Flores et al., Reference Renteria-Flores, Johnston, Shurson, Moser and Webel2008; Veum et al., Reference Veum, Crenshaw, Crenshaw, Cromwell, Easter, Ewan, Nelssen, Miller, Pettigrew and Ellersieck2009; Agyekum et al., Reference Agyekum, Columbus, Farmer and Beaulieu2019). Another study observed that diets with 9.8% WB, 19.5% SBP, and 9.8% soybean hull provided to pregnant sows (from day 26 until parturition) could not protect sows from body weight (BW) loss, despite increased average daily feed intake (ADFI) during lactation (Quesnel et al., Reference Quesnel, Meunier-Salaun, Hamard, Guillemet, Etienne, Farmer, Dourmad and Pere2009). When prepubescent gilts were fed a diet with a daily intake of 60 g inulin and 240 g cellulose, serotonin and melatonin concentrations in serum and follicular fluid were increased and follicular atresia reduced (Zhuo et al., Reference Zhuo, Cao, Gong, Tang, Jiang, Li, Yang, Xu, Li, Che, Lin, Feng, Fang and Wu2021). The effects were explained by a dietary impact on the intestinal microbial serotonin synthesis.
DF affects the intestinal microbiota, gut physiology, and immune reactions in sows
The intestinal microecosystem of a sow can be shaped by DF in different ways. Here, the ratio of SF to ISF can be a decisive variable. Sows fed with either SF or ISF demonstrated different fecal profiles of SCFA, and this observation was also noted in the colon digesta of their offspring (Li et al., Reference Li, Liu, Zhang, Yang, Lin, Zhuo, Fang, Che, Feng, Xu, Li and Wu2019a). Gestating sows fed diets with ISF:SF ratios of 3.89, 5.59, 9.12, and 12.81 produced piglets with the mean BW at weaning and BWG characterized by a linear decrease as the ISF:SF ratio increased. In addition, the crypt depth of the jejunum in weaned piglets linearly increased, whereas the duodenal weight, jejunal villus height, and villus height/crypt depth in newborn piglets and enzymatic activity of lactase, sucrase, and maltase linearly decreased. The observations were explained as the ISF:SF impact on development and enzymatic activity in the small intestine that enhanced piglet BW gain (Li et al., Reference Li, Zhang, Liu, Yang, He, Cao, Yang, Zhong, Lin, Zhuo, Fang, Che, Feng, Xu, Li, Zhao, Jiang and Wu2019b). An increase in leukocyte count was observed in sows fed diets with 25% SBP (McGlone and Fullwood, Reference McGlone and Fullwood2001). Differences in gut microbial community were associated with different ISF:SF ratios of 3.89 and 5.59 versus 9.12 and 12.81 in the sows' diets. Additionally, the intake of more SF also led to higher antioxidant enzyme activity, suppression of pro-inflammatory factors in sows and their piglets, a higher hepatic expression of Nrf2 and HO-1 and lower expression of nuclear factor-κB (NF-κB) in piglets (Li et al., Reference Li, Liu, Zhang, Yang, Lin, Zhuo, Fang, Che, Feng, Xu, Li and Wu2019a). Fecal butyrate and propionate concentrations, as well as bacteria belonging to Roseburia and Eubacterium-hallii group, and Bacteroides, were elevated in gestating sows fed with diets with 1.5% guar gum and 1.5% cellulose (Wu et al., Reference Wu, Xiong, Zhong, Li, Wan, Wu and Liu2020). Sows fed with diets enriched with 15% WB and 10% SBP during gestation and 7.5% WB and 5% SBP in lactation showed an enhanced intestinal barrier and a reduction of Subdoligranulum and Mogibacterium, whereas Lactobacillus counts increased in the colon (Shang et al., Reference Shang, Liu, Liu, Mahfuz and Piao2021). Diets rich in oil reduced the SCFA-producing microbes, a phenomenon which was reversed when fiber (300 g inulin and cellulose per day) was added to the feed (Zhuo et al., Reference Zhuo, Cao, Gong, Tang, Jiang, Li, Yang, Xu, Li, Che, Lin, Feng, Fang and Wu2021). A very recent study demonstrated that sows fed diets enriched with highly fermentable DF, such as SBP (15%) compared to less fermentable lignocellulose (15%), had increased fecal SCFA, Muribaculaceae and microbial Shannon diversity index but decreased Terrisporobacter spp. during the periparturient period (Grześkowiak et al., Reference Grześkowiak, Saliu, Martínez-Vallespín, Wessels, Männer, Vahjen and Zentek2022).
DF and welfare of sows
Maintaining sows in groups has a positive impact on their social behavior (Verdon et al., Reference Verdon, Hansen, Rault, Jongman, Hansen, Plush and Hemsworth2015). However, some individuals also tend to show aggressive attitudes with consequences on performance, welfare, and health. The inclusion of DF in diets has been reported to affect behavior in pigs. A tendency to less aggressive interactions and fewer fights was noted in sows fed diets enriched in different DF sources such as a mixture of grape pomace, lignocellulose, and soybean hulls (Priester et al., Reference Priester, Visscher, Fels, Rohn and Dusel2020). Soybean hulls (19%) or resistant starch (11%) had a similar effect. Higher satiety by more constant blood glucose and non-esterified fatty acids with higher gut fill (satiety) could explain the observed effects (Sapkota et al., Reference Sapkota, Marchant-Forde, Richert and Lay2016). Sham-chewing is considered a behavior related to stress in sows (Tatemoto et al., Reference Tatemoto, Bernardino, Alves and Zanella2019). Interestingly, sows kept outdoor had increased sham-chewing when fed a diet with 25% SBP, which was not observed in sows kept indoors (McGlone and Fullwood, Reference McGlone and Fullwood2001). A reduction of stereotypical behavior was observed when diets contained high amounts of DF levels from alfalfa meal (>27%) and oat hulls (27–45%) (Bergeron et al., Reference Bergeron, Bolduc, Ramonet, Meunier-Salaun and Robert2000). On the contrary, an HF diet (9.8% sunflower meal, 9.8% WB, 19.5% SBP) fed to pregnant gilts had limited effects on farrowing behavior and reproductive performance; however, piglets reared by sows which received the HF gestation diets showed a 13.5% higher growth rate during the first week of life (Guillemet et al., Reference Guillemet, Hamard, Quesnel, Pere, Etienne, Dourmad and Meunier-Salaun2007). When gestation diets included 35% of soybean hulls, piglets showed fewer skin lesions compared to piglets from sows fed with a low-fiber diet, indicating less aggressive interactions in the litters (Bernardino et al., Reference Bernardino, Tatemoto, Morrone, Mazza Rodrigues and Zanella2016).
In summary, both, gestation and lactation periods seem to be promising for dietary interventions in sows to also influence the fitness of their offspring. By altering the sow's physiology, colostrum composition, and intestinal microbial ecology and function through nutritional factors, it may be possible to influence the piglet's microbial development and health. However, still too little is known about the impact of diet on the microbial association between sow and offspring and the establishment of the gut microbiota in neonatal piglets. Moreover, a growing body of evidence suggests a beneficial impact of DF on sows' behavior related to stress.
DF in suckling piglets
The immune system of piglets needs to acquire passive immunity through colostrum and milk (Stokes, Reference Stokes2017). Thus, feeding gestating and/or lactating sows with certain DF offers an attractive way to beneficially modulate colostrum composition, and the sow's fecal microbiota and metabolites, which in turn can have a positive impact on the health of piglets.
An overview of studies on the impact of DF offered as a creep feed or supplemented formula on suckling piglets is provided in Table 2. To increase productivity in sows, a decisive breeding goal in piglet production is to enlarge litter sizes. This has an impact on the health and welfare of sows and piglets (Rutherford et al., Reference Rutherford, Baxter, D'Eath, Turner, Arnott, Roehe, Ask, Sandoe, Moustsen, Thorup, Edwards, Berg and Lawrence2013). The extremely high litter size in modern sow breeds implies difficulties in raising all the piglets due to the limitation of functional teats and low individual birth weights, which may result in undernourished or deceased piglets (Kobek-Kjeldager et al., Reference Kobek-Kjeldager, Moustsen, Theil and Pedersen2019). Additional milk replacers in suckling piglets offer an opportunity for higher survival of piglets in large litters and an early introduction of solid feed to the piglets' diets (Van Hees et al., Reference Van Hees, Davids, Maes, Millet, Possemiers, den Hartog, van Kempen and Janssens2019). Data on the physiological impact of DF on suckling piglets are sparse. It has been demonstrated that piglets consuming human infant formula supplemented with 1% pectin had a reduced ileal digestibility of DM and CP, lower feed intake, and BWG, as well as a decrease in energy digestibility (Fleming et al., Reference Fleming, Richards, Bradley, Pan, Li and Dilger2020). Still, suckling piglets can benefit from DF intake due to its potential to guide the developing gut microbiota in a process known as ‘early microbial programming’ and DF may therefore have an impact on intestinal function and health in growing animals (Li et al., Reference Li, Liu, Zhang, Yang, Lin, Zhuo, Fang, Che, Feng, Xu, Li and Wu2019a, Reference Li, Zhang, Liu, Yang, He, Cao, Yang, Zhong, Lin, Zhuo, Fang, Che, Feng, Xu, Li, Zhao, Jiang and Wu2019b). Hence, in very young piglets, DF supply may be beneficial for the early immune programming and thus, together with microbial programming, it is of particular importance for building up a better resilience against pathogens. It may contribute to the prevention of common health problems such as gut dysbiosis, leading to neonatal mortality and especially post-weaning diarrhea (PWD) (Lindberg, Reference Lindberg2014).
It has been demonstrated that fiber-supplemented liquid feed and milk replacers for suckling piglets could improve intestinal microecology and gut epithelial health. For instance, increasing concentrations of polydextrose (0, 0.17, 0.43, 0.85, 1.7%) in infant formula, increased numbers of lactobacilli, propionic, and lactic acid linearly whereas pH and pro-inflammatory cytokine patterns decreased in the ileum digesta (Herfel et al., Reference Herfel, Jacobi, Lin, Fellner, Walker, Jouni and Odle2011). Moreover, certain DFs have been demonstrated to reduce enteric infections in suckling piglets. Administration of 0.75% soy polysaccharides or fructo-oligosaccharides in infant formula prevented Salmonella Typhimurium-related diarrhea and improved gut function, probably by means of increased glutamine and total ion transport as assessed in the small intestine of suckling piglets (Correa-Matos et al., Reference Correa-Matos, Donovan, Isaacson, Gaskins, White and Tappenden2003). DF has also been demonstrated to influence the behavior of suckling piglets. Offering a supplementary diet enriched in 5% cellulose to suckling piglets from 5 to 22 days of age, resulting in higher piglet activity, increased suckling time and interactions with pen mates, as compared to control piglets (Clouard et al., Reference Clouard, Stokvis, Bolhuis and van Hees2018).
Administration of DF in a creep feed to suckling piglets may be challenging due to exposure to a high load of new antigens in the developing intestine; however, along with ingestion of sow milk, it may offer an opportunity to influence the gut microecology and health directly at an early stage of life. A recent study demonstrated an increase in SCFA in the hindgut and a reduction of the Escherichia–Shigella group in the colon of 3-week-old suckling piglets that were offered a creep feed supplemented with 5% cellulose from the second day onwards after birth (Van Hees et al., Reference Van Hees, Davids, Maes, Millet, Possemiers, den Hartog, van Kempen and Janssens2019). Interestingly, piglets were reported to consume the creep diets well. Therefore, fiber-enriched creep feeds, if consumed at an early age, might be an attractive way to directly support the gut health of suckling piglets.
Nursing piglets have constant contact with sow feces after birth, which may facilitate the microbiota colonization process (Mach et al., Reference Mach, Berri, Estelle, Levenez, Lemonnier, Denis, Leplat, Chevaleyre, Billon, Doré, Rogel-Gaillard and Lepage2015). Hence, the sow diet may indirectly shape the microecosystem in their offspring's gastrointestinal tract and thereby influence piglet performance, immune status, and other attributes (Table 1). For instance, feeding the sows with diets supplemented with Jerusalem artichoke, with an estimated daily intake of 1.3 kg DM, had increased IgG serum levels in their offspring (Werner et al., Reference Werner, Schubbert, Schrodl, Kruger and Sundrum2014). The addition of 3% inulin to the sows' diets during gestation and lactation resulted in increased levels of enterococci, eubacteria, and the Clostridium leptum group in the gut contents of suckling piglets (Paßlack et al., Reference Paßlack, Vahjen and Zentek2015). Maternal supplementation with a combination of SBP and WB during late gestation (15% WB and 10% SBP) and lactation (7.5% WB and 5% SBP) improved growth, immune responses, intestinal morphology, barrier function, and microbiota in piglets, as compared to control diet (corn–soybean meal). Moreover, immunoglobulins and cytokines in colostrum and milk were also increased in sows fed with SBP–WB, which may have accounted for the observed beneficial effects in piglets (Shang et al., Reference Shang, Liu, Liu, Mahfuz and Piao2021). In another study on maternal dietary effects on the offspring, sows fed diets enriched with SBP (15%), as compared to lignocellulose (15%), had reduced Clostridioides difficile concentrations and Escherichia–Shigella abundance in feces of their suckling piglets (Grześkowiak et al., Reference Grześkowiak, Saliu, Martínez-Vallespín, Wessels, Männer, Vahjen and Zentek2022).
In summary, feed supplementation with DF is a feeding strategy with the potential to control the metabolic and immune patterns and microbial colonization in sows and offspring. By feeding DF to sows, piglets could benefit from the sow–piglet association phenomenon and early microbial and immune programming. Thereby DF could offer a promising way to protect against gut pathogen dissemination in suckling piglets.
DF in weaner pigs
In several studies on specific physiological effects of DF sources in diets for weaner pigs, digestive function, intestinal microbiota, immune reactions, and occurrence of PWD were investigated (Table 3).
Table 3. Effects of DF sources in feed on weaner pigs

DF and gastrointestinal function in weaner pigs
DF influences gastrointestinal function and performance by different mechanisms. DF is often considered an anti-nutritional factor by reducing CP, amino acid (AA), and fat digestibility due to reduced enzymatic hydrolyzation, absorption, and/or increased endogenous secretion (Blank et al., Reference Blank, Schlecht and Susenbeth2012). NDF increments are seen to result in a reduction of ileal apparent CP digestibility and reduction of the digestibility coefficient of energy in pigs (Dégen et al., Reference Dégen, Halas and Babinszky2007). However, dietary inclusion of 5–15% DM of partly HCl-hydrolyzed straw meal did not lead to a reduction of BWG or feed intake (Münchow et al., Reference Münchow, Hasselmann and Finger1988). The reduction of digestibility can be more severe with the addition of SF as compared to ISF fiber types. In general, SF can increase the viscosity of the digesta and thus, might slow down gut transit time in the small intestine (Bach Knudsen et al., Reference Bach Knudsen, Jensen, Andersen and Hansen1991) due to suppressed intestinal contractions (Cherbut et al., Reference Cherbut, Albina, Champ, Doublier and Lecannu1990), which in turn leads to reduced efficacy of digestive enzymes. In contrast, ISF may stimulate pancreatic protease enzyme activity (Langlois et al., Reference Langlois, Corring and Fevrier1987). Thus, DF may reduce nutrient digestibility due to nutrient dilution, reduced nutrient absorption, reduced enzymatic breakdown, and/or increased endogenous excretion in pigs, depending on fiber type (Lenis et al., Reference Lenis, Bikker, van der Meulen, van Diepen, Bakker and Jongbloed1996; Agyekum and Nyachoti, Reference Agyekum and Nyachoti2017). Chicory roots and ribwort at increasing concentrations of 4–16% had only a minor effect on the total apparent nutrient digestibility in piglets. Although the concentrations of coliform bacteria changed with age, there was no evidence that the DF supplements led to clear functional effects on the gastrointestinal tract (Ivarsson et al., Reference Ivarsson, Frankow-Lindberg, Andersson and Lindberg2011). Oat husks are a typical non-fermentable and lignin-rich fiber source. At a 2% inclusion rate, the performance of weaner pigs was not influenced, although the apparent total tract digestibility of energy and DM was reduced (Kim et al., Reference Kim, Mullan, Hampson and Pluske2008).
DF and intestinal microbiota in weaner pigs
Moderate and varying effects of DF were demonstrated when either 5% corn cobs, 2% chicory roots, 12% SBP, or 7.5% WB was added to a diet for weaner pigs. Specifically, corn cobs favored lactobacilli and bifidobacteria in the stomach and proximal duodenum digesta, whereas WB increased Escherichia coli in the jejunum digesta and mucosa and increased levels of lactobacilli and bifidobacteria in the gastric and jejunal digesta. All in all, the changes in intestinal microbiota were moderate (van Nevel et al., Reference Van Nevel, Dierick, Decuypere and De Smet2006). WB was investigated in various studies in weaner piglets. At an inclusion level of 4%, it had a stimulating effect on the formation of SCFA, at the same time reducing E. coli counts in the digestive tract (Molist et al., Reference Molist, Hermes, de Segura, Martin-Orue, Gasa, Manzanilla and Perez2011). The use of native, fermented, or extruded WB at 15% did not affect performance in piglets. Nevertheless, there were effects on the number of goblet cells in the ileum, which was higher when native and extruded WB were used compared to fermented WB. Intestinal counts of E. coli were lower in control piglets than in the groups with added WB. The modified WB variants resulted in lower concentrations of SCFA in the colon compared to unprocessed WB. Furthermore, a reduction of lipid radicals was also demonstrated when using the native WB (Kraler et al., Reference Kraler, Schedle, Schwarz, Domig, Pichler, Oppeneder, Wetscherek, Pruckler, Pignitter, Pirker, Somoza, Heine and Kneifel2015). At a level of 10%, WB also increased fecal bifidobacteria (Yu et al., Reference Yu, Zhang, Yang, Peng, Zhu, Zeng and Qiao2016). A 4% coarsely ground WB administered to piglets increased SCFA, reduced Bacteroidetes, and increased Firmicutes (Molist et al., Reference Molist, Manzanilla, Perez and Nyachoti2012). When using 5% grape pomace, increasing concentrations of Lactobacillus delbrueckii, Olsenella umbonata, and Selenomonas bovis were found in the cecum of young piglets (Wang et al., Reference Wang, Yu, Fang, Jin, Zhao, Shen, Zhou, Li, Wang, Fu and Zhang2020).
DF and immune system of weaner pigs
Reduced levels of intraepithelial lymphocytes in the jejunum were detected in weaner pigs fed 2% chicory roots or 5% corn cobs, whereas an increase was observed when fed with 12% SBP or 7.5% WB; however, the functional significance was not reported (van Nevel et al., Reference Van Nevel, Dierick, Decuypere and De Smet2006). The inclusion of 10% WB and pea fiber resulted in increased diamine oxidase activity and expression of transforming growth factor-alpha (TGF-α), trefoil factors and major histocompatibility complex (MHC)-II in the ileum tissues (Chen et al., Reference Chen, Mao, He, Yu, Huang, Yu, Zheng and Chen2013).
The use of 1.5% lignocellulose reduced the cadaverine concentrations in the digesta, but no effects on the expression of immune-relevant genes were found in ileum, spleen, liver, or mesenteric lymph node tissues (Slama et al., Reference Slama, Schedle, Wetscherek, Pekar, Schwarz and Gierus2020). In another study using 5% grape pomace, the expression of some cytokines in the intestinal tissue was down-regulated (IL-1β, IL-8, IL-6, and tumor necrosis factor-alpha (TNF-α)). Additionally, the levels of IgG were increased in the serum. In that study, however, effects on the occurrence of diarrhea were not observed (Wang et al., Reference Wang, Yu, Fang, Jin, Zhao, Shen, Zhou, Li, Wang, Fu and Zhang2020).
DF and prevention of diarrhea in weaner pigs
PWD is a common problem in piglets. Oat hulls (2%) were effective when animal protein-based diets were supplemented with rice, whereas supplementation of animal protein-based diets with wheat did not protect against diarrhea. This indicates a fiber–matrix interaction and it was explained by differences in carbohydrate and proteinaceous materials entering the colon (Kim et al., Reference Kim, Mullan, Hampson and Pluske2008). Importantly, the particle size of DF might be relevant for the preventive effect on PWD. When 4% coarsely ground WB was administered to piglets, significantly firmer fecal consistency was observed after a challenge with E. coli K88+, whereas finely ground WB did not have a similar protective effect. Besides a higher binding of E. coli to the coarser fiber, a higher water-binding capacity of the intestinal digesta was discussed as a possible cause (Molist et al., Reference Molist, Manzanilla, Perez and Nyachoti2012).
The abovementioned studies demonstrate the importance of evaluating chemical and physical characteristics when examining the effects of DF, a fact that has been neglected in most studies. Positive effects on the incidence of diarrhea in weaner piglets were also shown when using WB in a dosage of 10%, which could be associated with multiple changes in the intestinal morphology, microbiota composition, increased zonula occludens-1, and occludin mRNA and higher diamine oxidase activities, TGF-α, trefoil factor family, and MHC-II levels in the ileum and colon tissues (Chen et al., Reference Chen, Mao, He, Yu, Huang, Yu, Zheng and Chen2013). Contrarily, no protective effects on the occurrence of diarrhea after weaning could be observed when 5–10% WB, soybean hulls, or oat husks were included in the diets of weaner pigs (Yu et al., Reference Yu, Zhang, Yang, Peng, Zhu, Zeng and Qiao2016).
Lignocellulose is a mixture of cellulose, hemicelluloses, and lignin. When included in finely ground form at 1% in the diet, a measurable effect was noted on the occurrence of PWD. At the same time, the lignocellulose-treated group showed changes in immune parameters and acute phase proteins (Superchi et al., Reference Superchi, Saleri, Borghetti, Ferrarini, Cavalli, Sereni, Zavattini and Sabbioni2017). The inclusion of 2.5% soybean hulls or 1.5% lignocellulose in a complete diet did not evoke negative effects on the performance and feed expenditure and fecal DM (Slama et al., Reference Slama, Schedle, Wetscherek, Pekar, Schwarz and Gierus2020).
Inulin, as a highly fermentable fiber, induced a positive effect on intestinal physiology in piglets after weaning. Specifically, the inclusion of 3% of dietary inulin in feed for gestating and lactating sows and their offspring thereafter increased glucose transport and altered intestinal barrier function in post-weaned piglets (Awad et al., Reference Awad, Ghareeb, Paßlack and Zentek2013). Similarly, a diet with 8% WB lowered epithelial resistance in the cecum of piglets (Stumpff et al., Reference Stumpff, Lodemann, Van Kessel, Pieper, Klingspor, Wolf, Martens, Zentek and Aschenbach2013).
In summary, the use of fiber-rich components in the diet of weaner piglets can affect nutrient digestibility, performance, and health. The effects described depend on fiber sources, particularly with regard to their fermentability, on the matrix into which the fiber-rich feed is mixed, and on physical properties. The latter is demonstrated by the importance of particle length (Molist et al., Reference Molist, Manzanilla, Perez and Nyachoti2012). Moderately fermentable fiber sources with larger particle sizes appear to have beneficial effects on the intestinal health of piglets after weaning.
DF in fattening pigs
Adaptive potential to fiber-enriched diets in fattening pigs
The use of fiber-rich components is increasingly discussed, especially with regard to the application of more fibrous by-products in the nutrition of pigs (Table 4). Straw meal was considered early as an interesting alternative in the feeding of pigs. First investigations were performed with 5–10% hydrolyzed straw meal in fattening pigs, indicating positive dietetic effects (Bergner and Betzin, Reference Bergner and Betzin1979) but higher intestinal nitrogen (N) losses (Simon et al., Reference Simon, Bergner and Partridge1987). When using a diet with 10% of wheat straw, no negative effects on feed intake and performance of fattening pigs could be observed. However, in the jejunum and colon, there was clear evidence of increased cell proliferation (Jin et al., Reference Jin, Reynolds, Redmer, Caton and Crenshaw1994). Pigs can obviously adapt to HF diets by an increase of the cellulolytic capacity of the intestinal microbiota. Very high inclusion rates of 50% alfalfa meal in diets for pigs induced an initial suppression of the intestinal microbial activity followed by an adaptive increase (Varel et al., Reference Varel, Pond, Pekas and Yen1982). The numbers of cellulose-degrading bacteria in the intestine were higher and increased microbial cellulase activity was observed within 3 days (Varel et al., Reference Varel, Pond and Yen1984, Reference Varel, Robinson and Jung1987). Increased weight of the visceral organs and cellulolytic intestinal bacteria was also observed in barrows weighing 55 kg (Anugwa et al., Reference Anugwa, Varel, Dickson, Pond and Krook1989). Extremely high quantities (80%) of alfalfa meal resulted in a reduced BWG and higher weights of the gastrointestinal tract, but also of the liver and kidney (Pond et al., Reference Pond, Jung and Varel1988). Digestibility of feed was reduced by the use of correspondingly high amounts of alfalfa despite a microbial adaptation in the sense of an increase in cellulolytic bacteria was observed (Varel et al., Reference Varel, Jung and Pond1988). Increasing dietary NSP content up to 19.5% led to a decrease in nutrient digestibility although it was dependent on the solubility of the NSP (Högberg and Lindberg, Reference Högberg and Lindberg2004). Digestibility of protein was reduced in growing pigs when diets contained 21% wheat aleurone fiber; 37% rye aleurone fiber reduced also the apparent digestibility of fat and starch. Rye products were more likely to be fermented in the cecum and wheat products in both the cecum and the colon (Le Gall et al., Reference Le Gall, Serena, Jorgensen, Theil and Bach Knudsen2009).
Table 4. Effects of DF sources in feed on fattening pigs

DF sources and intestinal effects on fattening pigs
The use of peas and lupins, which were either peeled or unpeeled, did not affect the true digestibility of N and AAs in pigs, indicating that the inclusion of 3–7% of crude fiber (CF) does not cause a depression in the true digestibility of N and AAs (Meier et al., Reference Meier, Kesting and Poppe1981). A reduction in the digestibility of various nutrients, which was due to a reduced intestinal transit time and an increase in fecal N excretion, was observed when 50% of ground oats was included at the expense of corn in a diet for pigs at 35 kg BW (Ravindran et al., Reference Ravindran, Kornegay and Webb1984). Besides digestibility, the effects of different fiber sources on the intestinal microbiota and microbial fermentation, especially the formation of SCFA, were characterized. Grass meal and SBP with levels of 13.5–17% CF for fattening pigs gave clear indications that microbial fermentation was significantly increased in the first third of the porcine colon (Schnabel et al., Reference Schnabel, Schneider and Bolduan1990). Oat bran (15%) increased butyrate formation in the intestinal tract of pigs, especially in combination with rolled oats (Bach Knudsen et al., Reference Bach Knudsen, Jensen, Andersen and Hansen1991). The use of soybean hulls and wheat middling in dosages of 30% each led to a significant reduction in the net energy of the feed and performance of growing pigs, which was higher in pigs at 25.4 kg BW compared to finishers at 84.8 kg BW (Stewart et al., Reference Stewart, Kil, Ji, Hinson, Beaulieu, Allee, Patience, Pettigrew and Stein2013).
So far, little research has been devoted to the dependency of endogenous N losses on fiber intake and the association of true CP and AA ileal digestibility. The published studies on pigs of different production stages focused on the availability of threonine with 15% soybean hulls (Mathai et al., Reference Mathai, Htoo, Thomson, Touchette and Stein2016), 0, 4, 8, or 12% pectin, or 8% cellulose (Zhu et al., Reference Zhu, Rademacher and de Lange2005). As an outcome, pigs had a higher requirement for dietary threonine to maintain growth when the DF was increased. Among the essential AAs, the metabolism and the utilization of threonine are likely most severely influenced by DF effects on intestinal endogenous secretion and microbial activity (de Lange et al., Reference de Lange, Sauer, Mosenthin and Souffrant1989; Lien et al., Reference Lien, Sauer and Fenton1997). The utilization of other AAs that are present in endogenous secretions, such as cysteine and branched-chained AAs, may be influenced as well (Zhu et al., Reference Zhu, Rademacher and de Lange2005). The use of alfalfa flour, SBP, or WB in doses of 44, 60, and 41%, respectively, led to a depressive effect on the standardized ileal digestibility of AA in pigs. WB had a more pronounced effect than the other two products inclusion (Eklund et al., Reference Eklund, Rademacher, Sauer, Blank and Mosenthin2014).
When using WB at 3% or pine pollen at 1.3 or 2.6%, positive effects on villus height in jejunum and ileum were observed in piglets with an average BW of 8.3 kg. Furthermore, there was some evidence that WB influences the expression of NF-κB in the stomach and jejunum. Also, there were effects on the expression of TNF-α, TGF-β, caspase 3, CDK4, and IGF-1 in the colon. In the mesenteric lymph nodes, the expression of NF-κB and TGF-β was upregulated (Schedle et al., Reference Schedle, Pfaffl, Plitzner, Meyer and Windisch2008). The use of 37% WB, 28% SBP, or 41% dried distillers' grains with solubles (DDGS) from maize products in pigs resulted in a reduction in the digestibility of nutrients, while diets with 33% inner pea fiber or 18% pea hulls led to improved N utilization. Pea fiber was able to stimulate the formation of SCFA in the ileum and colon. However, the concentration of intestinal ammonia also increased (Jha and Leterme, Reference Jha and Leterme2012). Supplementation of wheat-barley or corn/wheat gluten-based diets with 3% inulin fed to 6-week-old pigs for 3 or 6 weeks did not affect growth but increased the prevalence of pigs with bifidobacteria in the colon (40 versus 13%) and decreased acetate and total SCFA concentrations while butyrate proportion was higher. Interestingly, 20–50% of the ingested inulin was already degraded in the jejunum (Loh et al., Reference Loh, Eberhard, Brunner, Hennig, Kuhla, Kleessen and Metges2006). Feeding an HF diet with 37 or 23% SBP even reduced ammonia emissions from gestating sows and fattening pigs under barn conditions by 25–50%; however, it increased CH4 emissions by 30–50% (Philippe et al., Reference Philippe, Laitat, Wavreille, Nicks and Cabaraux2015). When using WB as a component in a diet with 4 or 24% of crude fat with sunflower margarine and sweet cream butter as a model for a ‘westernized diet’ for growing pigs, the weight of the digestive organs increased, and some changes in the microbiota were noted. Despite lower overall levels of bacteria in the cecum digesta, there was an increase in SCFA concentration in both the colon and cecum (Heinritz et al., Reference Heinritz, Weiss, Eklund, Aumiller, Heyer, Messner, Rings, Louis, Bischoff and Mosenthin2016a, Reference Heinritz, Weiss, Eklund, Aumiller, Louis, Rings, Messner, Camarinha-Silva, Seifert, Bischoff and Mosenthin2016b). Dietary carbohydrate composition may have potential in preventing intestinal disorders in certain periods of the growing phase, as could be deduced by the changes in the microbiota and coliform diversity, mainly at the ileocecal ostium when increasing NSP levels up to 19.5% (Högberg et al., Reference Högberg, Lindberg, Leser and Wallgren2004). Long-chain inulin (2%) and Jerusalem artichoke (4%) improved the performance and health-related measurements in growing pigs (Samolińska et al., Reference Samolińska, Kowalczuk-Vasilev and Grela2018); however, 0.5% inulin failed to induce a positive impact on animal performance in another study (Wang et al., Reference Wang, Chen, Yu, Huang, Luo, Zheng, Mao, Yu, Luo and He2019). The inclusion of 30% DDGS in a corn–soybean meal increased dietary NDF from 8.6 to 12.8%. Feeding the diet until 28, 21, 14, or 0 days prior to slaughter did not affect feed intake and feed conversion nor the final BW (Lerner et al., Reference Lerner, Tokach, DeRouchey, Dritz, Goodband, Woodworth, Hastad, Coble, Arkfeld, Cartagena and Vahl2020).
Metabolic effects of DF on fattening pigs
The use of HF diets in pigs not only aims to elicit effects on intestinal and microbial metabolism but also targets intermediary effects. Different aspects were considered in previous studies, such as the influence of fiber-rich components on the nutritional quality of pork products. Additionally, associated aspects, such as the impact on boar taint, which is a problem for the marketing of pork, have been investigated. The inclusion of 13–20% fermentable fiber from a mixture of SBP, rye bran, and citrus pulp led to a reduction of cholesterol levels in the blood of pigs. Altered cholesterol and bile acid re-absorption from the gut and partial bacterial degradation was considered as an explanation for the observed effects (Kreuzer et al., Reference Kreuzer, Hanneken, Wittmann, Gerdemann and Machmuller2002). Diets with arabinoxylans from rye flakes (66%) or 6.5% raw potatoes and 17% of a maize product as resistant starch sources induced beneficial effects on genes related to colonic health and glycemic responses (Nielsen et al., Reference Nielsen, Theil, Purup, Norskov and Bach Knudsen2015).
The use of chicory at a dosage of 10–13% reduced intestinal indole concentrations and boar taint in male pigs (Hansen et al., Reference Hansen, Stolzenbach, Jensen, Henckel, Hansen-Moller, Syriopoulos and Byrne2008). Boar taint reduction was also tested in diets using raw potato starch (10%) in a combination with WB (5 or 10%) or inulin (5%). Interestingly, no effects on boar odor were observed for an application period of 4–6 weeks before slaughter (Aluwe et al., Reference Aluwe, Millet, Nijs, Tuyttens, Verheyden, De Brabander, De Brabander and Van Oeckel2009).
An experiment with multi-catheterized pigs provided evidence that the type of DF influences the hepatic uptake of certain SCFA. Changing the fermentable substrate from cellulose to a fiber rich in arabinoxylans resulted in increased hepatic propionate and butyrate uptake (Bach Knudsen et al., Reference Bach Knudsen, Jørgensen and Theil2016). Using a comparative metabolomic approach with pigs and human beings, changes in the plasma concentrations of a range of plasma metabolites, including oleic acid, AAs, phosphatidylcholine, LysoPC, betaine, choline, and carnitine, were observed within 30–120 min postprandially, depending on contents and composition of DF in the diet (Nielsen et al., Reference Nielsen, Hartvigsen, Hedemann, Laerke, Hermansen and Bach Knudsen2014).
Intestinal helminths, microbiota, and immune response in fattening pigs
Intestinal helminths are one of the most widespread infections globally in people and animals. They produce metabolites and products that can interact with the digestive process. Ascaris suum, Trichuris suis, and Oesophagostomum dentatum are of significant importance, as these organisms reside in the intestine, a triangular relationship exists between parasites, microbiota, and the pig immune system. These helminths may release antimicrobial products and thereby alter the host's gut microbiota, motility, growth, and gene expression. This was associated with increased production of SCFA and subsequent modulation of immune responses. In return, the microbiota can influence nematode infection at egg-hatching, larval development, and by providing pro- or anthelmintic molecules. Both nematodes and microbiota modulate the immune system and thereby indirectly the microbiome or helminths in the intestinal tract (Midha et al., Reference Midha, Ebner, Schlosser-Brandenburg, Rausch and Hartmann2021). In the interrelation between parasites, the microbiota, and the immune system, a link to the diet composition, in particular DF, seems to play a crucial role in the outcome of infection. Compared to a diet with 30% oat hull meal, the inclusion of 16% inulin or 6% inulin and 15% SBP fiber revealed a reductive impact on worm burdens of pigs infected with T. suis (Thomsen et al., Reference Thomsen, Knudsen, Hedemann and Roepstorff2006; Petkevicius et al., Reference Petkevicius, Thomsen, Bach Knudsen, Murrell, Roepstorff and Boes2007). However, diets with high levels of NSP and lignin tended to increase O. dentatum worm load (Petkevicius et al., Reference Petkevicius, Bach Knudsen, Nansen, Roepstorff, Skjoth and Jensen1997, Reference Petkevicius, Nansen, Bach Knudsen and Skjoth1999). Additionally, piperazine treatment against intestinal parasites became more efficient when pigs received a diet with 21% oat husk meal (Praslicka et al., Reference Praslicka, Bjørn, Nansen and Hennessy1997). A diet with 10% dietary inulin was demonstrated to cooperatively enhance the anti-inflammatory immune response induced by the pig whipworm, T. suis, accompanied by changes in the microbiota composition (Myhill et al., Reference Myhill, Stolzenbach, Hansen, Skovgaard, Stensvold, Andersen, Nejsum, Mejer, Thamsborg and Williams2018). Thus, a fiber supplementation in a pig infected with an intestinal nematode may synergistically influence the bacterial gut composition, the Th2-driven immune response and lead to enhanced mucosal barrier integrity.
In summary, fattening pigs seem to easily adapt to high inclusion of DF in their diets. Supplementation of DF to fattening pigs has been shown to increase the formation of SCFA in the gut and to beneficially affect host metabolism, physiology, and immune responses. Some evidence suggests a beneficial effect of DF against intestinal parasites in fattening pigs, but more studies are still needed here. Therefore, DF offers a promising way to improve the health of fattening pigs.
Conclusion
This review demonstrates that DF has numerous positive effects on the health and well-being of pigs, including sows, piglets, and fattening pigs. DF interacts with many aspects of the pig's digestive physiology, immunology, microbiology, and even behavior. An increasing body of evidence suggests that DF can have the potential to influence piglet health through the sow diet. This makes DF an attractive feed ingredient with regard to offspring manipulation through maternal factors. The diverse origin of DF, which increasingly includes by-products of industrial food processing, leads to large variation in its composition as well as physico-chemical and biological properties. This knowledge gap calls for mechanistic studies on the intestinal and intermediary effects of DF. In addition, a better and uniform characterization and evaluation system for the fibrous feed materials is required in the future. Fibrous feed materials have a great potential to improve the sustainability of pork production under the consideration of animal welfare, but the relationships between type and structure of fiber, inclusion rates, and associated intestinal and metabolic effects are by far not clear and call for more systematic in-depth research on this topic.
Acknowledgments
We thank Dr Stefan Jordan (Charité – Universitätsmedizin Berlin) for reviewing the manuscript.
Financial support
Ł. Grześkowiak was financially supported by the German Research Foundation (Deutsche Forschungsgemeinschaft, DFG) grant GR 5107/2-1.
Conflict of interest
The authors declare no conflict of interest.