In the beginning: 1950s–1970s
Russian (former Soviet) systematic studying of Antarctica started 30 November 1955. At that time the flagship ‘Ob’ left the port of Kaliningrad and sailed to the Southern Polar Region. It was the first ship of the First Complex Soviet Antarctic Expedition under the leadership of Mikhail Somov. In a month, 5 January 1956, ‘Ob’ reached the aim of its trip. On 13 February 1956, the state flag flew up over the building area of the first Russian station in Antarctica (Fig. 1).
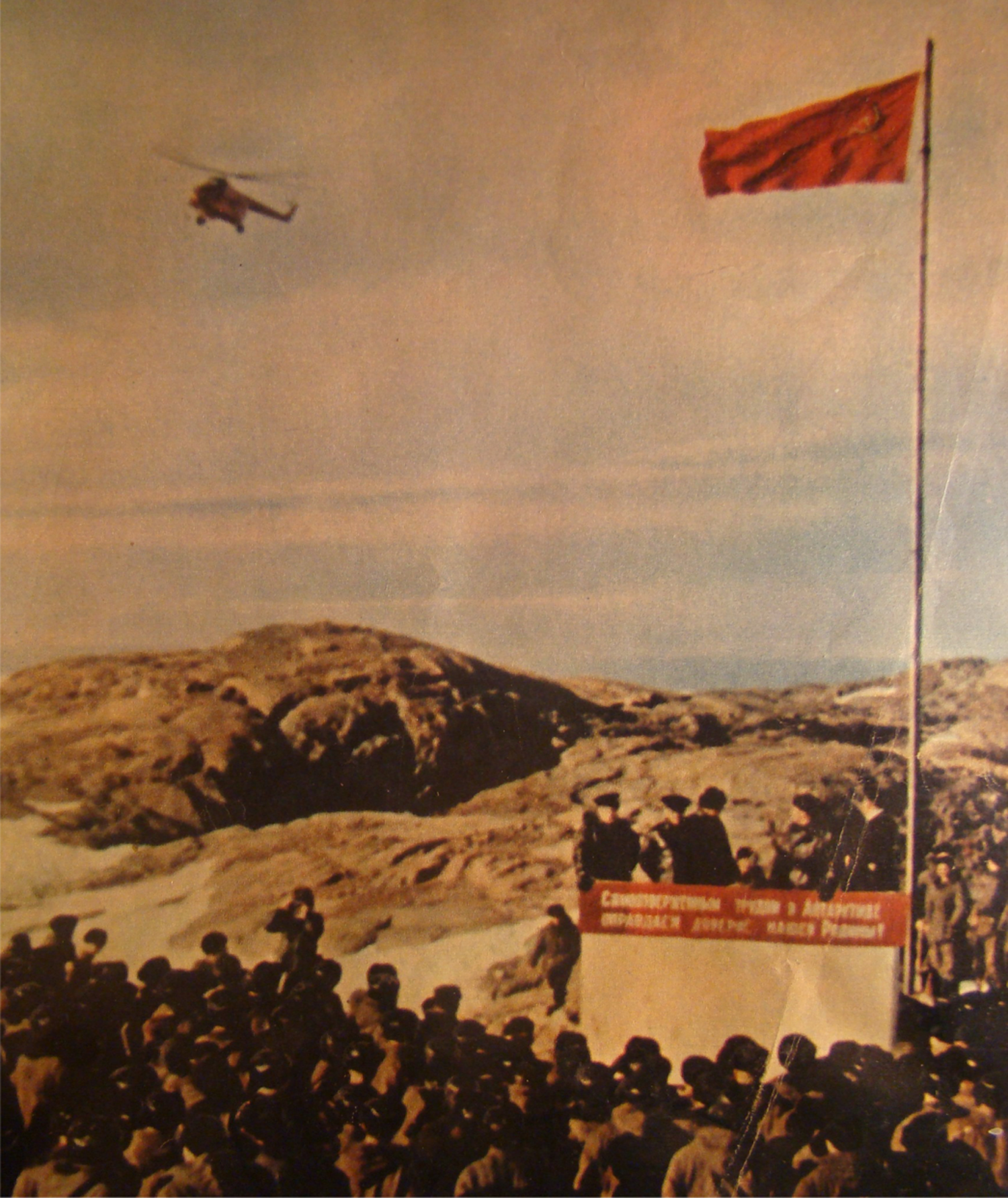
Fig. 1. Establishing the first Russian station Mirny (photo from the cover of Russian magazine ‘Ogonek’, July 1956).
The first 12 years of logistic and scientific activity formed the basic infrastructure for all future work. Keystone stations which were the bases for ground-based and airborne geophysical investigations were opened during those 12 years. On 2 April 1956, the first scientific traverse left Mirny station to inland Antarctic (Fig. 2) lead by Mikhail Somov. On 4 May, after about 400 km over the snowy and windy desert, the tractors stopped and on 27 May, the first Russian inland Antarctic station, Pionerskaya, was opened. Reflection seismic measurements, which were carried out on the traverse, provided the first measurements of ice thickness and subice topography of this part of inland Antarctic. The network of regional scientific traverses, field bases and scientific stations, including Vostok, were formed over the next 11 years (Fig. 3).

Fig. 2. Firsts logistic and scientific traverses from Mirny to inland Antarctic (photo from Treshnikov (Reference Treshnikov1963)).

Fig. 3. Location of Russian radio-echo investigations. Airborne RES in the period of: 1-1955/56–1970/71; 2-1971/72–1973/74; 3-1980/81–1984/85; 4-1985/86–1989/90; 5-1990/91–1994/95; 6-1999/2000–2004/05; 7-2005/06–2009/10; 8-2010/11–2014/15; 9-2015/16–2018/19; ground-based RES: 10-1955/56–1989/90; 11-2003/04–2012/13; 12 – reflection seismic; 13 – area of ground-based RES survey; 14 – area of ground-based RES and reflection seismic survey; 15 – subglacial lakes on (Popov and Chernoglazov, Reference Popov and Chernoglazov2011; Wright and Siegert, Reference Wright and Siegert2011); 16 – outcrops and ice front on ADD7 (2016). AIS, Amery Ice Shelf; AP, Antarctic Peninsula; CL, Cotes Land; DA, Dome Argus; DF, Dome Fuji; DML, Dronning Maud Land; DS, Davis Sea; EL, Enderby Land; FRIS- Filchner-Ronne Ice Shelf; KGI, King George (Waterloo) Island; MRL, Mac. Robertson Land; PB, Prydz Bay; PCM, Prince Charles Mountains; PEL, Princess Elizabeth Land; PM, Pensacola Mountains; PMC, Princess Martha Coast; RB, Ridge B; SRM, Mount Sør-Ronnane; TAM, Transantarctic Mountains; VSL, subglacial Lake Vostok; WS, Weddell Sea; YM, Yamato Mountains.
In February 1964, staff from the Arctic and Antarctic Research Institute (AARI), the leader of Soviet and then Russian polar research, carried out the first radio-echo sounding (RES) tests, a new promising geophysical method based on electromagnetic pulse propagation in the glacier. Reflections from heterogeneous and physical boundaries form the radio-echo time-section which gives fundamental information about the structure of the glacier and also the subglacial topography and morphology (Bogorodskiy and others, Reference Bogorodskiy, Rudakov and Tulpin1965, Reference Bogorodskiy, Bentley and Gudmandsen1985; Navarro and others, Reference Navarro, Macheret and Benjumea2005; Macheret, Reference Macheret2006). This new method was revolutionary in studying polar areas and is cheaper and less time intensive than reflection seismics. Fitting ice radar to aircraft revolutionized the scientific world because it further increases the effectivity of this method. It became possible to study ice sheet and subice relief of Antarctica's 14 million square kilometer covered by ice. The mapping of the subice topography of Antarctica, Greenland, Svalbard and other areas is due to radio-echo sounding.
The first Russian tests and experiments studying the subice topography and ice sheet were carried out using a standard Russian naval radar system GUYS1M4. Its main technical characteristics are listed in Table 1 (Bogorodskiy and others, Reference Bogorodskiy, Bentley and Gudmandsen1985). Two years later, in February 1966, GUYS1M4 was fitted to the short-range Ilyushin Aircraft IL-14 (Fig. 4a). For more than two decades it was used for airborne geophysical surveys. It was the turning point of our investigations of Antarctica because it was the birth of Russian airborne radio-echo sounding (Bogorodskiy and Fedorov, Reference Bogorodskiy and Fedorov1967; Fedorov, Reference Fedorov1967). Two years later, in 1968, using this radar system, the first Russian airborne RES survey in Enderby Land with 50 km between profiles (Fig. 3) was conducted. The total length of the profiles was more than 11,000 km (Kozlov and Fedorov, Reference Kozlov and Fedorov1968; Fedorov, Reference Fedorov1973).

Fig. 4. Russian short-range Ilyushin Aircraft IL-14 (a), the main airline used in 1960s–1980s for airborne geophysical investigations and short-range Antonov Aircraft An-2 (b), which has being used since 1989 (photo from PMGE archive).
Table 1. Main technical characteristics of Russian ice-penetrating radars

In 1967, the AARI research team designed the first deep-penetrating radar RLS-60-67 to study Antarctic ice sheet and subice topography. Its main technical characteristics are listed in Table 1. Technical characteristics of other Russian ice radars of that time can be found in the well-known book of Bogorodskiy and others (Reference Bogorodskiy, Bentley and Gudmandsen1985).
The positive experience from the design and testing of the special ice-penetrating radar system lead to radio-echo sounding surveys in the large coastal area of Mac. Robertson, Princess Elizabeth and Wilhelm II Lands, East Antarctica, under the umbrella of Operation ‘Amery’, the widespread Russian 3-year (1971–1974) geological and geophysical multidisciplinary scientific project (Fig. 3). These airborne surveys, using a Ilyushin IL-14 aircraft, with 20 km spacing between profiles gave the first images of ice thickness and subice topography of this area. These surveys have only recently been updated by Russian, Australian and US radio-echo sounding investigations. Scientific flights with RES were also done to the Pole of Inaccessibility and Vostok Station. At the same time, in the framework of Operation ‘Amery’, a 30 × 30 km seismic reflection grid was acquired (Fig. 3). Despite the venerable age these data still exist and are used for mapping of the bottom of the Lambert Dip (Popov and Kiselev, Reference Popov and Kiselev2018).
The promising scientific results of Operation ‘Amery’ were a good launch-pad for future radio-echo scientific research using the Ilyushin II-14 aircraft. In the period 1975–1984 a seismic reflection survey with a shotpoint spacing of 10 and 20 km was carried out in the western part of the Filchner-Ronne Ice Shelf (Fig. 3). At the same time (1980/81) airborne RES covered the western part of the ice shelf and the Pensacola Mountains (1981/82). In 1984 an ice-penetrating radar system MPI-60 (Fig. 5) with analog registration on 36-mm film was specially designed for airborne radio-echo sounding within the joint project Polar Marine Geosurvey Expedition (PMGE) with the A.M. Gorky Mari Polytechnic Institute (now Volga State University of Technology). Its main technical characteristics are listed in Table 1 (Popov and Kiselev, Reference Popov and Kiselev2018).

Fig. 5. Russian ice-penetrating radar MPI-60 used for airborne radio-echo sounding by PMGE.
At the end of the 1970s Russian scientific investigations were focused in East Antarctica (Fig. 3). Airborne radio-echo investigations were then interrupted and the research was focused on ground-based scientific traverses. During the field seasons of 1978–1984 the studies were carried out on the scientific traverse Mirny–Komsomolskaya–Ridge B, Denman Glacier and Dome Concordia (Bogorodskiy and Sheremet'ev, Reference Bogorodskiy and Sheremet'ev1981; Bogorodskiy and others, Reference Bogorodskiy, Bentley and Gudmandsen1985; Sheremet'ev, Reference Sheremet'ev1986). An RLS-60-74 pulse radar with analog registration, designed by AARI, was used. Its main technical characteristics are listed in Table 1 (Sheremet'ev, Reference Sheremet'ev1986). Investigations in 1979/80 revealed the system of subglacial lakes in Ridge B area (Bogorodskiy and Sheremet'ev, Reference Bogorodskiy and Sheremet'ev1981). Three years later a new method designed by AARI based on analyzing the reflected radio-echo signal gave the possibility of measuring the velocity of ice. It was estimated at 1 m/year (Sheremet'ev, Reference Sheremet'ev1986). The next type of investigations, soundings using the common-depth point technique, were carried out there in the same time to measure the average velocity of the electromagnetic wave propagation in the glacier. It was the first Russian investigation of this type. In Ridge B the velocity was 165.9 ± 2.3 m/μs (Sheremet'ev, Reference Sheremet'ev, Bogorodskiy and Gavrilo1989). Doppler radar navigation for airborne and astronomic navigation for all ground-based work was used during this time. Elevation for the flights was based on barometric measurements.
On 22 February 1968, the Russian station Bellingshausen was opened on King George Island. It was the first station on the Antarctic Peninsula. After that, in April 1970 the AARI research team carried out the first ground-based radio-echo sounding in the Sub-Antarctic (Fig. 3). The investigations were carried out using the ice-penetrating radar with a frequency of 440 MHz (Govorucha and others, Reference Govorucha, Chudakov and Shalygin1974). AARI team designed a number of ice-penetrating radars in that time for different purposes, including investigations in Arctic islands. Their characteristics can be found in Bogorodskiy and others (Reference Bogorodskiy, Bentley and Gudmandsen1985). The next time a Russian team, from Institute of Geography, Moscow, went back to these glaciers with RES was in 1991/92, 1995–2000, 2003 and 2006 (Kotlyakov and Macheret, Reference Kotlyakov and Macheret2016).
Regular airborne RES: from the 1980s until the present-day
After the interruption, airborne geophysical investigations were continued on the regular basis. From 1980 they were systematic and augmented with magnetic (as in Operation ‘Amery’) and sometimes gravity measurements to study the deep structure of the Earth crust and subglacial morphology. Airborne RES on Princess Marta Coast (1986/87) and the Coats Land (1988/89) was acquired with a regular grid with the spacing of 20 km between profiles. In addition, in the Borg Massif area (1986/87) and on the Ritcher Plateau (1988/89), Dronning Maud Land (Fig. 3), airborne surveys were carried out on a grid with the spacing between profiles of 5 km (Popov and Leitchenkov, Reference Popov and Leitchenkov1997).
For the field seasons of 1985/86, 1987/88 and 1990/91, the RES campaigns were concentrated on the eastern part of Dronning Maud Land and on Enderby Land (Fig. 3), with 20 km spacing, using a MPI-60 ice-penetrating radar fitted to Ilyushin IL-14 and IL-18 (1990/91) aircraft (Kurinin and Aleshkova, Reference Kurinin and Aleshkova1987). Doppler radar navigation with barometric measurements for height was used before the introduction of GPS in 1990. The change from Doppler radar to satellite navigation increased the spacial accuracy of the geophysical work from about 500 m, sometimes 1500 m to better than 100 m in the first years using this new technique.
A regional RES survey with 50 km spacing between the flights which covered a wide area of inland Antarctic including Dome Fuji and the Gamburtsev Subglacial Mountains (Fig. 3) was carried out during the field seasons of 1987–1990. Flight laboratory IL-18D GAL, based on the long-range Ilyushin aircraft IL-18, was used. Airborne radio-echo sounding, magnetics and gravimetry measurements were made with this geophysical system. These missions, the first covering the Gamburtsev Subglacial Mountains since its discovery in 1958 (Gan and others, Reference Gan, Drewry, Allison and Kotlyakov2016), also revealed a number of subglacial lakes (Popov and Masolov, Reference Popov and Masolov2007). Doppler radar navigation used in the first campaign of 1987/88 gave an estimation of the positioning error of 4700 m for long profiles. The next campaigns used satellite positioning by satellite navigation system reducing the errors to 150 m (1988/89) and better than 100 m (1989/90).
The longest Russian regional flight in Antarctica between Molodezhnaya and McMurdo stations was undertaken over the Gamburtsev Subglacial Mountains (Fig. 3). Airborne radio-echo and ice-sheet sections are depicted in Figure 6. The later international investigations such as the AGAP project (Antarctica's Gamburtsev Province) further improved our knowledge about this keystone Antarctic region (Bell and others, Reference Bell, Studinger, Fahnestock and Shuman2006, Reference Bell2011; Bo and others, Reference Bo2009; Witze, Reference Witze2009; Cui and others, Reference Cui2010).

Fig. 6. Airborne radio-echo (a) and ice-sheet (b) sections along the regional scientific flight ‘Molodezhnaya Station–McMurdo Station’.
On 7 November 1987, a flight from Molodezhnaya to Vostok was done. At the end of the profile, near Vostok Station, intensive planar subice reflections were registered (Popov and others, Reference Popov, Masolov, Lukin and Popkov2012). This was the result of reflections from Lake Vostok of Lake Vostok discovered after analyzing the remote sensing data (Ridley and others, Reference Ridley, Cudlip and Laxon1993).
After a long interruption, RES investigations in the region of Lambert Glacier were resumed. They were enabled by the opening of the field base Soyuz on the coast of the Beaver Lake, Jetty Oasis, Prince Charles Mountains in December 1982 as logistic support for geological investigations and base for aircraft for future airborne geophysics. In 1985, the PMGE team commenced the systematic radio-echo and magnetic studies of this area (Popov and Pozdeev, Reference Popov and Pozdeev2002). For logistic (especially aviation) support of airborne geophysical and geological studies Druzhnaya-4 (1 January 1987) and Progress (1 April 1988) Stations were opened. For a long time, they have been used as a base for aircraft. Since 2017 this support has been through the aerodrome of Mirny Station.
Ice-penetrating radar MPI-300 (an MPI-60 radar with adjusted frequency) designed by PMGE for investigations of the Arctic islands fitted to an Ilyushin IL-14 aircraft used for one field season of 1985/86. This survey covered the oasis of Else Platform, Prince Charles Mountains, with the spacing between profiles of 2 km chosen for geological reasons. Other surveys in this region have been carried out with a spacing of 5 km between profiles (Fig. 3). Since 1986, the main workhorse for RES has been the MPI-60 although it has been updated several times without changing its principal characteristics (Popov and Kiselev, Reference Popov and Kiselev2018).
In 2013, a new ice radar (PLK-130) was designed and introduced into service in January 2015. Its main technical characteristics are listed in Table 1 (Popov and Kiselev, Reference Popov and Kiselev2018). The location of the radio-echo profiles in the area of Lambert Glacier is depicted in Figure 3. Ilyushin IL-14 aircraft used until 1989 was replaced by an Antonov An-2 biplane (Fig. 4b) which is still in use.
Before 2000, airborne radio-echo sounding data had been registered on 35-mm film. Digital data acquisition revolutionized geophysics by making possible computer signal processing which enhanced its possibilities. The first analog-digital converter (ADC) characterized by a 140 ns interval with 6-bit discretization. New ice-penetrating airborne radar RLK-130 designed in 2013 has ADC with 24 bit and 38.46 ns of discretization sampling interval. A detailed technical description of this period is well described in Popov and Kiselev (Reference Popov and Kiselev2018).
Ground-based RES investigations in scientific traverses: 1997–2013
The motivation for resuming the Russian scientific traverses was the discovery of Lake Vostok in the 1990s (Ridley and others, Reference Ridley, Cudlip and Laxon1993; Kapitsa and others, Reference Kapitsa, Ridley, Robin, Siegert and Zotikov1996). Because the location of Lake Vostok is very close to Vostok Station it opened widespread possibilities. In 1998 PMGE team completely upgraded the old RLS-60-74 ice-penetrating radar system (see above) to RLS-60-98 and commenced ground-based investigations of Lake Vostok. Technical characteristics of this radar are presented in Table 1. The maximal ice thickness recorded by RLS-60-98 is 4350 m (northern part of Lake Vostok). This is the absolute record for the Russian ice-penetrating radars (Popov and others, Reference Popov, Masolov, Lukin and Popkov2012). In 2006 RLS-60-98 was improved. New version RLS-60-06 has practical and the same technical characteristics (see in Table 1), but more powerful transmitter.
Over the lake, radio-echo sounding of the ice–water interface was carried out together with reflection seismic (commenced in 1995) measurements of the depth of the lake. Special mobile block Vityaz which was carried by old Russian vehicles Ishimbay, STT-1 Kharkovchanka, STT-2 Kharkovchanka-2 and ATT (Fig. 7a) has been used. Ground-based RES and reflection seismic soundings were finished in 2008. A total of 5190 km of RES profiles and 318 reflection seismic measurements were carried out (Popov and others, Reference Popov, Masolov, Lukin and Popkov2012). Besides, allowing for better understanding of the Earth's crustal structure, passive seismic investigations have been carried out on the profile across the Lake Vostok near Vostok Station (Isanina and others, Reference Isanina, Krupnova, Popov, Masolov and Lukin2009; Popov and others, Reference Popov, Masolov, Lukin and Popkov2012).

Fig. 7. Russian STT-2 Kharkovchanka-2 with mobile block Vityaz (a) used for ground-based RES and new mobile block (b) carried by Kässbohrer Pisten Bully Polar (photo by S. Popov).
Deep ice core drilling at Vostok Station was commenced in 1970. The historical review of the drilling is well described in Vasiliev and others (Reference Vasiliev2007). By 1993 the borehole practically reached the water-table. Drilling was interrupted for a short time. The estimation of ice thickness in the vicinity of the drilling site was very important to avoid the drilling fluid contaminating the lake. The next important task was to determine if Lake Vostok was closed or connected with the external subglacial hydrological network. It was important due to the theoretical possibility of water outbursting from Lake Vostok (Clarke, Reference Clarke2006; Evatt and others, Reference Evatt, Fowler, Clark and Hulton2006; Wingham and others, Reference Wingham, Siegert, Shepherd and Muir2006; Evatt and Fowler, Reference Evatt and Fowler2007). We note that Russian (Popov and others, Reference Popov, Masolov, Lukin and Popkov2012) and US (Studinger and others, Reference Studinger2003) data demonstrated that such an outburst of the lake is unlikely in the lakes current configuration (Richter and others, Reference Richter2014a,Reference Richterb).
To increase the accuracy of the ice thickness measurements vertical seismic profiling in the 5G borehole (1996–98), and multi-offset radio-echo sounding in the vicinity of the drilling site (1999/2000) were undertaken. The acoustic velocities in meteoric ice and water were measured as 3920 ± 20 and 1490 m/s, respectively; the average acoustic velocity in the glacier was measured as 3810 ± 20 m/s (Masolov and others, Reference Masolov, Lukin, Sheremetyev and Popov2001). The average velocity of the electromagnetic wave propagation in the glacier was 168.4 ± 0.5 m/μs. Ice thickness in the drilling site vicinity was predicted to be 3760 ± 30 m based on the seismic data, and 3775 ± 15 m based on RES data (Popov and others, Reference Popov2003). During the penetration of Lake Vostok on 5 February 2012, the ice thickness was 3758.6 ± 3 m (Lipenkov and others, Reference Lipenkov, Ekaykin, Polyakova and Raynaud2016). Therefore, the reflection seismic data were extremely accurate in comparison to the RES data which was less accurate than expected. This requires further revision and investigation.
Compilation of Russian and US (Studinger and others, Reference Studinger2003) data collected around and over Lake Vostok better reveals the features of the ice sheet and subice topography of this area. The ice surface over Lake Vostok is very flat and horizontal with a small slope of about 0.5′ to the south, but the ice thickness over the lake vary within 3600–4350 m. Due to the hydrostatic equilibrium, the boundary of the lake range from −600 m above sea level in the north to −150 m in the south. The total volume of Lake Vostok is about 6100 km3, the average depth is about 400 m, and the maximum depth is 1200 m (Popov and others, Reference Popov, Masolov, Lukin and Popkov2012). RES investigations revealed that the lake is completely isolated with a total area of 15790 km2, excluding 365 km2 occupied by 11 islands. Fifty-six isolated subglacial water caves have been identified outside Lake Vostok (Popov and Chernoglazov, Reference Popov and Chernoglazov2011). Subice topography and the Lake Vostok depth is shown in Figure 8.

Fig. 8. Subice topography and water caves in the Lake Vostok area. 1 – bedrock topography contours in meters; the contour space is 150 m; 2 – sea level (surface of WGS-84); 3 – Lake Vostok shoreline; 4 – subglacial lakes. Location chart is shown in section a; Russian RES profiles are shown by red lines; US airborne profiles (Studinger and others, Reference Studinger2003) are shown by green lines; seismic sounds are shown by yellow dots. The depth of Lane Vostok is depicted in section b. Contour interval is 150 m.
Russian scientific traverses resumed on January 2004. Initially, they operated between Mirny and Vostok stations using old Russian vehicles Ishimbay, STT-1 Kharkovchanka, STT-2 Kharkovchanka-2 and ATT (Fig. 7a). Due to the Russian strategy for the supply of Vostok Station, since 2008 the logistic hub has been at Progress Station, therefore the scientific traverses also have to be operated between these stations (Shibaev and Savatugin, Reference Shibaev and Savatugin2008). The vehicles also have been changed to Kässbohrer Pisten Bully Polar ATT (Fig. 7b). Ice-penetrating radars RLS-60-98 and RLS-60-06 were used. The location of RES profiles is shown in Figure 3. These glaciological observations were conducted by the AARI team with geodetic measurements by the team from Technische Universität Dresden, Institut für Planetare Geodäsie (TUD). Due to technical problems with the RLS-60-06, an Italian INGV-IT radar system was used, which was previously well-proven (Tabacco and others, Reference Tabacco2002) and kindly provided by Dr. A. Zirizzotty, Istituto Nazionale di Geofisica e Vulcanologia (INGV, Rome, Italy). This scientific instrument suite yielded a better understanding of the ice-sheet dynamics and structure of inland Antarctic (Richter and others, Reference Richter2010; Schröde and others, Reference Schröder2017) including revealing the main features of the subice topography and the ice sheet. Figure 9 shows the radio-echo and ice-sheet section along ‘Mirny–Vostok’ scientific traverse. Subice studies lead to the discovery of the lake 50 km north from Komsomolskaya Station (named Komsomolskoe Lake) and the system of the lakes under Pionerskaya Station (main lake which is just under the station and is named Pionerskoe Lake). Details of the surveys were described in Popov and others (Reference Popov, Masolov, Lukin and Popkov2008), Popov (Reference Popov2015) and Popov and Popkov (Reference Popov and Popkov2015).

Fig. 9. Radio-echo (a) and ice-sheet (b) sections along the regional scientific traverse ‘Mirny Station–Vostok Station’.
Ground-based GPR investigations: since 2012 until now
It is well-known that the GPR technique is the most efficient method to study the near-surface layer of the Earth for wide range scientific and applied tasks, especially over snow and ice sheet (Plewes and Hubbard, Reference Plewes and Hubbard2001; Annan, Reference Annan2002, Reference Annan, Rubin and Hubbard2005; Jol, Reference Jol2008; Blindow and others, Reference Blindow, Salat and Casassa2012; Bristow, Reference Bristow2013). Russian GPR investigations in Antarctica was commenced in the field season of 2012/13. A commercial GSSI SIR10B (Geophysical Survey Systems, Inc., USA) GPR with 200 MHz antenna has been used near Vostok and Progress stations and also along the Progress–Vostok scientific traverse. A GSSI SIR-3000 (Geophysical Survey Systems, Inc., USA) GPR with 400 MHz antennae has been also applied for investigations around Progress Station. Widespread research was carried out including studying the megadune area close to Vostok Station (Ekaykin and others, Reference Ekaykin2016), also the snow-firn structure of the Lake Vostok area and along the scientific traverse between Vostok and Progress stations (Popov and Eberlein, Reference Popov and Eberlein2014). Glaciological studies by the AARI team and geodetic studies by the TUD team have been carried out along with RES investigations.
After that, GPR investigations were concentrated in the vicinity of Progress and Mirny stations for revealing and studying crevasses for safety reason, and also to test and better interpret GPR time-sections for safety applications. GPR techniques are the best geophysical method to reveal and study crevasses in glaciers (Arcone and Delaney, Reference Arcone and Delaney2000; Taurisano and others, Reference Taurisano, Tronstad, Brandt and Kohler2006; Zamora and others, Reference Zamora2007; Eder and others, Reference Eder, Reidler, Mayer and Leopold2008). During three field seasons of 2013–2016 glaciological and GPR engineering surveys have been carried out close to Mirny Station to find the place to site a new airfield for operating medium-range aircraft with ski landing gear. Aviation activities at Mirny Station has been interrupted since 1991 and its resumption was a very important logistical task. In addition, the current plans for airborne geophysical investigations (including RES) include the surveying of the Mirny Station area and to the east. Therefore the snow-airstrip there is very important also for Russian scientific works.
Engineering surveys included aerial photography, GPR profiling, ice core drilling and the installation of benchmarks to measure the velocity of the glacier motion. Finally, these works provided the choice of location and construction of the snow runway (Popov and others, Reference Popov, Mezhonov, Polyakov, Mart'yanov and Lukin2016, Reference Popov, Polyakov, Pryakhin, Mart'yanov and Lukin2017; Popov and Polyakov, Reference Popov and Polyakov2016). On 10 February 2016, all these investigations provided the first middle-range aircraft DC-3T (BT-67) ‘Turbobustler’ landed there (Fig. 10). Since 2017, snow runway of Mirny Station has been used to carry out the Russian airborne geophysical works.

Fig. 10. The first landing of the aeroplane on the snow-runway at Mirny Station after a long interruption (photo by S. Popov).
The marginal zone of the Antarctic ice sheet is rich with crevasses. The vehicles of logistic traverses sometimes open-up cracks when crossing snow bridges and may partly drop into crevasses (Fig. 11a). Therefore the second important direction of the Russian GPR engineering surveys is in revealing crevasses and subice water channels to provide safety of the logistic traverses from Progress to Vostok. This is more important due to the delivery of the super-heavy cargo to Vostok Station for the construction of new buildings. Engineering surveys at Progress Station area were commenced in the field season of 2014/15 and included GPR, geodetic, glaciological and hydrological investigations. GPR investigations were carried out using GSSI (Geophysical Survey Systems, Inc., USA), Zond-12 (Radar Systems, Inc., Latvia) and OKO-2 (LOGIS Inc., Russia) radar systems with frequencies of 150 MHz, 270 MHz, 400 MHz and 900 MHz (Popov and Polyakov, Reference Popov and Polyakov2016). Annual monitoring reduced the risks of accidents. A typical GPR time-section obtained over a wide crevasse is depicted in Figure 11b.

Fig. 11. Crevasses at the logistic traverse Progress–Vostok where a fuel tank fell into in 2012 (a) and GPR time-section at 900 MHz obtained at this crevasse during the 2014/2015 field season (b) (photo by RAE team).
Frequently, but not every year, Russian scientists also carry out the GPR research on the marine ice for the applied task of ship unloading and construction of snow-airstrips. The main results can be found in Popov and others (Reference Popov, Kuznetsov, Pryakhin and Kashkevich2018).
Not only crevasses are dangerous. On 30 January 2017, the wide depression 183 m × 220 m, about 20 m average deep with the deepest value of 43 m was formed in the western side of the Dålk Glacier, not far from Progress Station. It completely destroyed the main road which connected the station with the airdrome and the start point of the logistic traverses to Vostok Station. Eyewitnesses said that it was only luck that prevented the death of 20 people who crossed this area on a tractor less than half an hour before the dip formation (Popov and others, Reference Popov, Pryakhin, Bliakharskii, Pryakhina and Tyurin2017). Next year GPR engineering survey proceeded to find a new safe path bypassing the dip. On 23 January 2018, the returning logistic traverse from Vostok Station followed this new route. It provides the safe return of the vehicles to Progress Station for wintering conservation.
Formation of the dip displays a new challenge. A subglacial water body was located underneath the dip, fed by melted water, then ice stress increased and the water outburst. Emptying the water cave increased the stress in the icy roof. Overloaded it crashed. The outburst of the lakes near the Russian stations and field bases is observed very often and GPR research is of great use in the identification and risk estimation of such features.
Concluding remarks
Russian (former Soviet) systematic studying of the ice-sheet and subglacial topography investigations by radio-echo sounding has been ongoing for 55 years. About 5.5 million sq. km of the Antarctic continent has been covered by our surveys. It has contributed significantly to a better understanding of its structure and evolution. The results have been presented in a number of national and international projects such as Bedmap (Lythe and others, Reference Lythe and Vaughan2001) and Bedmap2 (Fretwell and others, Reference Fretwell2013). GPR techniques together with classic glaciological observations have enabled the detailed study of the near-surface zone especially for safety reason, and also subglacial hydrological processes. Russian investigations have had success in translating these scientific insights to more applied problems.
Acknowledgments
The author thanks his colleagues from PMGE team (Yu.B. Chernoglazov, V.M. Kirillov, A.V. Kiselev, V.N. Masolov, A.M. Popkov, A.I. Savelov, A.N. Sheremet'ev and O.B. Soboleva), AARI and Russian Antarctic Expedition (V.V. Kharitonov, V.V. Lukin, V.L. Mart'yanov, A.L. Novikov, S.P. Polyakov and S.S. Pryakhin) and St. Petersburg State University (S.D. Grigoreva, A.A. Sukhanova) with whom he worked in the field and processed RES and GPR data; also tractor drivers who provided the scientific investigations in the logistic and scientific traverses ‘Mirny–Vostok’ and ‘Progress–Vostok’, also the wintering and summaring team of Vostok, Progress and Mirny stations. The author also thanks Achille Zirizzotti and Stefano Urbini from INGV for RES and GPR equipment and also Dr. L. Eberlein, L. Schröder and A. Richter, his colleagues from TUD for help in the field. The author thanks Martin Siegert and three anonymous reviewers for numerous corrections and suggestions for improving English that allowed him to significantly improve the manuscript. This study was funded by Russian Foundation for Basic Research (RFBR) according to the research project No 17-55-12003 NNIO.