1. Introduction
In the framework of the German Antarctic glaciological research program, accumulation rates and the isotopic content of the near-surface layers in the eastern part of the Ronne Ice Shelf have been determined. These investigations have been carried out in relation to mass-balance and ice-core studies. Sampling started during the pre-site survey for the German overwintering base in 1979–80 (Reference ReinwarthReinwarth, 1981) and has continued during the 1983–84, 1985–86, 1989–90 and 1991–92 field seasons. Accumulation rates based on the evaluation of only one 10 m firn core at Filchner Station and ten snow pits were published by (Reference Reinwarth, Rauert, Stichler and MoserReinwarth and others 1982, Reference Reinwarth, Graf, Stichler, Moser and OerterReinwarth and others 1985) and Reference Graf, Moser, Oerter, Reinwarth and StichlerGraf and others (1988). The δ18O temperature relationship, though still debatable, was used for the climatic interpretation of the 18O content of a 100 m ice core (Reference Graf, Reinwarth and MoserGraf and others 1990a). A remarkable step forward was taken during the 1989 90 and 1991–92 field seasons by drilling 16 firn cores to 10 m depth and two ice cores to 215 and 320 m depth. The sampling locations cover much of the central part of the Ronne Ice Shelf. Also, the area of Berkner Island (Reference WagenbachWagenbach and others, 1994), the Filchner Ice Shelf at the ice edge and the area south of Henry Ice Rise were sampled in 1990 with the help of the German Do-228 aircraft Polar 2 and Polar 4. Great effort has been expended to date the firn cores as accurately as possible by combining several stratigraphical methods. Consequently, the distribution of the accumulation rates and of the stable-isotope content of the firn, as well as the δtemperature relationship, can now be established more accurately. The results from the two cores from the southernmost part of the ice shelf are very helpful in estimating particle paths in the ice shelf and in interpreting the structural parameters of ice cores B13 and B15
2. Sampling and experimental procedures
Figure 1 shows the area under investigation with the drilling sites of the 10 m firn cores and of the two deeper ice cores B13 and B15. The 10 m firn core at point D246 and ice core BIS were drilled in 1992 (Reference Oerter, Drücker, Kipfstubl, Nixdorf, Graf and OerterOerter and others, 1992a) and the other firn cores and ice core B13 in 1990 (Reference Miller and OerterMiller and Oerter, 1991). As part of the glaciological program, in all locations the temperature of the firn at approximately 10 m depth was measured. The drilling sites of eight firn cores are located at or close to the flowline through B15 and B13, four sites lie on the parallel flowline 50 km to the northwest, one at Filchner Station, one in a direction toward Berkner Island and the last two on the northern and southern domes of Berkner Island, Reinwarthhohe and ThyssenhÖhe, respectively (Institut fr Angewandte Geodäsie, 1993).
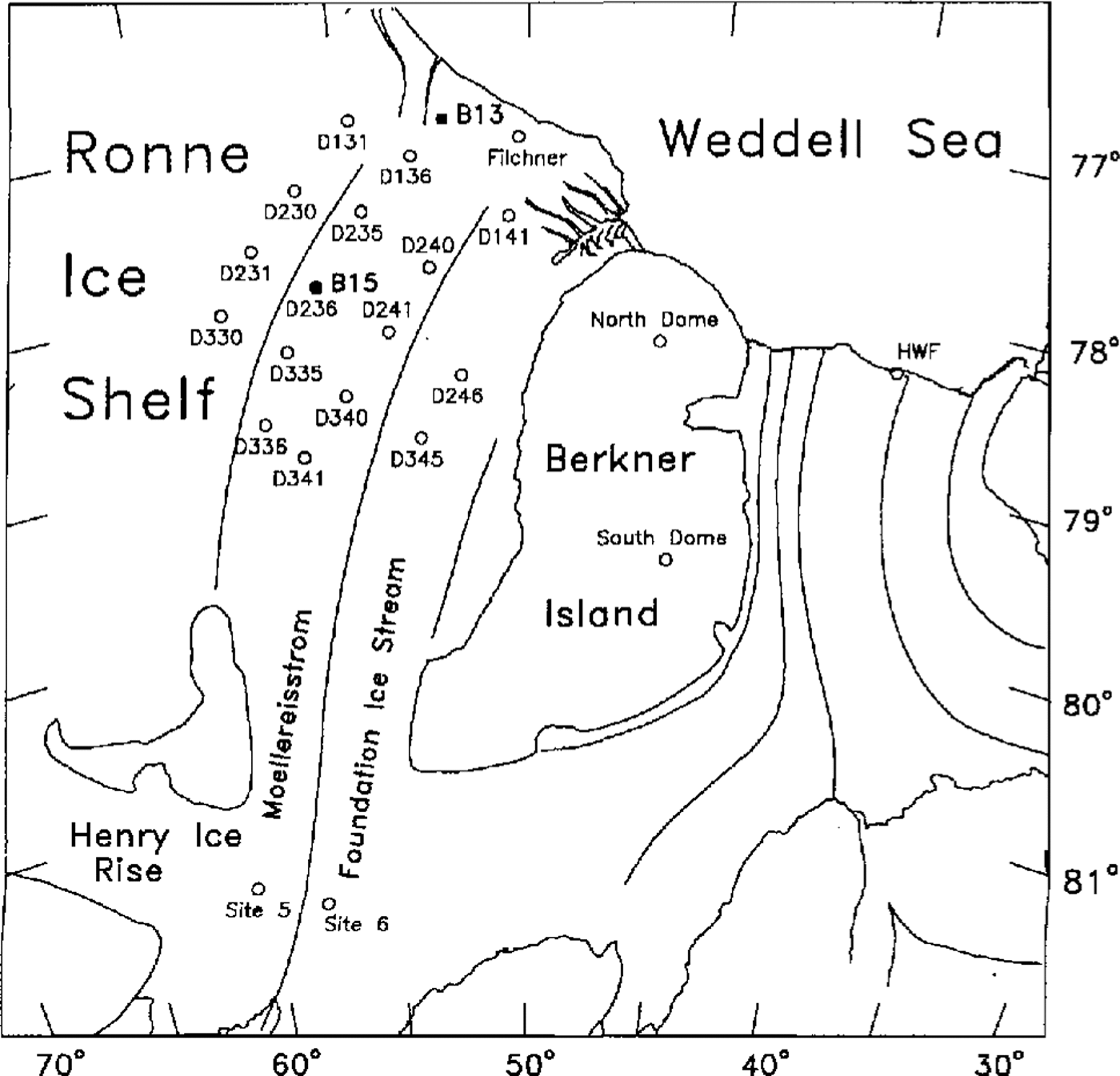
Fig. 1. The drilling sites of the 10 m firm cores and of ice cores B13 and B15 on the Ronne Ice Shelf (map after Institut für Angewandte Geodihie, 1993)
The bulk d.c. conductivity (ECM method) of the firn was measured in the laboratory to obtain initial information on the annual layering (Reference Minikin, Wagenbach, Graf and KipfstulMinikin and others, 1994), To ensure a consistent depth scale for all high-resolution profiles, the cores were sub-sampled in the same way for isotopic and chemical analyses. Depth resolution was designed to get at least ten samples for each annual layer according to the signals in the ECM profiles. The samples were analyzed with respect to their stable-isotope content (2H, 18O) and chemical constituents. Among the chemical profiles, only the non-sea-salt (nss) sulfate records were used for dating purposes in this paper (Reference Minikin, Wagenbach, Graf and Kipfstulfor further details, see Minikin and others (1994)). The 3H contents were measured on integrated samples over annual layers according to the δ2H stratigraphy.
The data on the deep ice cores reported here were measured on drill-chip samples for B13 (Reference OerterOerter and others, 1992b) and core segments of approximately 20 cm length for B15 (Reference Oerter, Drücker, Kipfstubl, Nixdorf, Graf and OerterOerter and others 1992a). High-resolution profiles of ice core B13 in selected depth ranges have been given by Minikin and others (Reference Minikin, Wagenbaeh, Graf, Reinwarth, Oerter, Miller and Oerter1991)
The 2H contents are expressed as δ values With respect to V-SMOW (Vienna Standard Mean Ocean Water) measured with a precision of 1.0‰.3H contents arc given in tritium units (TC) and referred to the measuring date, March 1992.
3. Isotope profiles and dating of the firn cores
The firn cores were dated using the δ2H and, to some extent, the 3H and chemical profiles (Table 1). Both the stable isotopes and the non-sea-salt (nss) sulfate profiles show seasonal variations. It is well known that the 2H content of precipitation is governed by the local temperature (Reference DansgaardDansgaard, 1964). The nss sulfate concentration of the Antarctic troposphere appears to be controlled by the exhalation of dimethyl sulfide from the Southern Ocean and hence shows a pronounced summer maximum at coastal sites (Reference Wagenbach, rlach, Moser and nnichwagenbach and others, 1988; Reference Savoie, Prospero, Larsen and SaltzmanSavoie and others, 1992). Therefore, the seasonal concentration pattern is similar for both parameters. In the firn, these parameters provide highly valuable stratigraphic information depending on the build-up of the snow cover. To distinguish between layers accumulated in different years by stable-isotope profiles, at least parts of both the summer arid winter precipitation have to be preserved in the snow cover, otherwise a quasi-periodic signal, necessary for reliable dating, is not detectable. To distinguish between nss sulfate peaks of different years, it is sufficient that the summer layers with their relatively high sulfate peaks are separated by some layers accumulated in autumn, winter or spring.
Table I Mean annual values δ2H and accumulation rates deduced from shallow firn cores drilled in 1990 and 1992 in the eastern part of the Ronne Ice Shell and from snow pits dug in 1984 and 1986. The deviation (1σ) gives the scatter of the mean annual values

At some locations, or during years with low-accumulation rates, the stratigraphy may be seriously affected by the build-up of the snow cover, which depends primarily on the already-mentioned seasonal distribution of precipitation but also on the re-working of the snow surface by wind action, e.g. wind scouring (Reference Fisher, Koerner, Paterson, Dansgaard, Gundestrup and ReehFisher and others, 1983). Note that the stable isotopes are additionally smoothed by diffusion processes during the transformation of snow to firn and ice Reference JohnsenJohnsen, 1977)
The 3H content in the troposphere also varies with the season (Reference Taylorsee e.g, Taylor, 1968). Since 3H measurements are very time-consuming, we measured only the 3H content of annual layers, which seems sufficient for dating purposes. According to investigations at the South Pole (Reference Jouzel, Merlivat, Pourchet and LoriusJouzel and others, 1979), 3H distributions in the Antarctic firn show distinctive features, which were used for the interpretation of the 3H profiles: a strong increase in the 3H content starting in 1963 after the nuclear weapons test series in the early 1960s. an absolute maximum in the years 1965-66 and a slow-down during the 1970s after the moratorium with a secondary maximum in 1973.
3.1. High-resolution δ2H and nss sulfate profiles
The δ2H depth profiles of four firn cores and the corresponding dating are shown in Figure 2. Obviously, the observed amplitudes of the seasonal δ2H variation depend on the accumulation rates and are lowest at sites in the southernmost part of the ice shelf Annual-layer identification is based on the stable-isotope profiles but also on ECM, nss sulfate and 3H profiles, which supplement each other. For example, at D335 (Fig. 3) the firn at a depth of 2.6m contains two summer horizons according to nss sulfate, whereas the δ2H profile displays only one maximum, which is broadened by a shoulder. Obviously, the winter accumulation with its low δ2H value is missing here, similarly at D336 at the 3.1 m depth (Fig. 2).

Fig. 2. Profiles of theδ2H values (full lines) and 3H contents (dashed lines) of the 10m firn cores drilled at D136, D336, BAS site 5 and BAS site 6. The dating deduced from the profiles is indicated; the annual layering could be deduced from the δ2H profiles only at D136 and D336. 3H contents are referred to the measurement date, March 1992.

Fig. 3. High-resolution profiles of the δ2H values and of the non-sea-salt (nss) sulfate concentrations of the firn core drilled at D335. The annual layering deduced from the profile is indicated.
3.2. 3H profiles
The distributions of the 3H contents were determined at D136, D230, D236, 8330, D336 and BAS sites 5 and 6.The tritium fall-out at Berkner Island has been discussed by Wagenbach and others (Reference Wagenbach1994). At D136 and D336, the appearance of the absolute maximum in 1966 strongly confirms the stratigraphical dating technique (Fig. 2). The temporal 3H change in the late 1960s and 1970s, less significant than the increase after 1963, is at least compatible with that at the South Pole and therefore supports the stratigraphic dating of the firn cores.
The annual sub-sampling at BAS sites 5 and 6 was based on the first interpretation of the stable-isotope profiles (Reference Graf, Miller and OerterGraf and others, 1991). The measured 3H content made it evident that this initial dating was wrong. Therefore, the sampling error at BAS sites 5 and 6 is on the order of the thickness of an annual layer. Because of this error and due to the much higher 3H fall-out at these sites, the 3H distribution is somewhat broader. At site 5, relatively high 3H contents are found at 2 m depth; this horizon was assigned to 1966, according to the fallout history at the South Pole. The very sharp increase of 3H content at site 6 occurring at 2.2 m is most probably caused by the fall-out in 1965. At site 5, the high-resolution δ2H profile shows only 13 cycles down to 2 m depth and the nss-sulfate profile 18 of 25 layers, according to the 3H dating. Accumulation rates, derived from the 3H dating, are about 90 kg m-2 a-1) at both sites and therefore are lower by factors of 1.7 and 1.5, respectively, than hitherto assumed.
4. Accumulation rates and the δ2h values of surface layers
The accumulation rates and the mean 2H values of the surface layers follow from the dating given in the previous section. They are given in Table 1 and their regional distribution is given in Figures 4 and Figures 5. For completion, the results from the previous snow-pit studies are added. Generally, the accumulation rates and the δ2H values represent means of the mean annual values ovcr the period covered by the cores or snow pits. Only annual layers, which encompass the entire deposition sequence for a year, are taken into account. The standard deviations indicate the year-to-year variability, which is caused by the build-up of the snow cover. However, averaging over several years results in rather representative mean values. Assuming a possible dating error of I year, the accumulation rates arc accurate to about 5%. The inaccuracy of the density values introduces an error of the same order.

Fig. 4. Distribution of the δ2H values of the near-surface layers in the eastern part of the Ronne Ice Shelf. Isolines of δ2H values are plotted at intervals of 8‰

Fig. 5. Distribution of the accumulation rates in the eastern part of the Ronne lce Shelf: lsolines of accumulation rates are plotted at intervals of 10 kg m2 a 1.
The δ2H value in the near-surface firn decreases from -195‰ at the drilling site of BI3 (BC90), 30 km distant from the ice edge, to -251‰ at BAS sites 5 and 6 south of Henry Ice Rise. Along the flowline through BI3 and B15, δ2H decreases by 9.1‰ per 100 km to the south. The accumulation rates decrease accordingly from 200 to 90 kg m-2 a-1.
5. The δ2h-temperature relationship
The areal analysis of the stable-isotope content of near-surface firn and the 10m firn temperature provides information on the sensitivity of the isotopic content to temperature changes (Reference Robin and RobinRobin, 1983). The δ2H values of the near-surface layers in the eastern part of the Ronne Ice Shelf (Table 1) are closely correlated to the 10 m firn temperatures, representing the mean ambient temperatures at the measuring sites (Fig. 6). The best correlation is found between δ2H values of firn cores drilled in 1990 and firn temperatures θ measured in the same year, which is described by the regression line δ2H = 42 + 10.3 (±0.6)θ, r = 0.97 (line l in Fig. 6). The values from snow-pit samples taken in 1984 and 1986 do not fit this line and change abruptly between points D141 and D240. They may, however, be correlated by two separate regression lines for the sites close to and away from the ice edge, respectively, which are nearly parallel to line I. The data set of the near-coast stations was complemented by values from the coastal station Druzhnaya I (Reference Graf, Reinwarth, Oerter and MillerGraf and others, 1990b).

Fig. 6 Relationship between the δ2H values of near-surface layers and the firn temperature at 10 m depth in the eastern part of the Ronne lce Shelf: See text for the explanation of the different regression lines 1, 2 and 3.
The shift between the regression lines may reflect calibration errors of the thermocouple used for measuring the 10 m firn temperature. However, shifts of the regression lines parallel to each other are of no importance for the calibration of isotope climatic records because only the gradients (dδ2H/dθ) are of interest.
The temperature gradient of the δ2H values (dδ2H/dθ)on the Ronne Ice Shelf is surprisingly large compared to δ2H-θ relationships found in other areas of Antarctica (Reference Robin and RobinRobin 1983;Fig. 7). The gradients may reflect the conditions of the snow-forming processes during moist-air advection, governed mainly by the isobaric cooling of air masses over the Ronne Ice Shelf; whereas adiabatic cooling prevails on the Antarctic Peninsula (Reference Peel and ClausenPeel and Clausen, 1982 and along the traverse from Durmont d'Urville to Dome C (Reference Lorius and MerlivatLorius and Merlivat, 1977), leading to lower temperature gradients of dδ2H/dθ = 7.2 and 6.0‰K1, respectively. In addition, in the interior of Antarctica the difference between the surface temperature and the temperature above the inversion affects the δ2H-θ relationship. Though the gradients are different, all values follow roughly a common regression line. This suggests a similar history of the advected moist-air masses reaching Antarctica.

Fig. 7. Relationship between theδ2H values of near-surface layers and the firn temperature at 10 m depth in three areas of Antarctica
6. The relation of the δ2h values of ice cores bl3 and bl5 to the surface data
Ice core B13 contains ice of meteoric origin down to 152.8 m, followed by the so-called marine ice accumulated from the water column (Reference OerterOerter and others, 1992b). At B15, 140 km south of B13, the boundary between the ice of different origins is found at the same depth. In this section, we will give three examples of the application of surface data in interpreting the δ2H profile of the meteoric ice Further information on the structural parameters of the two ice cores have been given by Eicken and others (Reference Eicken, Oerter, Miller, Graf and Kipfstuhl1994).
The relatively high flow velocities of the Ronne Ice Shelf mean that the deepest ice of meteoric origin recovered from the cores was deposited far upstream of the drilling site. The δ2H values of ice cores B13 and B15 reflect the progressive depiction of the snow in the heavier isotope 2H along the flowline to the south (Fig. 8). δ2H variations caused by climatic changes are superimposed and can only be separated by knowing both the origin of the ice by ice-flow modeling, e.g. using the simple model of Thomas (Reference Thomas1973) and the δ2H distribution along the principal surface flowline.

Fig. 8. The δ2H values of cores Bl3 and Bl5 in comparison to the values of the near-surface layers on the Ronne Ice Shelf at locations along the flowline through BI3 and B15. The ice below 137 m in core BI3 and below 142 m depth in core Bl5 originated from the inland ice.
On the other hand, because the expected climatically induced δ2H variations are small compared to the spatial changes upstream from the drilling site of the cores, the origin of the ice may be estimated at least qualitatively from a comparison of the ice core and surface-snow isotope data (Fig. 8).For example, the δ2H value suggests that the ice at a 100 m depth originates from the region south of Henry Ice Rise.
The δ2H values decrease rapidly below 137 m in BI3 and below 142 m in B15 to about -300‰. It is unlikely that such low values would be encountered at the surface of the Ronne Ice Shelf, even in the region of the grounding line as indicated by the broken line in Figure 8. we conclude, therefore, that the ice in these deepest layers was deposited on the ice sheet at higher elevations. Only in the improbable case that the δ2H values in the surface snow decrease between BAS site 5 and the grounding line by 50‰ within a distance of only 100km (about five times faster than between the ice edge and BAS site 5) could the ice originate from precipitation on the ice shelf. From the rather small thickness of the layer in BI3 originating from the inland ice, strong basal melting is inferred between the grounding line and the area where basal accumulation starts (Reference OerterOerter and others, 1992b).
Acknowledgements
Financial support of this work by the Deutsche For-schungsgemeinschaft (DFG) is greatly acknowledged. In particular, we thank Mr Rauert for carrying out the 3H measurements.