Of all the macronutrients, proteins have repeatedly been shown to be the most satiating, either over the short term as well as over 24 h(Reference Lejeune, Westerterp and Adam1–Reference Poppitt, McCormack and Buffenstein5). The satiating properties of proteins seem to depend on the protein source: for instance, ingestion of whey protein resulted in a more pronounced satiety, and a decreased energy intake during a subsequent meal, than ingestion of casein(Reference Hall, Millward and Long6). The authors suggested that the difference between these two protein sources might be mediated by differences in digestion and absorption rate. Thus, compared with the ‘slow-absorbable’ casein, ingestion of the ‘fast-absorbable’ whey protein resulted in higher peak values of amino acids, which were associated with higher peak values of the ‘satiety hormones’ glucagon-like peptide (GLP)-1 and cholecystokinin(Reference Hall, Millward and Long6). However, it cannot be excluded that other mechanisms contribute to these differences in satiety, as several alternative mechanisms have been suggested to underlie the satiating capacity of dietary proteins, including the thermic effects of protein consumption or the utilisation of amino acids for gluconeogenesis(Reference Veldhorst, Smeets and Soenen3).
The amino acid composition may partially influence the satiating properties of a dietary protein. In particular, the amino acid tryptophan (TRP) has gained specific interest, as it may act as a precursor for the neurotransmitter serotonin(Reference Wurtman and Wurtman7). Brain serotonin has been suggested to be involved in appetite regulation(Reference Leibowitz and Alexander8), which is supported by the anorexigenic effects of serotonergic drugs in human subjects(Reference Halford, Harrold and Boyland9, Reference Toornvliet, Pijl and Hopman10). Several dietary intervention studies have attempted to increase brain serotonergic activity, mainly through increasing central TRP availability(Reference Wurtman and Wurtman7, Reference Markus, Olivier and de Haan11, Reference Markus, Olivier and Panhuysen12). TRP transport to the brain is facilitated by the so-called L-transporter, which also facilitates the transport of the other large neutral amino acids (LNAA) valine, leucine, isoleucine, tyrosine and phenylalanine in a competitive manner(Reference Wurtman and Wurtman7, Reference Beulens, Bindels and de Graaf13). Therefore, brain TRP uptake may not only depend on plasma TRP concentrations, but also on the plasma ratio of TRP to the sum of these other LNAA(Reference Wurtman and Wurtman7, Reference Beulens, Bindels and de Graaf13).
As protein sources dramatically differ in their TRP content, they may distinctly influence plasma TRP concentrations or plasma TRP:LNAA ratio. The whey peptide, α-lactalbumin, contains relatively high levels of TRP, and has been shown to increase plasma TRP concentrations and plasma TRP:LNAA ratio when ingested alone(Reference Markus, Olivier and de Haan11, Reference Markus, Olivier and Panhuysen12) or as part of a meal(Reference Beulens, Bindels and de Graaf13). In contrast, collagen hydrolysate, or gelatin, contains very low levels of TRP and is even used as an experimental approach to induce central serotonin depletion in laboratory animals(Reference Lieben, van Oorsouw and Deutz14) as well as in human subjects(Reference Evers, Tillie and van der Veen15).
As these differences in TRP content may influence brain serotonin activity, and thereby appetite or energy intake, we investigated the differences in satiating properties of two different breakfasts, one containing α-lactalbumin and one containing gelatin as the only protein source. In order to be able to attribute possible differences to differences in TRP content, we included a third breakfast in which we added TRP (gelatin+TRP) to the gelatin breakfasts, resulting in a similar TRP content to the α-lactalbumin-containing breakfast.
Subjects and methods
Subjects
Thirty healthy male and female volunteers (aged 18–45 years) were recruited by advertisements in local newspapers and on notice boards at the university. They underwent a screening including medical history, measurement of body weight and height and cognitive restrained eating using a Dutch translation of the Three-Factor Eating Questionnaire(Reference Stunkard and Messick16, Reference Westerterp-Plantenga, Westerterp and Rubbens17). Twenty-four subjects (eleven male, thirteen female; aged 19–37 years; BMI 22–29 kg/m2) were selected on being in good health, non-smokers, non-vegetarian, not having cognitive dietary restraint (Three-Factor Eating Questionnaire factor 1 score < 9), not using medication apart from oral contraceptives and at most moderate alcohol users ( < ten alcoholic consumptions per week). Their mean age was 21 (sem 0·8) years and their BMI was 24·8 (sem 0·4) kg/m2 (Table 1). A written informed consent was obtained from these participants and the study protocol was approved by the Medical Ethical Committee of the Academic Hospital Maastricht.
Table 1 Subject characteristics at baseline
(Mean values with their standard errors)

TFEQ, Three-Factor Eating Questionnaire; TEE, total energy expenditure.
* The TFEQ measures three different factors of human eating behaviour.
† TEE is calculated by multiplying the BMR (calculated from the Harris–Benedict equation) by an activity index of 1·75.
Study design
The study was performed in a randomised, single-blind, within-subject design. Subjects reported to the laboratory for six times, separated by at least 3 d. Randomisation took place in two blocks of three test days: in both blocks, subjects received the three different breakfasts (containing either α-lactalbumin, gelatin or gelatin+TRP as the sole protein source) in random order divided over the three test days. They were instructed to fast from 22.00 hours the night before the test day.
On the first three test days, a permanent Teflon catheter was inserted into a dorsal vein of the hand, which was placed in a thermoregulated (60°C) box for arterialised venous blood sampling(Reference Abumrad, Rabin and Diamond18). At 08.30 hours, the protocol started with scoring hunger ratings. Breakfast was offered (t = 0 min) and completed within 20 min. With the first and the last bite taste perception was scored. Hunger ratings were completed at 20, 40, 60, 80, 100, 120, 180 and 240 min after breakfast. Blood samples were withdrawn immediately before breakfast, and at 20, 30, 40, 60, 80, 90, 100, 120, 180 and 240 min after breakfast for determination of plasma concentrations of amino acids, GLP-1, ghrelin, glucose and insulin.
In the second series of three test days, the protocol started at 08.30 hours with a breakfast as above. At t = 180 min, subjects were offered an ad libitum lunch. The lunch consisted of Turkish bread (400 g) with egg salad (400 g) with 13, 41 and 46 % energy from protein, carbohydrate and fat, respectively, and an energy density of 11·4 kJ/g. The bread was prepared in such a way that a thin, homogeneous layer of egg salad was placed between two thin layers of bread, so that bites were energetically homogeneous. Subjects were instructed to eat till they were comfortably full. Subjects were allowed to drink a maximum of three glasses of water spread over the entire test period.
Breakfast
Breakfast was offered as custard, produced by NIZO Food Research bv. (Ede, The Netherlands), and had a protein, carbohydrate and fat content of 10, 55 and 35 % energy, or 2·2, 12·4 and 3·7 % by weight, respectively. In these studies, three different custards were used, containing a single protein source, being either α-lactalbumin (BioPURE-Alphalactalbumin™; Davisco Foods International Inc., Eden Prairie, MN, USA), gelatin (Solugel LMC/3; PB Gelatins GmbH, Nienburg/Weser, Germany) or gelatin with TRP (Sigma-Aldrich, Steinheim, Germany) added to the level present in α-lactalbumin, as a single protein source. The custard contained tapioca starch (Farinex VA50T and Perfectamyl 3108; AVEBE, Veendam, The Netherlands) and sunflower-seed oil (Reddy; Vandemoortele Roosendaal NV, Roosendaal, The Netherlands) as the carbohydrate and fat sources, respectively. All three custards were citrus-vanilla flavoured (J.B. de Lange, Belfeld, The Netherlands). Extensive product development and use of a taste panel led to custards that did not differ significantly in colour, taste or viscosity. The breakfast contained 20 % of daily energy requirement, calculated as BMR, according to the equations of Harris and Benedict, multiplied by an activity index of 1·75(Reference Harris and Benedict19). The mean energy content of the breakfast (20 % of calculated daily total energy expenditure) was 2·54 (sem 0·06) MJ; this resulted in a mean intake of protein, carbohydrate and fat of 14·5 (sem 0·4), 81·7 (sem 2·1) and 24·4 (sem 0·6) g, respectively. The amino acid composition of the three different custards is presented in Table 2. TRP:LNAA ratios were 88 × 10− 3, 6 × 10− 3 and 280 × 10− 3 for the custards containing α-lactalbumin, gelatin, and gelatin with added TRP, respectively. All subjects received all three breakfasts in random order on the first series of three test days, as well as on the second series of three test days.
Table 2 Amino acid composition of the three breakfasts (μmol/100 g custard)
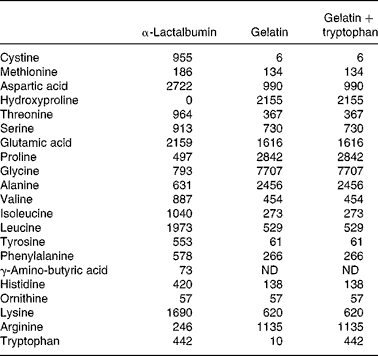
ND, not detected.
Measurements
Hunger ratings were obtained using a paper version of a 100 mm visual analogue scale, anchored with ‘not at all’ and ‘extremely’. Subjects were instructed to rate the hunger dimensions by marking the scale, using a pen, at the point that was most appropriate to their feeling at that time.
Energy intake during lunch was calculated by weighing the lunch before and after eating, and multiplying the difference of the weight by the energy value of the lunch as determined by the product labels (11·4 kJ/g).
Blood analysis
In both experiments, arterialised venous blood was collected in heparinised tubes (Becton Dickinson Vacutainer system; Plymouth, UK), which were kept on ice to minimise enzymic reactions. Plasma was obtained by centrifugation at 4°C for 10 min at 3000 g. Subsequently, 250 μl plasma was deproteinised with 20 mg dry sulfosalicylic acid for the analysis of plasma amino acid concentrations and enrichment. Each sample of plasma was frozen immediately in liquid N2 and stored at − 80°C, until analysis. All samples from one subject were run in the same assay. Amino acid concentrations were measured using an HPLC system (Pharmacia, Woerden, The Netherlands) as previously described (CV < 3 %)(Reference van Eijk, Rooyakkers and Deutz20). Urea concentrations were measured spectrophotometrically on a COBAS Mira S (Roche Diagnostica, Hoffmann-La Roche, Basel, Switzerland) (CV < 5 %). Concentrations of active ghrelin were measured by RIA (Linco Research, Inc., St Charles, MO, USA) (within-assay CV 6 %, between-assay CV 16 %). Concentrations of active GLP-1 were measured using ELISA (EGLP-35K; Linco Research Inc.) (within-assay CV 7 %, between-assay CV 8 %). Insulin concentrations were measured by an electrochemiluminescence immunoassay (Roche Diagnostica, Hoffmann-La Roche) (CV < 10 %). Glucose concentrations were measured using an enzymic assay (G6-PDH; Roche Diagnostica) (CV < 5 %).
Statistical analysis
Data are presented as mean changes from baseline with their standard errors, unless otherwise indicated. In order to perform regression analyses, for hunger scores, plasma concentrations of total and individual amino acids and TRP and for plasma TRP:LNAA ratios, the area under the curve (AUC) of changes from baseline was calculated using the trapezoidal method. A repeated-measures ANOVA, followed by Fisher's protected least significant difference (PLSD) post hoc test, was carried out to determine possible differences between the different types of protein. Bonferonni correction was used for multiple comparisons. Regression analysis was performed to determine the relationships between the AUC of hunger scores and the AUC from baseline of plasma concentrations of total amino acids and of plasma TRP:LNAA ratios for all three breakfasts. Stepwise multiple regression analysis was performed to determine the relationships between AUC of hunger scores and the AUC from baseline of all amino acids measured. A P value of < 0·05 was regarded as statistically significant. Statistical procedures were performed using StatView 5.0 (SAS Institute Inc., Cary, NC, USA).
Results
Following breakfast, hunger scores dropped immediately (Fig. 1). In the subsequent 240 min after ingestion, hunger scores gradually returned to pre-breakfast values. At t = 240 min hunger scores were significantly lower in the α-lactalbumin breakfast group compared with the gelatin breakfast groups, either with or without added TRP. The AUC of changes in hunger scores from baseline were − 10 031 (sem 883), − 7679 (sem 1072) and − 8288 (sem 1131) mm × min for the α-lactalbumin, gelatin and gelatin+TRP breakfasts, respectively (NS). Energy intake of an ad libitum lunch, provided 180 min after breakfast, was 2·65 (sem 0·28), 2·56 (sem 0·24) and 2·61 (sem 0·26) MJ for the α-lactalbumin, gelatin and gelatin+TRP breakfasts, respectively (NS).

Fig. 1 Changes in subjective ratings of hunger, as assessed by visual analogue scales (VAS), after ingestion of a custard based on α-lactalbumin (–□–), gelatin (–△–) or gelatin with tryptophan (TRP) addition (–○–). Values are means, with standard errors represented by vertical bars. * Mean value was significantly different from that following ingestion of the gelatin+TRP custard (P < 0·05). †† Mean value was significantly different from that following ingestion of the gelatin custard (P < 0·01).
Plasma total amino acid concentrations rose immediately after breakfast ingestion, reaching peak values between 60 and 80 min after breakfast (Fig. 2). After the α-lactalbumin breakfast, total amino acid concentrations returned to pre-breakfast values slightly quicker than after the gelatin breakfasts. Thus, at 180 min after breakfast, total amino acid concentrations were significantly lower after α-lactalbumin than after gelatin without added TRP; at 240 min after breakfast, total amino acid concentrations were significantly lower after α-lactalbumin than after gelatin with or without added TRP. The AUC of changes in plasma total amino acid concentrations from baseline were 95 058 (sem 8365), 122 788 (sem 19 326) and 112 577 (sem 10 462) μm × min for the α-lactalbumin, gelatin and gelatin+TRP breakfasts, respectively (NS). There was no significant correlation between the AUC of the hunger scores and the AUC of plasma total amino acid concentrations following ingestion of any of the breakfasts.

Fig. 2 Plasma total amino acid (AA) concentrations after ingestion of a custard based on α-lactalbumin (–□–), gelatin (–△–) or gelatin with tryptophan (TRP) addition (–○–). Values are means, with standard errors represented by vertical bars. * Mean value was significantly different from that following ingestion of the gelatin+TRP custard (P < 0·05). † Mean value was significantly different from that following ingestion of the gelatin custard (P < 0·05).
For each individual amino acid, peak plasma concentrations and AUC from baseline are shown in Table 3. Peak concentrations of asparagine, tyrosine, isoleucine, phenylalanine, leucine and lysine were higher, and peak concentrations of glycine, arginine and ornithine were lower after the α-lactalbumin breakfast than after the gelatin and gelatin with added TRP breakfasts. Peak plasma concentrations of TRP were higher after the ingestion of gelatin with added TRP than after gelatin alone, but not different from the peak values of TRP after ingestion of the α-lactalbumin-containing breakfast. The AUC from baseline of asparagine, histidine, threonine, tyrosine, isoleucine, phenylalanine, leucine and lysine were higher, and the AUC from baseline of serine, glycine, arginine, alanine, taurine and ornithine were lower after the α-lactalbumin breakfast than after the gelatin and gelatin with added TRP breakfasts. In addition, the AUC from baseline of valine was higher after α-lactalbumin than after gelatin with added TRP. The AUC of changes in plasma TRP concentrations from baseline was significantly lower after ingestion of the gelatin breakfast than after ingestion of the α-lactalbumin and gelatin with added TRP breakfast. Stepwise multiple regression analysis showed a significant correlation between the AUC of the hunger scores and the AUC from baseline of glutamic acid for the α-lactalbumin breakfast, and between the AUC of the hunger scores and the AUC from baseline of asparagine and taurine for the gelatin with added TRP breakfast. Thus, there was no significant correlation between the AUC of the hunger scores and the AUC of plasma TRP concentrations following ingestion of any of the breakfasts.
Table 3 Amino acid responses to ingestion of a custard containing either α-lactalbumin, gelatin or gelatin with added tryptophan as the single protein source‡
(Mean values with their standard errors)

AUC, area under the curve.
* Mean value was significantly different from that following ingestion of the α-lactalbumin custard (P < 0·05).
† Mean value was significantly different from that following ingestion of the gelatin custard (P < 0·05).
‡ Responses are shown as peak plasma concentrations and as AUC from baseline.
In the course of time, plasma levels of TRP were unaffected by the breakfast containing gelatin without added TRP (Fig. 3). Plasma TRP increased after ingestion of a breakfast containing either α-lactalbumin or gelatin with added TRP; however, from t = 20 to t = 60 min after breakfast, this increase was significantly larger after the α-lactalbumin breakfast than after the gelatin with added TRP breakfast, despite similar TRP levels in the breakfasts. From 80 to 180 min after breakfast, plasma TRP concentrations were higher after the breakfasts both with α-lactalbumin and with gelatin with added TRP than after the gelatin without TRP breakfast.

Fig. 3 Tryptophan (TRP) concentrations in plasma samples after ingestion of a custard based on α-lactalbumin (–□–), gelatin (–△–) or gelatin with tryptophan (TRP) addition (–○–). Values are means, with standard errors represented by vertical bars. *** Mean value was significantly different from that following ingestion of the gelatin+TRP custard (P < 0·001). ††† Mean value was significantly different from that following ingestion of the gelatin custard (P < 0·001). ‡‡‡ Mean value was significantly different from those following ingestion of the α-lactalbumin and gelatin+TRP custards (P < 0·001).
Of the different custards, the TRP:LNAA ratios were the highest in the gelatin+TRP custard, followed by the α-lactalbumin custard and the lowest in the gelatin custard (88 × 10− 3, 6 × 10− 3 and 280 × 10− 3 for the custards containing α-lactalbumin, gelatin, and gelatin with added TRP, respectively). In the plasma, the changes in TRP:LNAA ratios were not different between the conditions at any time point, with the exception of t = 100 min, where the change in TRP:LNAA was significantly lower after the ingestion of gelatin+TRP custard when compared with the gelatin or α-lactalbumin custards (Fig. 4). The AUC of changes in plasma TRP:LNAA ratios were 191 (sem 185), 2 (sem 25) and − 66 (sem 13) × min for the α-lactalbumin, gelatin and gelatin+TRP breakfasts, respectively (NS). There was no significant correlation between the AUC of the hunger scores and the AUC of plasma TRP:LNAA ratios following ingestion of any of the breakfasts.

Fig. 4 Changes in plasma tryptophan:large neutral amino acid (TRP:LNAA) ratio after ingestion of a custard based on α-lactalbumin (–□–), gelatin (–△–) or gelatin with TRP addition (–○–). Included in LNAA are valine, isoleucine, leucine, tyrosine and phenylalanine. Values are means, with standard errors represented by vertical bars. * Mean value was significantly different from that following ingestion of the gelatin+TRP custard (P < 0·05). † Mean value was significantly different from that following ingestion of the gelatin custard (P < 0·05).
Plasma GLP-1 concentrations increased immediately after ingestion of the custard, reaching peak levels at 30 min, which is followed by a gradual decline towards pre-breakfast levels (Fig. 5 (a)). There were no differences in plasma GLP-1 responses between any of the custards. Plasma ghrelin concentrations decreased after ingestion of the custard, reaching lowest levels between 40 and 60 min, which is followed by a gradual return towards, or even slightly above, pre-breakfast levels (Fig. 5 (b)). There were no differences in plasma ghrelin responses between any of the custards.

Fig. 5 Plasma glucagon-like peptide (GLP)-1 (a) and ghrelin (b) concentrations after ingestion of a custard based on α-lactalbumin (–□–), gelatin (–△–) or gelatin with tryptophan addition (–○–). Values are means, with standard errors represented by vertical bars.
Plasma concentrations of glucose increased after ingestion of the custard, with peak values at 40 min, after which glucose concentrations gradually declined, reaching pre-breakfast levels between 120 and 180 min after meal intake. At t = 40 min, glucose concentrations after ingestion of the α-lactalbumin-containing breakfast (7·0 (sem 0·2) mmol/l) were significantly lower than after ingestion of the gelatin (7·8 (sem 0·2) mmol/l) or after the gelatin+TRP-containing breakfast (7·5 (sem 0·3) mmol/l) breakfast. At other time points, there were no differences in plasma glucose concentrations between any of the custards. Plasma insulin concentrations also rose after ingestion of the custard, reaching peak values at 40 min. At t = 180 min, plasma insulin levels had returned to pre-breakfast levels for all three custards. There were no differences in plasma insulin responses between any of the custards.
Discussion
The present study shows that ingestion of a breakfast containing α-lactalbumin as the only protein source results in a more prolonged suppression of hunger than a breakfast containing gelatin, although the hunger-suppressive effect was insufficient to decrease energy intake during a subsequent lunch. The proteins were compared in a normal-sized meal with a normal macronutrient composition; although a higher protein load may have resulted in a more pronounced suppression of hunger and a decrease in energy intake, this may not reflect a physiological situation. Also, it cannot be excluded that the lack of an effect on energy intake is due to the timing of the lunch (180 min after breakfast), as the differences in hunger ratings did not reach statistical significance until 240 min after breakfast. There are only a few human studies that compare the satiating properties of different protein sources(Reference Veldhorst, Smeets and Soenen3, Reference Hall, Millward and Long6, Reference Borzoei, Neovius and Barkeling21–Reference Uhe, Collier and O'Dea25).
The faster recurrence of hunger after a gelatin-containing breakfast was not due to its low TRP content, as addition of TRP did not affect subjective ratings of hunger or satiety. It is therefore unlikely that the observed differences in hunger scores are related to the TRP content or the TRP:LNAA ratio. The rise in plasma TRP concentrations after the ingestion of α-lactalbumin occurred faster than after the ingestion of gelatin with added TRP, but those differences disappeared 100 min after breakfast. From that time on, plasma TRP responses were significantly lower after gelatin ingestions than after gelatin with added TRP, even though hunger ratings were similar. Nevertheless, even though the plasma responses of TRP were not correlated with hunger scores in any of the breakfasts offered, it cannot be excluded that the higher TRP levels in the first hour may have contributed to the lower perception of hunger 240 min after the α-lactalbumin-containing breakfast.
Still, in view of the competitive fashion of TRP and LNAA transport across the blood–brain barrier, TRP:LNAA ratios, rather than TRP concentrations, are suggested to determine brain TRP uptake. The changes in TRP:LNAA ratios were similar between the three breakfasts, with the exception of t = 100 min, where the TRP:LNAA ratio after the ingestion of gelatin with added TRP was significantly lower when compared with the other two breakfasts. Since the hunger responses were similar after the ingestion of gelatin with added TRP and after the ingestion of gelatin without added TRP, despite these differences in the TRP:LNAA ratio, and since hunger ratings were lower in α-lactalbumin v. gelatin without added TRP, despite similar TRP:LNAA ratios, the TRP:LNAA ratios provide no straightforward explanation for the observed differences in hunger ratings. Accordingly, hunger ratings did not correlate with plasma TRP concentrations or plasma TRP:LNAA ratios for any of the breakfasts.
Remarkably, the TRP:LNAA ratios in the different breakfast are not reflected by plasma TRP:LNAA ratios. As the plasma TRP concentrations largely reflect the TRP concentrations in the breakfasts (high in α-lactalbumin and gelatin with added TRP, low in gelatin without added TRP), LNAA responses should account for the incongruence between TRP:LNAA ratios in plasma and breakfast. In this respect, insulin may pay a role, as insulin selectively stimulates the uptake of LNAA(Reference Wurtman and Wurtman7, Reference Pan, Mauron and Glaeser26). However, as insulin concentrations were similar in all three groups at all time points, other mechanisms, such as amino acid metabolism in intestinal cells, must be involved.
As the differences in hunger ratings between the three breakfasts cannot straightforwardly be explained by changes in TRP concentrations or the TRP:LNAA ratios in the custard or plasma, other mechanisms must be involved. Unfortunately, these mechanisms are not elucidated by the present study. Already in 1956, Mellinkoff proposed an aminostatic regulation of food intake, implicating that increased plasma amino acid concentrations provide a satiety signal(Reference Mellinkoff, Frankland and Boyle27). Still, such a mechanism cannot explain the observed differences in subjective hunger ratings: total plasma amino acid concentrations were similar for all three breakfasts, with the exception of the period between 180 and 240 min after breakfast, when total plasma amino acid concentrations were the lowest after α-lactalbumin ingestion – which was associated with lower hunger ratings. Other individual amino acids, besides TRP, have also been suggested to be involved in the regulation of appetite and food intake. The responses of the individual amino acids to the α-lactalbumin- and gelatin-containing breakfasts showed marked differences, largely reflecting differences in amino acid composition. For instance, the leucine responses (both as peak values and AUC from baseline) were higher after the α-lactalbumin-containing breakfast than after the gelatin-containing breakfasts. In fasted rats, leucine has been shown to decrease food intake, presumably by hypothalamic activation of the mammalian target of rapamycin(Reference Cota, Proulx and Smith28). This may suggest that the increased leucine response may be involved in the prolonged suppression of hunger after α-lactalbumin ingestion. However, our studies did not reveal a significant relationship between the hunger scores and the plasma responses of leucine (as AUC) in any of the breakfasts provided. Therefore, the role of leucine (and other individual amino acids) in the observed difference in hunger perception between the different protein sources remains to be elucidated.
Plasma responses of GLP-1 and ghrelin, a ‘satiety’ and ‘hunger’ hormone, respectively, which are implicated in playing a role in the regulation of appetite and energy intake(Reference Dhillo and Bloom29), were similar for all three breakfasts – indicating that these hormones are not involved in the differences in hunger rates. In addition, recent studies showed that the increased satiety after a high-protein meal compared with a non-protein meal are not mediated by changes in circulating ghrelin and/or GLP-1 concentrations(Reference Lejeune, Westerterp and Adam1, Reference Smeets, Soenen and Luscombe-Marsh2, Reference Moran, Luscombe-Marsh and Noakes30). Therefore, the satiating properties of dietary protein are likely to be mediated by a more complex set of mechanisms, and this may even be protein specific, as has recently been suggested(Reference Veldhorst, Smeets and Soenen3).
All in all, the present study shows that a breakfast containing α-lactalbumin as the only protein source results in a more prolonged suppression of hunger than a breakfast containing gelatin. Although the mechanism behind this difference has not been elucidated, TRP concentrations and TRP:LNAA ratios in the breakfasts and the circulation, as well as total amino acid, GLP-1 and ghrelin responses, do not seem to be involved.
Acknowledgements
A. G. N., A. H-W., M. A. B. V., K. R. W., M. P. K. J. E., N. E. P. D. and M. S. W-P. designed the study. M. A. B. V. and A. H-W. collected and analysed the data. A. G. N. wrote the manuscript and A. H-W., M. A. B. V., K. R. W., M. P. K. J. E., N. E. P. D. and M. S. W-P. contributed to interpretation of the data and reviewed the manuscript. The study was executed under supervision of A. G. N., K. R. W. and M. S. W-P.
None of the authors had a personal or financial conflict of interest.