Obesity is a growing problem in both companion animal and human populations in many countries around the world. Diets with differing macronutrient compositions that increase satiety have been evaluated in the past, but data evaluating specific sources of macronutrients are more limited. It has been well documented that satiety can be affected by the macronutrient composition of the diet(Reference Holt, Brand Miller and Petocz1). Proteins, specifically, are noted to be the most satiating; however, dietary source may influence satiety. Satiety is regulated by the central nervous system, including long-term energy utilisation and body stores (multiple meal intake), and short-term energy intake and utilisation (meal intake)(Reference Cummings and Overduin2), allowing multiple systems to be targeted when trying to influence satiety. Orexigenic modifiers increase food intake and decrease energy expenditure (for example, ghrelin), while anorexigenic modifiers decrease food intake and increase energy expenditure (for example, glucagon-like peptide-1 (GLP-1), insulin)(Reference Delzenne, Blundell and Brouns3). Finding protein sources that can more effectively decrease orexigenic compounds and increase anorexigenic compounds may help decrease food intake.
Of the satiety-related hormones, some commonly analysed include an orexigenic compound, ghrelin, and GLP-1. Ghrelin, produced by gastric X/A-like cells, increases during fasting, leading to increased food intake. High-protein diets, when compared with high-carbohydrate diets, have been reported to decrease postprandial ghrelin concentrations, but with a more moderate and prolonged effect on ghrelin suppression(Reference Tannous dit El Khoury, Obeid and Azar4). GLP-1 is thought to regulate food intake by playing a role in the ileal brake phenomenon(Reference Wettergren, Schjoldager and Mortensen5) and through a direct effect on the hypothalamus(Reference Turton, O'Shea and Gunn6, Reference Tang-Christensen, Larsen and Göke7), leading to a reduced food intake or cessation of a meal, thereby decreasing the rate of nutrient influx into the blood(Reference Strader and Woods8). Circulating GLP-1 concentrations are influenced by macronutrient concentration; therefore, it is possible that fish substrates may lead to increased circulating GLP-1 and to a reduction in food intake.
Circulating plasma amino acids (AA) also have been implicated in the satiety response to a meal, including insulinogenic AA. The theory of AA influencing food intake was described by Mellinkoff(Reference Mellinkoff9), and suggests that the AA profile of food and the modifications this profile undergoes based on the needs of the intestine and liver may regulate its role in satiety. It also has been suggested that the tryptophan:large neutral AA (LNAA) ratio after a meal can lead to changes in food intake; specifically, a reduction in this ratio may increase food intake.
High-protein meals fed to human subjects have been shown to prolong satiation as compared with high-carbohydrate meals(Reference Barkeling, Rossner and Bjorvell10, Reference Johnson and Vickers11), but less research exists on the effect of the dietary source of protein on satiety. Holt et al. (Reference Holt, Brand Miller and Petocz1) developed a satiety index of common foods in humans and noted that fish was the most satiating protein source (compared with beef steak, baked beans, eggs, cheese, lentils and a white bread standard). A reduction in food intake following a fish pre-load or meal compared with beef and/or chicken has been noted in human subjects(Reference Uhe, Collier and O'Dea12, Reference Borzoei, Neovius and Barkeling13). These authors proposed that this prolonged satiety may be related to the fact that fish protein AA took longer to reach peak plasma concentrations compared with a beef or chicken meal, as well as increased plasma taurine and methionine concentrations(Reference Uhe, Collier and O'Dea12). While these effects have been noted, the mechanism of this satiety effect is unknown.
Dogs are an appropriate model to assess satiety response of humans due to similarities regarding select feeding behaviours. Dogs, like humans, will continue to consume a highly palatable diet beyond their energy requirements. Therefore, the food intake response following a pre-meal may simulate a response by healthy adult humans. Also, it was our decision to use the dog as a model for humans due to the nature of our substrates (dried, ground meat), which would not be palatable to human subjects. Finally by using the dog as a model for humans, we were able to better control other factors that influence hormone response and voluntary food intake, including taste preference, body weight and exercise pattern. Finally, these data provide information about canine diets, as influencing satiety in dogs to control body weight is also of importance.
The objective of the present study was to determine the effect of beef, chicken, pork or fish protein pre-meals on postprandial satiety response, plasma AA concentrations and 24 h food intake in healthy, adult dogs. We hypothesised that fish protein would be more satiating compared with mammalian and avian sources.
Materials and methods
Animals, diets and substrates
A total of ten purpose-bred, intact female hounds (Butler Farms, Clyde, NY, USA) with hound bloodlines were utilised in the present experiment (aged 4·5 ± 0·1 years; 22·1 ± 2·0 kg body weight). Dogs were individually housed (2·3 m × 1·1 m pens) in a climate-controlled room in the Edward R. Madigan Laboratory on the University of Illinois campus. Pens allowed for nose − nose contact between dogs in adjacent runs and visual contact with all dogs in the room. A 16 h light–8 h dark cycle was used. All dogs were fed the diets to maintain body weight throughout the study, but were allowed 2 × the metabolisable energy (ME) requirement during the protein postprandial tests. ME was determined based on previous feeding of the diet to maintain the body weight of each animal individually. All animal procedures were approved by the University of Illinois Institutional Animal Care and Use Committee (IACUC) before animal experimentation.
Dogs were fed an experimental diet throughout the experiment. The diet contained poultry by-product meal, maize and brewer's rice as the main ingredients and was formulated to contain 30 % crude protein and 20 % fat. Food intake was determined daily. We utilised five substrates in the present study including pork loin, beef loin, chicken breast, salmon fillet and pollock fillet. All sources were previously utilised as the main protein source in diets fed to dogs to determine nutrient digestibility(Reference Faber, Bechtel and Hernot14). The composition of these substrates also was determined previously by Faber et al. (Reference Faber, Bechtel and Hernot14). Briefly, all substrates were dried at 71°C for 6 h. They were then ground to pass a 2 mm screen and were stored at 4°C until used.
Experimental design
The present study was conducted as replicated 5 × 5 Latin squares in a completely randomised design. Testing was conducted at the same time each day with 2 or 3 d between tests. Dogs were food-restricted for a minimum of 12 h before collection days. Dogs were fed an amount of substrate required to provide 100 g protein in 200 ml water. Water was added to the substrates to ease consumption of the dried meat material. Blood was collected before feeding the substrate (time 0), and at 5, 15, 30, 60, 90 and 120 min postprandially. Dogs were fed 2 × the ME requirement 3 h following the feeding of the protein substrate to determine a satiety response. Food was weighed at 30, 60, 180 and 1440 min after food presentation.
Chemical analyses
Postprandial blood samples were analysed for plasma glucose, serum insulin, plasma AA, serum total ghrelin and plasma active GLP-1. Blood was collected by jugular venepuncture into 10 ml syringes and immediately transferred to appropriate vacutainer tubes. Blood used to measure plasma AA and glucose was transferred into vacutainer tubes containing lithium heparin to prevent clotting and centrifuged at 2000 g at 4°C for 15 min. Blood (1 ml) was transferred to a vacutainer tube containing an EDTA anti-coagulant and 10 μl dipeptidyl peptidase IV, according to the manufacturer's instructions, and centrifuged at 1000 g at 4°C for 10 min for measurement of active GLP-1. All other blood was placed in tubes containing no anti-coagulant where it was allowed to clot, and then centrifuged at 1300 g for 10 min. After centrifugation, the supernatant fraction was removed and was either immediately analysed (glucose) or stored (insulin and plasma AA, − 20°C; GLP-1 and total ghrelin, − 80°C) until analysed. Plasma glucose was analysed using the glucose peroxidase method (Sigma-Aldrich, St Louis, MO, USA). Serum was analysed for insulin using a Rat Insulin Enzyme Immunoassy kit (Cayman Chemical, Ann Arbor, MI, USA) noted to work in dogs(Reference Knapp, Parsons and Swanson15). Following a 10-fold dilution, total serum ghrelin was determined using a total ghrelin (rat, mouse) enzyme immunoassay (EIA) kit (Phoenix Pharmaceuticals, Inc., Burlingame, CA, USA) according to Vester et al. (Reference Vester, Liu and Keel16) and noted to work in dogs(Reference Lubbs, Vester Boler and Ridge17). Plasma active GLP-1 concentrations were determined using the Glucagon-Like Peptide-1 (Active) ELISA kit (Millipore, St Charles, MO, USA) noted to work in dogs(Reference Lubbs, Vester Boler and Ridge17). Plasma GLP-1 concentrations were determined using a linear–linear spline fit curve. Plasma AA concentrations were determined using ion-exchange analysis with post-column ninhydrin detection using lithium-based eluants after treatment with SERAPREP (Pickering Laboratories, Inc., Mountain View, CA, USA).
Statistical analysis
For all repeated data, incremental changes from baseline were first determined. Data then were analysed as repeated measures using the Mixed procedure of SAS (SAS Institute Inc., Cary, NC, USA). The main effects of protein pre-meal and time were tested and the interaction evaluated if significant. Dog and period were included as random effects in the model. All baseline (0 h samples) data were analysed using the Mixed procedure of SAS with the main effect of protein pre-meal tested. Dog and period again were included in the model as random effects. A P value of 0·05 was considered significant and P < 0·10 was considered a trend.
Results
Food intake
Food intake (Table 1) did not differ (P = 0·56) among pre-meals, when dogs were fed 100 g protein from each substrate, but incremental change in food intake decreased (P < 0·01) over time, as would be expected.
Table 1 Food intake values at 30, 60, 180 and 1440 min after dogs were fed mammalian, avian or fish protein sources containing 100 g protein
(Mean values for nine or ten dogs)

Plasma glucose and serum insulin
Baseline plasma glucose concentrations did not differ among diets (P = 0·73) and averaged 5·8 (sem 0·14) mmol/l. Overall incremental change in blood glucose (Fig. 1(a)) was greater (P = 0·03) when dogs consumed pork and beef compared with chicken, and was lower when dogs consumed pollock compared with pork. Blood glucose generally decreased (P < 0·01) over time. At 5 min postprandially, glucose was lowest (P = 0·03) in dogs fed the chicken pre-meal compared with pork, beef and pollock. Baseline serum insulin concentrations did not differ among diets (P = 0·87) and averaged 107·2 (sem 15·7) mmol/l. Incremental changes in insulin (Fig. 1(b)) were lower (P = 0·05) when dogs consumed pollock, pork and beef pre-meals compared with salmon. Insulin increased over time (P < 0·01) and had two peaks (5 and 30 min postprandially). At 30 min postprandially, insulin was greater (P = 0·02) with the salmon pre-meal compared with pollock, beef or pork. There were no differences in incremental area under the curve (AUC) for glucose or insulin.

Fig. 1 (a) Incremental changes in glucose concentrations in dogs consuming mammalian (●, beef; ▲, pork), avian (■, chicken) or fish (▾, pollock; ♦, salmon) protein sources. Values are means, with pooled standard errors represented by vertical bars. There were treatment (P = 0·03) and time (P < 0·0001) effects but no treatment × time effect (P = 0·97). * Mean value was significantly lower compared with those for pork, beef and pollock (P < 0·05). (b) Incremental changes in insulin concentrations in dogs consuming mammalian (●, beef; ▾, pork), avian (■, chicken) or fish (▲, pollock; ♦, salmon) protein sources. Values are means, with pooled standard errors represented by vertical bars. There were treatment (P = 0·05) and time (P < 0·0001) effects but no treatment × time effect (P = 0·11). * Mean value of chicken was significantly lower compared with those for pollock, beef and pork (P < 0·05).
Serum total ghrelin and plasma glucagon-like peptide-1
Baseline serum total ghrelin concentrations did not differ among diets (P = 0·62) and averaged 1·9 (sem 0·35) ng/ml. Ghrelin incremental change (Fig. 2(a)) did not differ due to diet (P = 0·86), but decreased (P < 0·01) over time. Ghrelin was lower (P = 0·03) by 60 min postprandially, regardless of the protein pre-meal. Baseline plasma GLP-1 concentrations did not differ among diets (P = 0·66) and averaged 6·1 (sem 2·72) pm. Incremental changes in GLP-1 (Fig. 2(b)) were lower (P = 0·04) when dogs consumed the pork, salmon and chicken pre-meals compared with beef. Overall, GLP-1 increased over time (P < 0·01). There were no differences in incremental AUC for ghrelin or GLP-1.

Fig. 2 (a) Incremental changes in total ghrelin concentrations in dogs after consuming mammalian (●, beef; ▾, pork), avian (■, chicken) or fish (▲, pollock; ♦, salmon) protein sources. Values are means, with pooled standard errors represented by vertical bars. There was a time effect (P < 0·0001), but no effects of treatment (P = 0·85) or treatment × time (P = 0·96). (b) Incremental changes in glucagon-like peptide-1 (GLP-1) concentrations in dogs after consuming mammalian (●, beef; ▲, pork), avian (■, chicken) or fish (▾, pollock; ♦, salmon) protein sources. Values are means, with pooled standard errors represented by vertical bars. There was a time effect (P < 0·0001), but no effects of treatment (P = 0·32) or treatment × time (P = 0·93). † Mean value of beef and pollock tended to be significantly higher compared with those for pork or salmon (P < 0·10).
Plasma amino acids
Incremental changes from baseline of plasma AA are presented in Fig. 3(a)–(e), Fig. 4(a)–(e) and Supplemental Fig. 1 (available online at http://www.journals.cambridge.org/bjn). Insulin-stimulating AA leucine (P = 0·003), isoleucine (P < 0·01) and histidine (P < 0·01) incremental changes from baseline were greatest when dogs consumed the chicken pre-meal compared with pork, beef or pollock. The plasma glutamine incremental change from baseline decreased (P < 0·01) when dogs consumed pork compared with all other pre-meals. There was an interaction between pre-meal and time (P < 0·01) in plasma histidine incremental change, with the chicken pre-meal increasing more (P = 0·001) after 60, 90 and 120 min compared with all other pre-meals.

Fig. 3 Incremental changes in insulin-stimulating plasma amino acids leucine (a), asparagine (b), isoleucine (c), glutamine (d) and histidine (e) concentrations in dogs after consuming mammalian (●, beef; ▾, pork), avian (■, chicken) or fish (▲, pollock; ♦, salmon) protein sources. Values are means, with pooled standard errors represented by vertical bars. * P < 0·05 (for contrasts, see Plasma amino acids section in the Results).

Fig. 4 Incremental changes in plasma amino acids valine (a), methionine (b) and taurine (c) concentrations, large neutral amino acids (LNAA) concentration (d) and tryptophan:LNAA ratio (e) in dogs after consuming mammalian (●, beef; ▾, pork), avian (■, chicken) or fish (▲, pollock; ♦, salmon) protein sources. Values are means, with pooled standard errors represented by vertical bars. * P < 0·05, † P < 0·10 (for contrasts, see Plasma amino acids section in the Results).
Valine (P < 0·01) and LNAA (P = 0·005) concentrations were greatest when dogs consumed the chicken pre-meal compared with pork, beef or pollock. Beef and pork pre-meals led to a lower (P < 0·01) overall plasma methionine change from baseline. Plasma taurine had a time × pre-meal interaction (P < 0·01) where, at 60 min postprandially, the salmon pre-meal led to the greatest (P < 0·01) taurine concentration compared with all other pre-meals, and the response following chicken was greater (P < 0·01) than after the beef or pork pre-meals. The tryptophan:LNAA ratio tended (P = 0·08) to be greater when dogs consumed pork, salmon and pollock pre-meals compared with beef, and this ratio following pork tended (P = 0·10) to be greater than after the chicken pre-meal.
Total AA (P < 0·01) incremental changes from baseline were greatest when dogs consumed the chicken pre-meal compared with pork, beef or pollock. Most AA, except glutamine (P = 0·93), changed over time, with most increased (P < 0·01) from baseline by 60 min. Leucine, asparagine, valine, histidine and LNAA concentrations increased (P < 0·01) by 30 min postprandially, while others were increased (P < 0·01) by 60 min postprandially. Plasma methionine tended (P = 0·09) to differ due to diet and time, with pollock increasing (P < 0·01) from 60 to 90 min postprandially, while all other pre-meals plateaued after 60 min.
Leucine (P = 0·09), isoleucine (P = 0·006), histidine (P = 0·001) and valine (P = 0·02) incremental AUC (Table 2) were greater when dogs consumed the chicken pre-meal. Methionine incremental AUC tended to be greater (P = 0·08) when dogs consumed chicken, pollock and salmon pre-meals. Taurine incremental AUC was greater (P = 0·003) when dogs consumed the salmon and pollock pre-meals. Incremental AUC was not affected by pre-meal for hydroxyproline, threonine, serine, asparagine, proline, glycine, alanine, citrulline, tryptophan, tyrosine, lysine, arginine, LNAA, tryptophan:LNAA or total (data not presented). All other changes in AA concentrations are provided in the online supporting material (available at http://www.journals.cambridge.org/bjn).
Table 2 Incremental area under the curve values for plasma amino acids in dogs fed mammalian, avian or fish protein sources
(Mean values with pooled standard errors for nine or ten dogs)
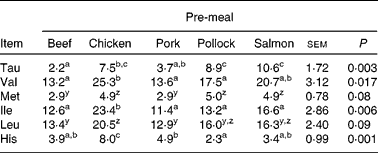
a,b,c Mean values within a row with unlike superscript letters were significantly different (P < 0·05).
y,z Mean values within a row with unlike superscript letters tended to be significantly different (P < 0·10).
Discussion
The present study aimed to determine if differing protein sources affected satiety hormones, plasma AA associated with satiety and voluntary food intake. All dogs were provided pre-meals consisting of 100 g of each protein source; therefore, amount provided, fat concentration and other nutrient concentrations differed (Table 3). This was done to allow for accurate interpretation of changes in plasma AA concentrations. It was recognised that providing pre-meals as liquid slurries may have an impact on the retention time in the stomach, thereby influencing the satiety response. While the present study provides important data about the differences among satiety responses due to animal protein source, a voluntary food intake response was not demonstrated. The strength of these data is that all outside influences were controlled, which may indicate that there are limited differences in satiety among animal protein sources. Including a plant-based protein source may have aided data interpretation.
Table 3 Substrate, fat, fibre, gross energy and amino acid (AA) intake by dogs consuming mammalian, avian or fish protein sources (per 100 g protein)

While we found no differences in voluntary food intake, fish proteins have been noted to be more satiating than beef(Reference Uhe, Collier and O'Dea12, Reference Borzoei, Neovius and Barkeling13) and chicken(Reference Uhe, Collier and O'Dea12) in human studies. Uhe et al. (Reference Uhe, Collier and O'Dea12) noted that 50 g chicken or beef protein were equally less satiating compared with fish (Mustelus antarticus) protein using subjective hunger and fullness scores, but subsequent food intake was not different. Borzoei et al. (Reference Borzoei, Neovius and Barkeling13) noted no changes in subjective satiety scores between beef and fish protein-based lunches, and there was an 11 % reduction in energy intake at the evening meal when fish protein was consumed. It is not completely unexpected that we were unable to reduce food intake in dogs after the consumption of a protein pre-meal. Again, many dogs, like humans, will over-consume when allowed ad libitum access to food. It should also be noted that these dogs are routinely limit-fed to maintain a healthy body weight and, therefore, the novelty of having copious amounts of food may have influenced results. Feeding to maintain body weight, however, was equally important, as circulating levels and the effectiveness of some satiety hormones can be affected by body fat mass. The weight of food provided is also known to correlate with satiety response(Reference Holt, Brand Miller and Petocz1), which may have led to more variation in our data.
Glucose and insulin responses in the present study were similar to those in previous reports of glucose and insulin changes following a protein dose(Reference Uhe, Collier and O'Dea12, Reference Borzoei, Neovius and Barkeling13) or high-protein mixed meal(Reference Schmid, Schusdziarra and Schulte-Frohlinde18, Reference Lang, Bellisle and Oppert19) fed to human subjects. Soucy & LeBlanc(Reference Soucy and LeBlanc20) noted that in adult male subjects at 90 min after a 50 g protein pre-load from either beef or cod, insulin was greater compared with baseline and this difference was greater after the beef pre-meal. In the present study, we noted differences only at 30 min postprandially, with pollock (a white fish cod) having the lowest insulin value; however, this trend was not noted at 90 min as was noted by Soucy & LeBlanc(Reference Soucy and LeBlanc20). Unlike the results of the present study, Pal & Ellis(Reference Pal and Ellis21) noted that insulin was greater at 30 min postprandially when adult male human subjects consumed tuna compared with turkey as fed in a chocolate-flavoured liquid pre-meal, and a greater drop occurred in glucose at 60 min. The subjects also had lower voluntary food intake and a greater feeling of fullness after the tuna pre-meal (50 g) compared with turkey. This may indicate that individual meat sources should be evaluated to determine potential impacts on satiety, as all types of fish did not behave similarly.
Total ghrelin responses in the present study were similar to those of Bowen et al. (Reference Bowen, Noakes and Clifton22) and Diepvens et al. (Reference Diepvens, Häberer and Westerterp-Plantenga23), where there was no effect of protein substrate on ghrelin concentrations throughout most of the postprandial test of adult human subjects. Diepvens et al. (Reference Diepvens, Häberer and Westerterp-Plantenga23) noted that milk protein and whey protein did not have the ghrelin-suppression capabilities of pea protein hydrolysate or whey protein+pea protein hydrolysate, but this was only noted at 120 min postprandially. To our knowledge, no published literature currently evaluates the effects of different animal protein sources on ghrelin. Total ghrelin remained suppressed after 120 min for all pre-meals, but it is unclear how physiologically relevant this small change was or how long this suppression lasts, as most dogs readily consumed two times their ME requirement within 3 h of the pre-meal. Ghrelin concentration decreases when subjects consume a high-protein meal compared with a high-carbohydrate meal(Reference Blom, Lluch and Stafleu24); however, some authors have argued that decreased ghrelin is unrelated to the satiating potential of high-protein meals(Reference Moran, Luscombe-Marsh and Noakes25–Reference Smeets, Soenen and Luscombe-Marsh27). While a high-protein v. lower-protein meal may be able to suppress ghrelin, it does not appear to be affected by animal protein source.
Overall, the present results indicate that protein led to increases in GLP-1 after the consumption of a high-animal protein pre-meal, which is also noted in human subjects. The present results were similar to those of Diepvens et al. (Reference Diepvens, Häberer and Westerterp-Plantenga23); however, they noted that GLP-1 concentrations returned to baseline within 120 min. In the present study, GLP-1 concentrations remained elevated after 120 min. This prolonged effect may be due to the differences in protein dose between the two studies. Diepvens et al. (Reference Diepvens, Häberer and Westerterp-Plantenga23) provided human subjects 15 g of protein, while in the present study in dogs, 100 g of protein were consumed. More pronounced changes were noted by Bowen et al. (Reference Bowen, Noakes and Clifton22) when human subjects were fed 50 g of protein from soya, whey, gluten or glucose. This difference may be attributable to the fact that the liquid pre-load fed to subjects by Bowen et al. (Reference Bowen, Noakes and Clifton22) contained carbohydrates. It is noted that GLP-1 is increased after a high-protein meal(Reference Johnson and Vickers11, Reference Lejeune, Westerterp and Adam26), and it is suggested that carbohydrates need to be included with the high-protein meal to stimulate the release of GLP-1(Reference Veldhorst, Smeets and Soenen28). Because we had no carbohydrates in our pre-meal, GLP-1 may not have changed as drastically as it would have done if a complete meal had been consumed.
Changes in plasma AA concentrations are affected by the AA content of the meal ingested, rate of digestion and absorption, and metabolism by the enterocyte and liver(Reference Hall, Millward and Long29). Several AA have been suggested to be insulinogenic and/or satiating. The five AA with the most insulin-stimulating activity in dogs include tryptophan, leucine, asparagine, isoleucine and glutamine(Reference Rocha, Faloona and Unger30). Leucine, asparagine, isoleucine and glutamine were all affected by the pre-meal and were greatest when dogs consumed the chicken and salmon pre-meals, which correspond with the insulin responses to these pre-meals. Tryptophan was not affected by diet in the present study. In human subjects, arginine is a noted insulinogenic AA(Reference Floyd, Fajans and Conn31); however, only essential AA were tested by Rocha et al. (Reference Rocha, Faloona and Unger30), and all were noted to have some degree of insulinogenic activity, with histidine having the lowest capacity. Plasma arginine was not affected by the protein substrate in the present study.
Soucy & LeBlanc(Reference Soucy and LeBlanc20) noted differences between beef and fish plasma AA concentrations 90 min postprandially for arginine, histidine and lysine in adult males. Of these AA, only histidine was affected in the present study. Soucy and LeBlanc(Reference Soucy and LeBlanc20) noted increased histidine concentrations following beef compared with a fish pre-meal. This is similar to results of the present study, as the two fish sources led to lower overall histidine incremental changes. This is probably due to the lower histidine concentrations in the fish pre-meals compared with the mammalian and avian sources in which similar compositional differences were noted by Soucy & LeBlanc(Reference Soucy and LeBlanc20). Although histidine was not identified as a major insulinogenic AA in dogs, other researchers have noted that carnosine (composed of histidine and β-alanine) or histidine increased plasma insulin(Reference Leblanc and Soucy32). Histidine, however, is not known to play a role in satiety.
Hall et al. (Reference Hall, Millward and Long29) noted increases in total plasma AA of human subjects fed a liquid pre-load of 48 g casein or whey protein. Whey protein led to a larger increase in total plasma AA, with the most dramatic difference between the two protein sources occurring 80 min postprandially. Whey also was noted to lead to greater concentrations of anorexigenic hormones, as well as a greater feeling of satiety by respondents. The authors noted that phenylalanine and tryptophan, AA associated with increased satiety(Reference Muurahainen, Kissileff and Derogatis33), were not affected in their study. This was similar to the present results showing that protein substrate did not affect phenylalanine or tryptophan, but both increased over time by 60 min postprandially.
Uhe et al. (Reference Uhe, Collier and O'Dea12) noted changes in plasma AA profiles after the consumption of 50 g protein from beef (topside steak), chicken (breast) or fish (Mustelus antarcticus) by adult male subjects. The authors noted increases in plasma taurine (45, 60, 90 min postprandially) and methionine (90, 150, 180 min postprandially) when subjects consumed the fish pre-meal. The change in tryptophan:LNAA ratio also was greater (45 and 60 min postprandially) after consumption of the fish pre-meal(Reference Uhe, Collier and O'Dea12). The authors attributed the increase in satiety scores noted in their study (fish being more satiating) to the tryptophan:LNAA ratio, suggesting an increase in serotonin activity. Normally, after ingestion of proteins, the tryptophan (a precursor for serotonin):LNAA ratio decreases, thereby limiting the amount of serotonin able to pass the blood–brain barrier. Therefore, increasing this ratio may lead to increased satiety by allowing more tryptophan to pass through this barrier. In the present study, a trend was noted for a greater tryptophan:LNAA ratio when dogs consumed pork, pollock or salmon meal, but was highest with a pork pre-meal. As noted previously, there were no changes in subsequent food intake after the pre-load meal; it is unclear if this greater ratio influenced feelings of satiety in the dog.
Satiety has been reported to be affected in high- v. normal-soyaprotein diets due to insulin and plasma taurine concentrations in human subjects(Reference Veldhorst, Nieuwenhuizen and Hochstenbach-Waelen34). The authors noted a positive relationship of taurine concentration with satiety visual analogue scale (VAS) AUC (r 0·39; P < 0·05); taurine concentration was also was negatively correlated with hunger VAS AUC (r − 0·43; P < 0·05)(Reference Veldhorst, Nieuwenhuizen and Hochstenbach-Waelen34). In the present study, we found no correlation between the weight of food consumed (g) at 30, 60 or 180 min and positive taurine AUC (data not reported). The weight of food consumed (g) by 1440 min after feeding tended to be correlated (r − 0·25; P = 0·08) with positive taurine AUC, meaning the higher the taurine AUC, the less food a dog consumed. These results are in agreement with VAS correlations presented by Veldhorst et al. (Reference Veldhorst, Nieuwenhuizen and Hochstenbach-Waelen34).
Conclusions
While we were able to influence satiety hormones and plasma AA in the dog after a protein pre-meal, we were unable to influence food intake. Numerically, dogs consumed the least amount of food after the consumption of a salmon or chicken pre-meal. This corresponds with many responses noted with decreased glucose and ghrelin and increased insulin, GLP-1, most insulin-stimulating AA and LNAA. The present study, along with other studies reporting variable results, may indicate that each protein source should be evaluated individually. Earlier reports of fish proteins being more satiating were conducted with Ling fish, a white fish. In the present study, we found that pollock, also a white fish, led to few changes in satiety-related hormones. Again, these substrates were provided in liquid slurries, which may make an impact on retention time in the stomach as well as other indices of satiety. The present study also was conducted using an acute one-time dose of each substrate. Feeding these protein sources as the main dietary protein source in mixed diets may lead to different results. These data, however, provide a starting point for evaluating whole-meal protein sources, with chicken and salmon leading to the greatest changes in satiety response.
Given that the test substrates were human-grade proteins, similar results would be expected in human subjects as well. Using these substrates with human subjects would allow for subjective determinations of satiety, which cannot be measured in dogs. Furthermore, pure skeletal muscle, the high-quality substrates used in the present study, are not commonly utilised in dog diets due to expense. Evaluation of by-products commonly used in the petfood industry may lead to different results, as common fish by-products vary in AA content compared with the substrates used in the present study(Reference Faber, Bechtel and Hernot14, Reference Folador, Karr-Lilienthal and Parsons35). Finally, fish proteins appeared to have similar abilities in modifying satiety markers as compared with avian or mammalian protein sources, but none was able to reduce voluntary food intake in the present study.
Acknowledgements
The present study was supported in part by a US Department of Agriculture, Agricultural Research Service (USDA/ARS) Cooperative agreement.
B. M. V. B. and K. S. S. designed the research; B. M. V. B., T. A. F. and L. L. B. conducted the research; B. M. V. B. analysed the data; S. S., P. J. B. and T. A. F. provided essential materials for this research; B. M. V. B. wrote the manuscript; and G. C. F. had primary responsibility for the final content. All authors read and approved the final manuscript.
P. J. B. works for the USDA/ARS who provided partial funding for the present study. B. M. V. B., T. A. F., L. L. B., K. S. S., S. S. and G. C. F. have no conflicts of interest.