Valine (Val) is one of the essential amino acids (AA) to maintain normal growth and regulate various physiological processes in fish(1). Val, isoleucine and leucine are a group of aliphatic neutral AA with branched-chain structure on the carbon chain, collectively referred to as branched-chain AA(Reference Brosnan and Brosnan2). Fish cannot synthesise Val; therefore, it must be obtained through the ingestion of protein or mixtures of AA in the diet(1). The optimum dietary Val requirements have been established for many fish species, and they range from approximately 1·77 to 4·82 % of dietary protein. Values have been quantified for the following species: lake trout (1·77–2·23 %)(Reference Hughes, Rumsey and Nesheim3), red sea bream (2 %)(Reference Rahimnejad and Lee4), channel catfish (2·96 %)(Reference Wilson, Poe and Robinson5), red drum (3·2–3·5 %)(Reference Castillo and Gatlin6), Indian major carp (3·8 %)(Reference Ahmed and Khan7), blunt snout bream (3·71–3·88 %)(Reference Ren, Habte-Tsion and Liu8), rainbow trout (3·85–4·1 %)(Reference Bae, Park and Yun9), Jian carp (4 %)(Reference Dong, Feng and Kuang10), Nile tilapia, (4·11–4·53 %)(Reference Xiao, Li and Zhu11), golden pompano (4·6–4·7 %)(Reference Huang, Tan and Zhou12) and grass carp (4·77–4·82 %)(Reference Luo, Feng and Jiang13). However, dietary Val imbalance (deficiency or excess) has also been reported to negatively influence growth performance, feed efficiency (FE), protein deposition and antioxidant status of fish(Reference Rahimnejad and Lee4,Reference Ahmed and Khan7,Reference Dong, Feng and Kuang10) .
Target of rapamycin (TOR) is a highly conserved serine/threonine kinase, complex compounds with two different structures and functions TORC1 and TORC2(Reference Eunjung14). In the eukaryotic cells’ TOR signalling pathway, TORC1 as a target for to perceive intracellular signals such as AA and growth factors has the function of regulating cell growth, metabolism and survival through phosphorylation of downstream translation regulatory factors S6 kinase 1 (S6K1)(Reference Lynch15–Reference Li, Yin and Tan20). Zhou et al.(Reference Zhou, Zhou and Peng21) reported that Val activated the mTOR pathway through regulating phosphorylation of S6K1 and eIF4E-binding protein in bovine mammary epithelial cells. On the other hand, growth hormone (GH) initiates muscle growth-promoting actions by binding to GH receptors (GHR) and stimulating the synthesis and secretion of insulin-like growth factor-1 (IGF-1) from the liver and other sites in fish(Reference Moriyama, Ayson and Kawauchi22–Reference Reindl, Kittilson and Bergan25). Further research suggests that the GH/IGF-1 system regulates protein synthesis and degradation through the target of PI3K/AKT/TOR signalling pathway(Reference Fuentes, Safian and Einarsdottir26). Brameld et al.(Reference Brameld, Gilmour and Buttery27) reported that in the pig hepatocyte culture system, the removal of a certain percentage of Val from the culture medium inhibited the expression of GH, GHR and IGF-I. However, studies have not investigated the effects of dietary Val on the interaction GH/IGF-1 and TOR pathway of fish.
Val can also affect the antioxidant status and immune system of animals. Feng et al.(Reference Feng, Luo and Jiang28) reported that Val deficiency in the diet impaired the structural integrity of fish gill, and Val may regulate the expression of antioxidant enzymes through the TOR and NF-E2-related factor 2 (Nrf2) signalling pathways. In red sea bream, it was reported that optimum dietary Val level significantly increased the content of lysozyme (LZM) and Ig(Reference Rahimnejad and Lee4).
Hybrid grouper is one of the excellent cultured marine fish species in China, and its optimum dietary levels of several nutrients have been established in our previous studies including protein and lipid levels(Reference Jiang, Wu and Li29,Reference Jiang, Wu and Luo30) , dietary protein:energy ratio(Reference Jiang, Wu and Luo30) and dietary reference AA profile(Reference Wu, Lu and Wu31), as well as optimum dietary arginine, lysine, leucine(Reference Wu, Wu and Lu32–Reference Zhou, Wang and Wu34) and isoleucine (Submissions in progress) requirement levels. No data are currently available on the optimum Val requirement of hybrid groupers; therefore, the main objective of the present study was to investigate the effects of dietary Val levels on growth, protein synthesis, antioxidant status and gut morphology of hybrid grouper juveniles and hence quantify the optimum dietary Val requirement of this species. This will facilitate the study of the relationship between branched-chain AA while maintaining an ideal dietary reference AA profile for hybrid groupers’ optimal performance.
Methods
Ethical statement
We declare that the study was conducted following the 3R principles, and the care and use of animals in this study obey Chinese Animal Management Regulations (2017).
Experimental diets
Seven isoenergetic (1423 kJ (340 kcal)/100 g DM), isoproteic (49·3 % of DM) and isolipidic (7 % of DM) experimental diets were formulated to contain graded l-Val levels, ranging from 1·21 to 1·94 % of DM (Table 1). The 49·3 % protein level and the 7 % crude lipid level in this research were selected based on our previous studies(Reference Jiang, Wu and Li29,Reference Jiang, Wu and Luo30,Reference Wu, Wu and Lu32–Reference Zhou, Wang and Wu34) . Dietary arginine, lysine and leucine levels were designed as 3·65, 2·16 and 3·25 % of DM, respectively, according to our published results(Reference Wu, Wu and Lu32–Reference Zhou, Wang and Wu34). Analysed dietary Val concentrations were 1·21, 1·32, 1·45, 1·58, 1·69, 1·82 and 1·94 % of DM (% DM) (Table 2).
Table 1. Formulations and analysed composition of experimental diets (DM basis)
(Percentages)
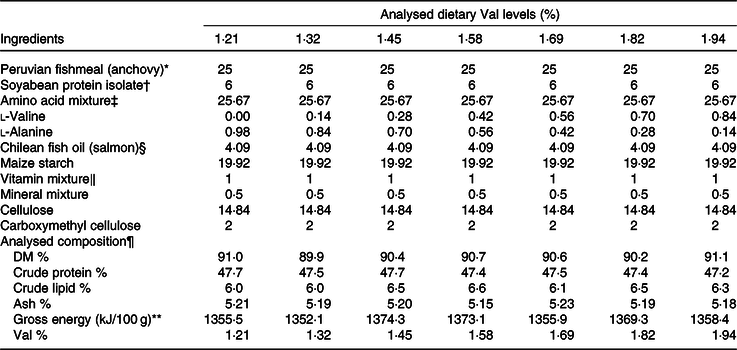
Val, valine.
* Yongsheng Feed Corporation; proximate composition (% DM): moisture, 7·3; crude protein, 71·29; crude lipid, 12·1.
† Sonac (China) Biology Co. Ltd; proximate composition (% DM): moisture, 0·02; crude protein, 91·55; crude lipid, 0·11.
‡ Amino acid mixture (g/100 g): l-arginine, 2·00; l-methionine, 1·26; l-threonine, 1·36; l-leucine, 1·40; l-phenylalanine,0·97; l-isoleucine, 0·82; l-histidine, 0·51; l-aspartic acid, 2·88; l-serine, 1·12; l-glutamic acid, 5·87; glycine, 1·77; l-alanine, 2·05; l-cystine, 0·60; l-tyrosine, 0·83; l-proline, 1·15; l-lysine sulphate (55 % lysine), 0·37.
§ Blue Ocean Marine Biological Technology Co. Ltd.
‖ Vitamin mixture and mineral mixture, see Lin & Shiau(Reference Lin and Shiau57).
¶ Values represent means of duplicate samples.
** By calculation: fuel values for carbohydrate, protein and lipid were 4·0, 4·0 and 9·0 kcal/g (16·7, 16·7 and 37·7 kJ/g), respectively.
Table 2. Analysed amino acid (AA) compositions of experimental diets (DM basis)
(Percentages)

Val, valine; EAA, essential amino acids; NEAA, non-essential amino acids.
Fishmeal was finely ground and sieved (mesh size: 0·250 mm), and all dry ingredients except crystalline AA were carefully weighed and mixed for 30 min in a Hobart mixer (A-200 T Mixer Bench Model unit; Resell Food Equipment Ltd). Crystalline AA were weighed individually for each of the experimental diets. The crystalline AA mixture (26·43 g/100 g diet) was pre-coated with 1·0 g carboxymethyl cellulose and 14·84 g cellulose/100 g DM in water at 40°C to form a dough which subsequently was rubbed into powder with hands. The bound crystalline AA was then mixed with other dry ingredients and re-coated with 19·92 g maize starch and another 1·0 g carboxymethyl cellulose/100 g of diet, and then lipid and water (30–50 % of dry ingredient mixture) were added gradually in sequence and mixed constantly. The diets were formed into a noodle-like shape of 3 mm diameter using a twin-screw meat grinder (Institute of Chemical Engineering, South China University of Technology, Guangzhou, PR China). All diets were air-dried at 25°C for 24 h, sieved and then packaged and stored frozen (−20°C).
Experimental procedures
Hybrid grouper juveniles were obtained from a commercial hatchery (Guilinyang). Prior to the feeding trial, experimental fish were acclimated to the water-recycling system consisted of twenty-one glass tanks (length 60 cm × width 45 cm × height 50 cm) with a commercial diet (crude protein: 50 % of DM, crude lipid: 7 % of DM) for 14 d. Thereafter, experimental fish (average initial body weight: 10·80 (sd 0·01) g) were randomly distributed into tanks at a density of fifteen fish per tank, and each dietary treatment had three replicates. Fish were fed their assigned diets by hand to apparent satiation twice daily (at 08.00 and 16.00 hours). The feed intake was recorded every day, and the feeding period was 6 weeks. Water temperature (27–29°C), total ammonia (0–0·20 mg/l) and dissolved oxygen (5·8–6·8 mg/l) were monitored daily. Fish were exposed to a 12 h light–12 h dark cycle.
Sampling and analysis
At the beginning of this trial, ten fish were sampled and stored at −20°C for analysis of initial whole-body proximate composition. At the end of the trial, experimental fish were fasted for 14 h prior to the sampling. After fish were anaesthetised with MS-222 (0·1 g/l), two fish per tank were collected for whole-body composition analysis and another three fish per tank were individually weighed, separately bled from the caudal vasculature using 1 ml heparinised (H6279; SIGMA) syringes and dissected to obtain viscera, liver and weights for computing body condition indices including hepatosomatic index ((liver wt./live wt.) × 100), respectively. Intraperitoneal fat was obtained by removing and weighing the fat from the abdominal cavity as well as that adhering to the gut of the fish. Condition factor was also computed as (body weight × 100)/(body length)3. White muscle was also taken off at this dissection. For gene expression assays, another three individuals from each tank were randomly collected and dissected to obtain pituitary, liver and head kidney samples which were immediately frozen in liquid N2 and then stored at −80°C. At this time, gastrointestinal tract samples for histological analysis were also obtained. After the sampling, the blood was centrifuged (at 3000 g , 15 min, 4°C) (centrifuge 5417 R; Eppendorf) and the serum was separated and stored at −80°C for later analysis.
Proximate analysis procedures included crude protein (N × 6·25) being determined by the Dumas combustion method using a rapid MAX N exceed system (Elementar), crude lipid being determined by ether extraction using a Soxtec System HT (Soxtec System HT6, Haineng SOX406) and DM being determined by heating about 2 g samples at 125°C for 3 h(35). For AA analysis, the diets were hydrolysed with 6 m hydrochloric acid at 110°C, being under an atmosphere of N2 for 24 h. AA analysis was carried out utilising a pre-column derivatisation procedure with aminoquinolyl-N-hydrosysuccinimidyl carbamate by HPLC (Waters 2690 System).
Histological examination of the foregut and midgut
For histological analysis, foregut and midgut were separated from the gastrointestinal tract and washed in a saline solution before fixing in Bouin’s fixative solution for 24 h. After serial dehydration steps in alcohol, samples were embedded in paraffin. The blocks of embedded tissue were sectioned at 5 μm, and sections were routinely stained with haematoxylin–eosin and observed under a light microscope (Olympus IX71) equipped with the Image-Pro Plus 7.0 software. Digitalised images were analysed to measure the micrometre length of various enteric structures. Average fold height, fold width, enterocyte height and microvillus height were determined per slice (fifteen fields per individual sample) according to the procedures described by Escaffre et al.(Reference Escaffre, Kaushik and Mambrini36).
Determination of serum antioxidant and immune indices
IgM concentrations and activities of LZM, superoxide dismutase and catalase in serum were determined using ELISA analysis kits (Kit nos: C0197170176, F18019756, E25019758 and F01019757; Cusabio) according to the procedures provided by the manufacturer.
Total RNA extraction and reverse transcription
Total RNA was extracted from grouper pituitary, liver and head kidney using Trizol Reagent (Invitrogen) followed by quality measurement on a 1·0 % denaturing agarose gel and yield determination on NanoDrop®ND-1000. The brightness detection integrity of total RNA at 28S, 18S and 5S was observed according to the electrophoresis bands, and the optical density ratio between 260 and 280 was in the range of 1·8–2·0. Next, the RNA was treated with RNA-free DNase (Takara) to remove DNA contaminant and reversely transcribed to complementary DNA by a Prime Script™ RT reagent kit with gDNA Eraser (Takara) following the instructions provided by the manufacturer.
Real-time quantitative PCR analysis of neuro-endocrine growth axis, target of rapamycin-related and antioxidant genes
Real-time RT-PCR was carried out in a quantitative thermal cycler (QuantStudio 6 Flex, Applied Biosystems). The amplification was performed in a total volume of 10 μl containing 5 μl TB Green™ Premix Ex Taq™ II (Takara), 0·2 μl of each primer (10 μmol/l), 4·1 μl of nuclease-free water and 0·5 μl of complementary DNA mix. The real-time RT-PCR programme was as follows: 95°C for 30 s, followed by forty cycles of 95°C for 5 s, 56°C for 30 s and 72°C for 30 s, then 95°C for 15 s, 60°C for 1 min and 95°C for 15 s. The real-time RT-PCR primer pairs for GH, GHR1, IGF-1, TOR, S6K1, Nrf2, Kelch-like-ECH-associated protein 1 (Keap1) and β-actin were designed by Primer Premier 5.0 based on the published nucleotide sequences and are listed in Table 3. At the end of each PCR reaction, melting curve analysis of amplification products was carried out to confirm that a single PCR product was present in these reactions. Standard curves were made with five different dilutions (in triplicate) of the complementary DNA samples, and amplification efficiency was analysed according to the following equation: E = 10(−1/slope) − 1. The expression levels of the target genes were calculated followed the 2−ΔΔct method described by Livak & Schmittgen(Reference Livak and Schmittgen37).
Table 3. Primers used for quantitative RT-PCR (qPCR)

GH, growth hormone; F, forward sequence; R, reverse sequence; GHR1, growth hormone receptor 1; IGF-1, insulin-like growth factor-1; TOR, target of rapamycin; S6K1, ribosomal protein S6 kinase 1; Keap1, Kelch sample-related protein-1; Nrf2, NF-E2-related factor 2.
Western blotting
The hepatic samples were used to detect the protein levels of S6K1. Protein extracts (30 μg) were separated via SDS-PAGE and transferred to a nitrocellulose membrane. The membranes were blocked in 5 % non-fat dried milk in TRIS-buffered saline–0·1 % Tween-20 (TBST) for 3 h. After the closure was finished, the primary antibody S6k1 (catalogue no. ab186753; Abcam) was added, primary antibody stock solution was diluted with TBST at 1:1000 and incubated overnight at 4°C. After being washed five times with TBST, the membranes were incubated at room temperature for 3 h with horseradish peroxidase-linked secondary antibodies: GAPDH (catalogue no. ab181602, Abcam) diluted 1:1000 in TBST (catalogue no. 7074; Cell Signaling Technology). Finally, the membranes were washed three times with TBST, followed by development using the Ultra hypersensitive ECL chemiluminescence kit according to the manufacturer’s instructions (Beyotime). Images were quantified using the Amersham Imager 600 QC system and ImageJ software.
Statistical analysis
Normality and homoscedasticity assumptions were confirmed prior to any statistical analysis. As mean separation tests after ANOVA are technically not a correct statistical analysis for quantitative data such as that generated by feeding graded levels of a nutrient(1), the regression analysis of orthogonal polynomial contrasts was performed using the SPSS 18.0 (SPSS Inc.) to evaluate the responses to graded Val levels and all evaluated variables were subjected to an ANOVA to determine if dietary Val levels significantly (P < 0·05) affected the observed responses. The asymptote of the regression was used to determine the optimum dietary Val requirements.
Results
Growth performance and feed utilisation
Results of the growth performance and feed utilisation of hybrid grouper juveniles fed different dietary Val levels are presented in Table 4. Survival showed no significant differences among all experimental treatments. Weight gain percentage (WG%) of experimental fish was significantly affected by dietary Val levels, with fish fed 1·58 % dietary Val having the highest WG% which decreased in fish fed higher dietary Val levels. Values of protein productive value, protein efficiency ratio and FE showed similar trends to that of WG%. Quadratic regression analysis of WG%, protein productive value, protein efficiency ratio or FE against dietary Val levels indicated that optimal dietary Val level of hybrid groupers was 1·56, 1·61, 1·61 or 1·60 %, respectively (Fig. 1). Daily feed intake showed an opposite tendency compared with FE.
Table 4. Growth performance and feed utilisation of hybrid grouper juveniles fed different dietary valine (Val) levels for 6 weeks
(Mean values with their standard errors)

WG%, weight gain percentage; FE, feed efficiency; DFI, daily feed intake; PER, protein efficiency ratio; PPV, protein productive value; PSE, pooled standard error of treatment means; SOP, second-order polynomial trend.
* WG% = 100 × (final average body weight − initial average body weight)/initial average body weight.
† FE = weight gain (g)/dry feed intake (g).
‡ DFI = 100 × dry feed intake (g)/weight gain (g)/number of days.
§ PER = weight gain (g)/protein intake (g).
‖ PPV = body protein weight gain (g)/protein intake (g).
¶ Survival % = 100 × (final number of fish)/(initial number of fish).

Fig. 1. Relationship of weight gain percentage, feed efficiency, protein productive value and protein efficiency ratio of hybrid grouper juveniles fed different dietary valine (Val) levels.
Body condition indices, whole-body and muscle proximate composition
Hepatosomatic index and condition factor were significantly affected by dietary Val levels (Table 5), both of which gradually increased with dietary Val levels, and obtained the maximum for fish fed 1·82 % dietary Val and decreased thereafter. Fish fed 1·58 % dietary Val showed higher protein contents in whole-body and muscle tissues than fish fed the other dietary Val levels. There were no significant differences in moisture content of whole-body and muscle tissues among all experimental treatments. Fish fed 1·58 and 1·96 % dietary Val levels also had higher lipid content in whole-body compared with fish fed the other levels of Val.
Table 5. Body condition indices, whole-body and white muscle compositions (wet basis) of hybrid grouper juveniles fed different dietary valine (Val) levels for 6 weeks
(Mean values with their standard errors)
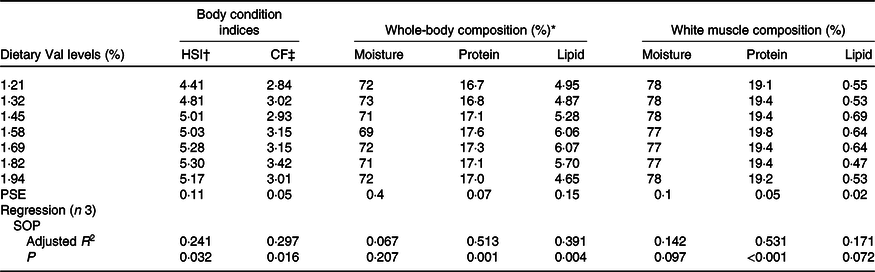
HSI, hepatosomatic index; CF, condition factor; PSE, pooled standard error of treatment means; SOP, second-order polynomial trend.
* Initial whole-body composition (%): moisture = 75·27; protein = 16·75; lipid = 3·56.
† HSI = 100 × liver weight (g)/whole-body weight (g).
‡ CF = 100 × body weight (g)/body length (cm)3.
Gut micromorphology and expression of growth hormone/insulin-like growth factor 1 axis and target of rapamycin-related genes
Gut morphometric parameters are summarised in Table 6, and they were significantly affected by different dietary Val levels with fish fed 1·58 % dietary Val having the highest fold height, fold width and microvillus height values in foregut and midgut samples among all experimental treatments.
Table 6. Gut micromorphology of hybrid grouper juveniles fed different dietary valine (Val) levels for 6 weeks

hF, fold height; wF, fold width; hMV, microvillus height; PSE, pooled standard error of treatment means; SOP, second-order polynomial trend.
The relative mRNA expression levels of pituitary GH and hepatic IGF-1 of hybrid grouper juveniles fed 1·58 % dietary Val were higher than those of fish fed the other dietary Val levels. The highest GHR mRNA expression occurred in fish fed 1·45 % dietary Val (Fig. 2). For TOR-related signalling molecules, fish fed 1·58 % dietary Val also had higher expression of TOR and S6K1 in liver than fish fed the other dietary Val levels (Fig. 3).

Fig. 2. Expression of pituitary growth hormone (GH), hepatic growth hormone receptor (GHR) and insulin-like growth factor-1 (IGF-1) of hybrid grouper juveniles fed different dietary valine (Val) levels for 6 weeks (n 9). Relative mRNA expression was evaluated by real-time quantitative PCR. The gene expression of the 1·21 % Val group was set at 1. SOP, second-order polynomial trend.
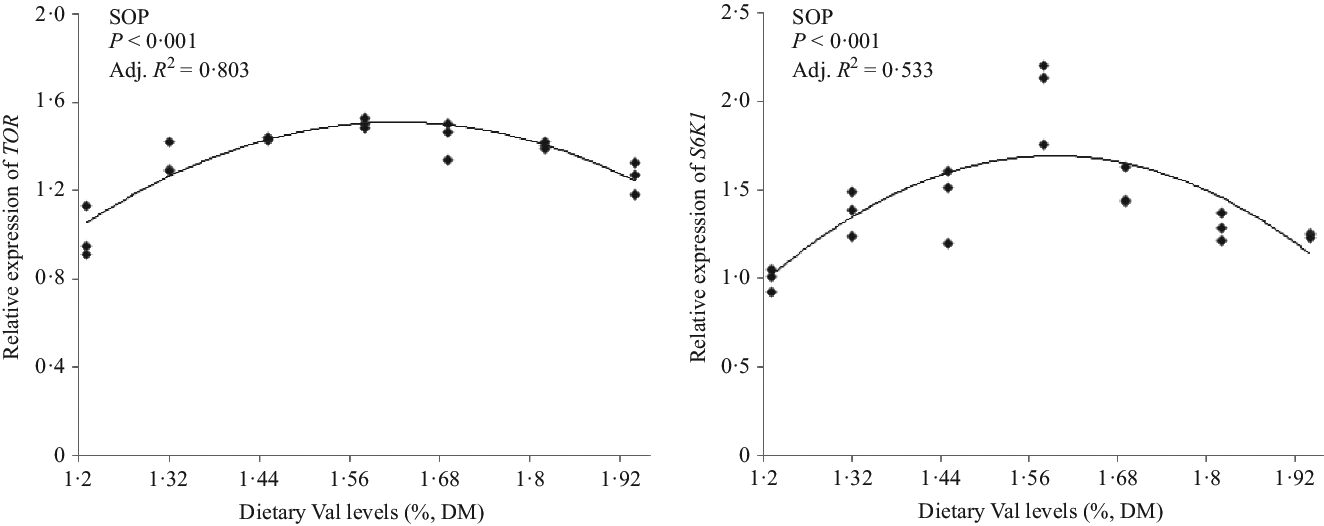
Fig. 3. Expression of hepatic target of rapamycin (TOR) and S6 kinase 1 (S6K1) of hybrid grouper juveniles fed different dietary valine (Val) levels for 6 weeks (n 9). SOP, second-order polynomial trend.
Protein levels of hepatic S6 kinase 1
The S6K1 protein levels were significantly affected by dietary Val levels (Fig. 4). Fish fed 1·21 % dietary Val had the lowest hepatic protein level of S6K1 among all experimental treatments.

Fig. 4. Protein levels of hepatic S6 kinase 1 (S6K1) of hybrid grouper juveniles fed different dietary valine (Val) levels for 6 weeks (n 3). GAPDH, glyceraldehyde 3-phosphate dehydrogenase.
Immune and antioxidant indices in serum and head kidney
Fish fed 1·58 % dietary Val had higher IgM concentrations as well as LZM, superoxide dismutase and catalase activities in serum compared with fish fed the other dietary Val levels (Fig. 5). Expression of Nrf2 in head kidney of fish fed 1·58 % dietary Val was higher than that of fish fed the other dietary Val levels (Fig. 6). Expression of keap1 in head kidney showed an opposite tendency compared with Nrf2, with fish fed 1·58 % Val having lower values than fish fed the other dietary Val levels.

Fig. 5. Serum IgM and lysozyme (LZM) concentrations, and superoxide dismutase (SOD) and catalase (CAT) activities of hybrid grouper juveniles fed different dietary valine (Val) levels for 6 weeks (n 3). SOP, second-order polynomial trend.

Fig. 6. Expression of NF-E2-related factor 2 (Nrf2) and Kelch-like-ECH-associated protein 1 (Keap1) in head kidney of hybrid grouper juveniles fed different dietary valine (Val) levels for 6 weeks (n 9). SOP, second-order polynomial trend.
Discussion
Results of the present study indicated that the optimum dietary Val requirement for maximum WG% of juvenile hybrid groupers was estimated to be 3·16 % of dietary protein (corresponding to 1·56 % of DM). This value was approximately equal to that (2·96 %) reported in channel catfish(Reference Wilson, Poe and Robinson5) and red drum (3·2–3·5 %)(Reference Castillo and Gatlin6), but higher than that reported for lake trout (1·77–2·23 %)(Reference Hughes, Rumsey and Nesheim3) and red sea bream (2 %)(Reference Rahimnejad and Lee4), lower than that reported for golden pompano (4·6–4·7 %)(Reference Huang, Tan and Zhou12), grass carp (4·77–4·82 %)(Reference Luo, Feng and Jiang13) and rainbow trout (3·85–4·1 %)(Reference Bae, Park and Yun9). The discrepancies among these research findings may be ascribed to various factors including differences in genetics of the various fish species, environment conditions during the growth trial and fish size/age.
In this study, the lower WG% observed in fish fed 1·21 or 1·94 % dietary Val compared with that of fish fed 1·58 % dietary Val indicated that both excess and insufficiency of Val in diets could induce a decrease in growth performance of juvenile hybrid groupers, being in agreement with the results reported in golden pompano(Reference Huang, Tan and Zhou12) and Indian major carp(Reference Abidi and Khan38). Studies in mammals show that due to the similarity of the side-chain structure of the three branched-chain AA, they compete with each other for common transport carriers and catabolic enzymes in the body such that imbalances of one or more branched-chain AA may cause antagonistic effects(Reference Block and Harper39–Reference Zhang, Zeng and Ren41). On the other hand, studies have shown that the effect of dietary Val imbalance on fish growth performance may be attributed to loss of appetite and low feed utilisation(Reference Rahimnejad and Lee4,Reference Ahmed and Khan7,Reference Dong, Feng and Kuang10) . It was also observed in the present study that when the levels of Val were insufficient or excessive, both lead to the decrease of FE and the increase of daily feed intake in hybrid groupers.
Protein deposition appears to be the main determinant of live weight (biomass) gain in fish(1). The higher muscle and whole-body protein contents in fish fed 1·58 % dietary Val, compared with other dietary Val levels, indicated that optimum Val supplementation improved protein deposition of hybrid groupers, which coincided with the higher protein productive value and protein efficiency ratio values observed in fish fed 1·58 % dietary Val level. These results also agreed with data from the studies on Indian major carp(Reference Ahmed and Khan7), red sea bream(Reference Rahimnejad and Lee4) and golden pompano(Reference Huang, Tan and Zhou12). Zhou et al.(Reference Zhou, Zhou and Peng21) reported the Val activated mTORC1 and the phosphorylation of downstream translation regulatory factors S6K1 and eIF4E-binding protein 1 in bovine mammary primary epithelial cells. Excessive cottonseed meal protein hydrolysate substituted for fishmeal decreased Val content in plasma and muscle, thus significantly decreasing liver TOR and S6K1 expression levels of blunt snout bream(Reference Yuan, Liu and Cheng42). In the present study, suitable Val supplementation levels in diets improved the transcription abundances of hepatic TOR gene and also the transcription abundances and protein concentrations of hepatic downstream translation regulatory factors S6K1. These results indicated that the optimal dietary Val requirement is able to stimulate protein synthesis via the TOR/S6K1 signalling pathway and thus promote the growth of juvenile hybrid groupers.
The GH/IGF-1 system is the key promoter to activate muscle growth in teleost species(Reference Fuentes, Einarsdottir and Valdes24). Studies have shown that dietary addition of suitable essential AA can up-regulate the transcription levels of GH/IGF-1 growth axis-related genes and thus promote fish growth(Reference Wu, Wu and Lu32). On other hand, the GH/IGF-1 system is known to regulate protein synthesis and degradation through the PI3K/AKT/TOR signalling pathways(Reference Fuentes, Safian and Einarsdottir26,Reference Bower and Johnston43) . Furthermore, studies in gilthead sea bream have shown that AA and IGF-1 produce synergistic effects that transduction involved in TOR/AKT signalling pathway and stimulate myocyte proliferation and differentiation(Reference Vélez, Lutfi and Jiménez-Amilburu44). Brameld et al.(Reference Brameld, Gilmour and Buttery27) reported that in a pig hepatocyte culture system, removal of a certain percentage of Val (16 % of control) from the culture medium inhibited the expression of GH, GHR and IGF-I. In the present study, it was found that fish fed 1·45 to 1·58 % dietary Val had higher expression of pituitary GH, hepatic GHR1 and IGF-1 compared with the other experimental groups. This demonstrated that dietary Val can serve as a factor regulating expression of genes of the GH/IGF axis, jointly promoting muscle cell growth and protein deposition of hybrid groupers via the TOR/S6K1 signalling pathway.
Nrf2 is a transcriptional factor regulating antioxidant stress in cells, and Keap1 is an actin-anchored protein that prevents nuclear translocation of Nrf2. Under oxidative stress, the ability of Keap1 to ubiquitinate and degrade Nrf2 is diminished, thus promoting restoration of body homoeostasis through Nrf2 binding to antioxidant response elements to regulate the transcription of downstream antioxidant genes(Reference Zhang45,Reference Tsion46) . Superoxide dismutase and catalase are two important enzymes in the antioxidant defence system in fish, helping to eliminate the superoxide anion free radicals generated by the organism’s oxidative processes, and their activity is regulated by gene transcription level(Reference Lambertucci, Levada-Pires and Rossoni47,Reference Wilhelm48) . Studies in mice have shown that the TOR signalling pathway is thought to activate key regulators of Nrf2 transcription and degradation of Keap1, thereby preventing oxidative damage in the liver(Reference Bae, Sung and Oh49). Feng et al.(Reference Feng, Luo and Jiang28) reported that Val deficiency impaired the structural integrity of grass carp gill and may regulate the expression of antioxidant enzymes through the TOR and Nrf2 signalling pathways, thus affecting their activity. Not only the gills but also the head kidney are considered important immune organs in teleosts(Reference Wu, Jiang and Liu50). In the present study, fish fed 1·58 % dietary Val had the highest expression of Nrf2 and the lowest expression of Keap1 both in head kidney. When dietary Val was imbalanced, the expression results of Nrf2 and Keap1 were similar to those of grass carp, indicating improvement of antioxidant status of hybrid groupers fed this optimum Val level. The variations in serum catalase and superoxide dismutase activity in the present study indicated that suitable dietary Val levels could improve antioxidant activity of hybrid groupers. Similar results were also observed in Nile tilapia(Reference Xiao, Li and Zhu11) and golden pompano(Reference Huang, Tan and Zhou12). However, the underlying mechanism between the TOR/S6K1 and Keap1-Nrf2 pathways requires further investigation.
In this study, dietary Val levels were found to significantly affect the innate immune responses of hybrid groupers regarding the alterations in serum LZM activity and IgM concentrations. IgM and LZM are important immune factors in response to external pathogenic micro-organisms involved in immune regulation(Reference Wilhelm48). IgM is a class of glycoproteins produced by differentiation of B lymphocytes after antigen stimulation, through antigen-specific non-covalent processes, and produces a series of immune responses(Reference Tian, Sun and Luo51). LZM is one of the important non-specific immune factors in fish, which can hydrolyse bacterial cell walls, and its bacteriolytic capability is positively correlated with its activity(Reference Grinde, Lie and Poppe52). Results from this study regarding IgM and LZM responses to dietary Val agree with those reported for juvenile red sea bream(Reference Rahimnejad and Lee4).
The gut is a critically important site for digestion and absorption of nutrients, which is related closely to the efficiency of feed utilisation and hence growth performance(Reference Dimitroglou, Merrifield and Moate53,Reference Zhou, Buentello and Gatlin54) . Allameh et al.(Reference Allameh and Toghyani55) reported that in broiler chickens, Val supplementation to low-CP diets improved villus height of the small intestine. Meanwhile, it was observed in broilers that suitable supplementations of leucine to diets may promote gut protein synthesis by activation of the mTOR pathway which promotes the gut epithelial cell proliferation, leading to the improvement of villus height and growth of the small intestine(Reference Chang, Cai and Liu56). However, in fish, no previous study has addressed the effects of dietary Val on gut morphology. In the present study, the morphological results in the foregut and midgut indicated that dietary Val at 1·58 % increased the fold height, fold width and microvillus height of hybrid groupers, which may also partially explain the rapid growth of fish fed this diet.
In conclusion, results of the present study demonstrated that the optimum dietary Val requirement for maximum weight gain of hybrid groupers was 1·56 % of DM, corresponding to 3·16 % of dietary protein. Below or above this optimum level, growth performance, protein synthesis, gut micromorphology, immune and antioxidant status of fish were reduced. The optimal dietary Val requirement is able to stimulate protein synthesis via the TOR/S6K1 signalling pathway.
Acknowledgements
The authors wish to thank the Editor and anonymous reviewers for their valuable suggestions for improving our manuscript.
This study was supported by a grant (no. 31760760) from The National Natural Science Fund of China and a grant (no. ZDYF2018055) from Key R&D projects of Hainan Province.
All authors have read the article and agreed to its publication in the British Journal of Nutrition.
The authors declare that there are no conflicts of interest.