Deficient early nutrition causes reduction in growth, augmented efficiency of fat storage and lower lean mass associated with a high risk of developing chronic diseases such as, hypertension, insulin resistance and obesity in adult life( Reference Hales and Barker 1 , Reference Falcao-Tebas, Bento-Santos and Fidalgo 2 ). Maternal malnutrition from conception through lactation may also affect the structure and/or function of the immune system by permanently altering specific cell populations during development( Reference Moreno Senna, Ferraz and Leandro 3 ). In humans, maternal protein restriction reduces the number of T lymphocytes and antibody responses to vaccination for the neonate and the young infant( Reference Jones, Berkley and Warner 4 , Reference Najera, Gonzalez and Toledo 5 ). In rats, previous studies have shown that a maternal low-protein diet (LP) is related to impaired spreading, phagocytosis and microbicide functions of macrophages when faced with an immune challenge in young rats( Reference Prestes-Carneiro, Laraya and Silva 6 , Reference Ferreira, Galvao and Ferraz-Pereira 7 ). Conversely, the high consumption of a Western diet, characterised mainly by industrialised foods rich in fat, refined carbohydrate, added sugar and energy content, contributes to an increase in body weight gain, fat accumulation that may contribute to the appearance of a chronic low-grade inflammation observed in obese individuals( Reference Ouchi, Parker and Lugus 8 ). It seems that the association of under- and overnutrition maximises the risk of metabolic disorders such as obesity, diabetes type II, dyslipidaemia, hypertension and CVD( Reference Gluckman, Hanson and Beedle 9 ).
A previous study has reported on the interaction between maternal diet and amplification by a post-weaning high-fat (HF) diet, and demonstrated that profound adult hyperphagia is a consequence of fetal programming and a key contributing factor in adult pathophysiology( Reference Vickers, Breier and Cutfield 10 ). Maternal protein restriction (8 % casein) during gestation and lactation followed by a post-weaning HF diet (41 % fat) induced an increased percentage of visceral fat, reduced insulin sensitivity and increased food intake in adult offspring( Reference Gosby, Maloney and Caterson 11 ). Continuous intake of a HF diet can also promote hypertrophy and dysfunction of adipocytes( Reference Ouchi, Parker and Lugus 8 ). This may induce infiltration of pro-inflammatory macrophages in adipose tissue, enhancing the production of pro-inflammatory cytokines in this tissue( Reference Ouchi, Parker and Lugus 8 ). The macrophage content of adipose tissue is positively correlated to two indices of adiposity, BMI) and adipocyte size; and lean individuals present a smaller number of inflammatory macrophages( Reference Weisberg, McCann and Desai 12 ). On the other hand, adipose macrophages in obese individuals are tilted towards an M1-like pro-inflammatory phenotype( Reference Aron-Wisnewsky, Tordjman and Poitou 13 ). Visceral fat samples show that adipose-resident macrophages up-regulate the pro-inflammatory cytokine gene and protein expression( Reference Aron-Wisnewsky, Tordjman and Poitou 13 ). Given the experimental model of fetal programming by maternal undernutrition, it is important to evaluate the functions, for example phagocytosis and nitric oxide (NO) production, of non-adipose tissue resident peritoneal macrophages in the beginning of fat accumulation, at least in young rats.
Phagocytosis consists of a process of recognition and ingestion of particles that results in the activation of a respiratory burst and production of reactive O and N species with important cytolytic properties( Reference Teshima, Rokutan and Takahashi 14 ). NO production is also an effector function of activated macrophages to kill pathogens( Reference Teshima, Rokutan and Takahashi 14 ). Resident peritoneal macrophages from adult pups submitted to perinatal LP diet showed reduced phagocytic activity against Candida albicans ( Reference Prestes-Carneiro, Laraya and Silva 6 ). Chronic consumption of a HF diet decreased the lipopolysaccharide (LPS)-induced inflammatory response of the peritoneal macrophages, which was associated to a down-regulation of the NF-κB signalling pathway( Reference Borges, Vinolo and Crisma 15 ). However, there is no information on how perinatal malnutrition followed by post-weaning consumption of a HF diet may alter peritoneal resident macrophage populations outside the adipose tissue and how this affects the phagocytosis activity and NO production by these macrophages.
The present study tested the hypothesis that the interaction between maternal diet and post-weaning HF diet causes increased food intake, fat accumulation and amplifies the impaired peritoneal macrophage functions in young rats. Thus, the main goal of this study was to evaluate the effects of a post-weaning HF diet on somatic growth, food consumption, fat accumulation, plasma glucose, lipids and leptin concentration, phagocytosis rate and the NO production by peritoneal macrophages of young rats submitted to a maternal LP diet.
Methods
The experimental protocol was approved by the Ethics Committee of the Biological Sciences Centre (protocol no. 23076.052139/2012-01), Federal University of Pernambuco, Recife, PE, Brazil, and followed the Guidelines for the Care and Use of Laboratory Animals( Reference Bayne 16 ).
Animals and diets
Virgin female albino Wistar rats (Rattus norvegicus) and males of the same strain were obtained from the Department of Nutrition, Federal University of Pernambuco. The female rats weighed 220–280 g and were 90–120 d old when they mated. The day on which spermatozoa were present in a vaginal smear was designated as the day of conception (day 0 of pregnancy). Pregnant rats were then transferred to individual cages and maintained at room temperature of 22±1°C with a controlled 12 h light–12 h dark cycle (06.00–18.00 hours). Pregnant rats were randomly divided into two groups (n 8/each): control (C, fed a normal protein diet with 17 % casein during gestation and lactation) and LP diet (fed a LP diet with 8 % casein during gestation and lactation) (AIN-93G)( Reference Reeves 17 ). Water and experimental diet were given ad libitum. After delivery, offspring were maintained as litters of eight pups (C and LP). At weaning (21d old), two or three male offspring from each litter received either commercial standard rodent chow Nuvilab® (CR1; Nuvital Nutrientes S/A) or a HF diet (Table 1). Then, four groups of male offspring rats (n 11/each) were formed as follow: C (animals fed normal protein diet during gestation and lactation and standard chow post weaning), LP (animals fed LP diet during gestation and lactation and standard chow post weaning), HF (animals fed normal protein diet during gestation and lactation and HF diet post weaning) and low protein–high fat (LP-HF, animals fed LP diet during gestation and lactation and HF diet post weaning). The HF diet consisted of commercial standard chow plus peanuts, milk chocolate and sweet biscuits in the proportion of 3:2:2:1( Reference Estadella, Oyama and Damaso 18 ). The fatty acids composition of HF diet is shown in Table 1. The pups were fed this experimental diet from weaning until the 60th day of life, when they were killed by decapitation.
Table 1 Chemical composition of high-fat diet and standard chow used in the experiments
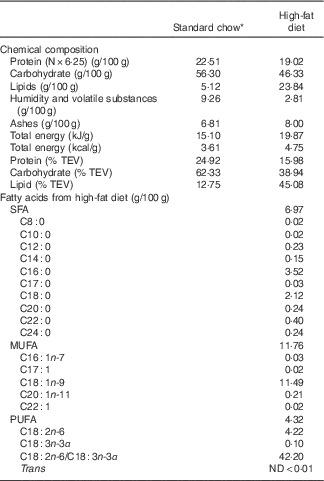
TEV, total energetic value; ND, non detectable.
* Nuvilab CR1 (Nuvital Nutrients S/A).
Mother’s body weight and food intake
The mother’s body weight was recorded on the 1st, 7th, 14th, and 21st days of gestation and lactation by using a Marte scale (BL3200H) with 0·001 g accuracy. The percentage of body weight gain (%BWG) was calculated as follows: (final body weight (g)×100/initial body weight (g))–100( Reference Fidalgo, Falcao-Tebas and Bento-Santos 19 ). Daily food intake was determined by the difference between the amount of food (g) provided at 09.00 hours and the amount of food (g) that remained 24 h later( Reference Lopes de Souza, Orozco-Solis and Grit 20 ). The energy intake was calculated by multiplying the amount of food intake during gestation and lactation by the energetic value of both LP diet and normal protein diet( Reference Ferro Cavalcante, Lima da Silva and da Marcelino da Silva 21 ). Feed conversion (FC) was calculated as: final body weight–initial body weight/total amount of food consumed.
Offspring assessment
Somatic growth and food intake
During lactation, body weight and body length were measured at days 1st, 7th, 14th, and 21st after birth. Body weight of the pups was recorded throughout the experiment with a Marte scale with 0·001 g precision. At 21 d of age, the body length (distance from nose to anus, cm) and tail length (distance from tail tip to tail base, cm) were measured with a digital caliper (Series 799; Starrett®) with a 0·01 mm precision. Abdominal circumference (immediately anterior to the forefoot, cm) and thoracic circumference (immediately behind the foreleg, cm) were evaluated.
Offspring body weight was assessed at 30, 40, 50 and 60 d old. At 60 d old, body length, BMI and tail length were calculated. Between the 30th to 36th days, and the 54th and 60th days of life, the pups were individually housed to evaluate food intake. The first 3 d were designed for adaptation to the cage. An animal’s daily food consumption was determined by the difference between the amount of food (g) provided at the onset of the dark cycle and the amount of food (g) remaining 24 h later( Reference Lopes de Souza, Orozco-Solis and Grit 20 ). Body and food weight were recorded using the Marte Scale. The energy intake was calculated by multiplying the amount of food intake by the energetic value of both the standard chow and the HF diet. On days 33 to 36 and 57 to 60, body weight, relative food intake (g/100 g of body weight), relative energy intake (kJ/100 g (kcal/100 g) of body weight) and FC were evaluated( Reference Ferro Cavalcante, Lima da Silva and da Marcelino da Silva 21 ).
Weight of organs
The abdominal cavity was exposed and the liver, heart, spleen, epididymal and retroperitoneal fat pads were removed and weighed using a scale (Marte XL 500, Class II, capacity 500 g with 0·001 g precision) to calculate absolute weight (g) and relative weight (g/100 g of body weight).
Biochemical and hormonal assessment
After fasting (6 h), blood samples were collected and centrifuged at 3500 rpm for 10 min to obtain the serum. Serum glucose, total cholesterol and TAG levels were measured with commercially available kits (Labtest; Minas Gerais) using a spectrophotometer Genesys 10S UV-Vis (Thermo ScientificTM), following the manufacturer’s instructions. The equation of Friedewald was used to calculate the levels of VLDL-cholesterol( Reference Friedewald, Levy and Fredrickson 22 ). All analyses were performed in duplicate. The leptin was analysed by an ELISA using a commercially available kit (Leptin Rat ELISA; BioSource and Thermo Fisher Scientific) according to the manufacturer’s instruction.
Peritoneal lavage
After decapitation, an intraperitoneal injection of 20 ml of PBS was administered. Peritoneal macrophages were harvested in tubes with Roswell Park Memorial Institute (RPMI) 1640 medium (Vitrocell) supplemented with 10 % fetal calf serum and then the samples were centrifuged at 300 g for 10 min at 4°C to obtain pellet. Cells were suspended in RPMI 1640 supplemented with 10 % fetal calf serum (Cultilab). More than 98 % of the peritoneal macrophages were viable as indicated by the trypan blue exclusion test.
Phagocytosis assay
The peritoneal macrophages (1×106) were incubated for 40 min at 37°C in 1 ml RPMI 1640 with opsonized particles of zymosan (zymosan A of Saccharomyces cerevisiae – Sigma Z-4250; Sigma). The particles (1×107) were opsonized by incubation in the presence of C rat serum for 40 min at 37 °C. The phagocytic capacity was defined as the number of macrophages that internalised 3 or more zymosan particles. The results were then reported as percentage of phagocytic macrophages. At least 100 cells were counted in each coverslip( Reference Leandro, de Lima and Alba-Loureiro 23 ).
Nitric oxide assay
The peritoneal macrophages were placed in a culture plate with RPMI 1640 with 10 % fetal calf serum (FCS) (1×106/well) and were stimulated with 1 ng/ml per well of LPS (LPS from Escherichia coli – Sigma L2880; Sigma) and 5 ng/ml per well of interferon γ (IFN-γ) (IFN-γ from rat – Sigma I3275; USA). IFN-γ is the main cytokine associated with M1 activation and the main Th1 cell product( Reference Martinez and Gordon 24 ). The cells were incubated for 24 h at 37°C and 5 % CO2 to induce NO production( Reference Ferreira, Galvao and Ferraz-Pereira 7 ). NO release was measured indirectly using a quantitative colorimetric assay based on the Griess reaction( Reference Ding, Nathan and Stuehr 25 ). In the present study, duplicate 100 μl aliquots of cell culture supernatants were incubated with 100 μl of freshly prepared Griess reagent (1 % sulfanilamide in 5 % o-phosphoric acid, 0·1 % naphthylethylene diamide dihydrochloride) at room temperature for 10 min. The absorbance of the azochromophore was measured at 550 nm (Bel Photonics 1105; Tecnal,). The NO concentration was determined using sodium nitrite as a standard (0–35 μm). All samples were assayed against a blank comprising RPMI 1640 with 10 % FCS incubated for 24 h in the same plates as the samples, but in the absence of cells( Reference Ferreira, Galvao and Ferraz-Pereira 7 ). All reagents were purchased from Sigma-Aldrich. The results were expressed in micromoles of NO per 1×106 cells.
Statistical analyses
During gestation and lactation, Student’s t test was used to compare LP and C mothers. After weaning, for the analysis of the differences among groups, two-way ANOVA (LP diet and HF diet as factors) and Sidak’s post hoc test were used. Values are presented as mean values with their standard errors. Significance was set at P <0·05. Data analysis was performed using the statistical program Graphpad Prism 6® (GraphPad Software Inc.).
Results
During gestation, there were no differences between LP and C dams in terms of body weight and food intake (C=411·2 (sem 16·5) g, and LP=398·9 (sem 11·5) g; P=0·78). The %BWG was also similar for the groups (C=37·4 % and LP=38·7 %, P=0·72). At delivery, the number of pups did not differ between groups (C=8–11 pups and LP=9–12; P=0·42). Throughout lactation, LP dams showed a reduced body weight (−15·6 %), and there was a trend of reduced food intake (C=651·9 (sem 24·6) g, and LP=574·5 (sem 28·8) g; P=0·06). For both groups, energy intake was not different for dams during gestation (C=6192·32 (sem 249·87) kJ/100g (1480 (sem 59·72)kcal/100g); LP=6008·22 (sem 327·40) kJ/100g (1436 (sem 78·25) kcal/100g), P=0·68) and lactation (C=9790 (sem 454) kJ/100g (2340 (sem 108·5) kcal/100g); LP=8351 (sem 584·1) kJ/100g (1996 (sem 139·6) kcal/100g), P=0·070), but FC was reduced in LP mothers compared with C (C=−0,01±0,01; LP=−0,08±0,02, P=0,02) during lactation.
For the pups, birth weight was similar in both groups, but pups from LP mothers showed lower body weight and body length during lactation (Figs. 1(a) and (b)). The %BWG of LP pups was 45·2 % lower than in pups from the C dams. At weaning (21 d of age), the groups were divided according to the consumption of HF diet. LP pups remained lighter than C until 50 d of age, even LP pups fed on a HF diet (Fig. 2(a)). At 60 d of age, the LP-HF pups showed similar body weight but a higher % BWG than HF (HF=C=406·6 % and LP-HF=798·1 %; P=0·001). Food intake was increased for LP pups and reduced for HF pups when compared with C pups, regardless of when it was measured (Fig. 2(b)). LP-HF pups showed a higher food intake than HF pups, but it was reduced when compared with LP pups. From 33–36 d old, FC was increased in LP-HF pups when compared with LP and HF pups (Fig. 2(c)). From 57–60 d old, FC was increased for HF and LP-HF when compared with their pairs C and LP, respectively (Fig. 2(c)). However, from 33–36 d old, energy intake (kJ/100 g (kcal/100 g)) increased in the LP–HF pups when compared with the LP pups, but normalised from 57 to 60 d old. From 57 to 60 d old, LP pups showed higher energy intake than the C (Fig. 2(d)). Conversely, in the period between 21 and 60 d old, LP and LP-HF pups showed a higher %BWG than C pups (increase of 40·9 % and 50·4 %, respectively) and LP-HF presented a higher %BWG than HF (increase of 74·83 %).
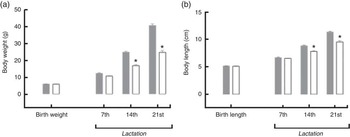
Fig. 1 Body weight (a) and body length (b) during lactation of pups from mothers fed either a low-protein () diet or control (
) diet during gestation and lactation. Values are means, with standard errors represented by vertical bars. * P<0·05 v. control, using Student’s t test.
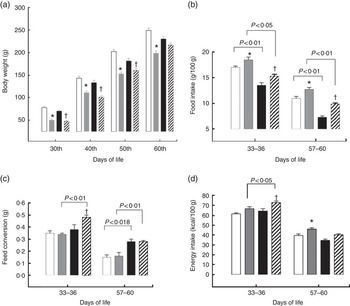
Fig. 2 Body weight (a), food intake (b), feed conversion (c) and energy intake (d) of pups fed a high-fat () diet from weaning to 60 d old. Male pups came from mothers fed either a low-protein diet (
) or control (
) diet during gestation and lactation. Values are means, with standard errors represented by vertical bars. * P<0·05 v. control, †
P<0·05 v. high-fat diet group using two-way ANOVA followed by Sidak’s post hoc test.
, Low protein–high fat. To convert kcal/100 g to kJ/100 g, multiply by 4·184.
A descriptive analysis of somatic growth, organ weight and biochemical parameters of offspring at 60 d of age is presented in Table 2. LP pups showed reduced body length, BMI and tail length. LP-HF pups showed a higher BMI and tail length than LP pups. Retroperitoneal fat weighed more in LP pups than C pups. HF and LP-HF pups showed increased weight of retroperitoneal and epididymal fat depots compared with C and LP pups. LP pups showed reduced serum glucose and total cholesterol, but increased TAG when compared with C pups. HF and LP-HF pups presented higher serum level of TAG and total cholesterol than C and LP pups. In addition, serum leptin was reduced in LP pups when compared with the C pups, but did not alter in HF and LP-HF pups (Table 2).
Table 2 Descriptive statistics of somatic indicators, organs weight and blood biochemical parameters of offspring fed a high-fat diet from weaning to 60 d old and submitted or not to a maternal low-protein diet during gestation and lactationFootnote ‡ (Mean values with their standard errors)
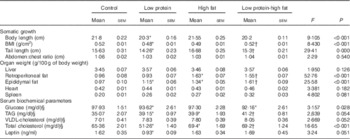
* Mean value was significantly different from that for control (two-way ANOVA followed by Sidak’s post hoc test).
† Mean value was significantly different from that for low-protein (two-way ANOVA followed by Sidak’s post hoc test).
‡ Pups were evaluated at 60 d old (n 11/each group).
§ To convert glucose in mg/dl to mmol/l, multiply by 0·0555. To convert TAG in mg/dl to mmol/l, multiply by 0·0113. To convert cholesterol in mg/dl to mmol/l, multiply by 0·0259.
Macrophages from the LP pups showed a higher phagocytosis rate than the C pups (Fig. 3). Animals that consumed a HF diet from weaning until 60th day of life, irrespective to the maternal protein restriction, showed no effects on phagocytosis rate of peritoneal macrophages. At baseline, macrophages from LP and LP-HF pups showed an increase of the NO production (Fig. 4). After LPS+IFN-γ stimulation, LP and LP-HF pups showed reduced NO production when compared with their pairs (Fig. 4).
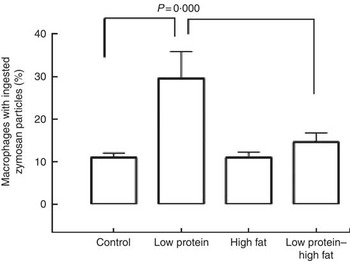
Fig. 3 Phagocytic activity of peritoneal macrophages of pups fed a high-fat diet from weaning to 60 d old. Male pups came from mothers fed either a low-protein diet or control diet during gestation and lactation. Values are means, with standard errors represented by vertical bars. Two-way ANOVA followed by Sidak’s post hoc test was used.
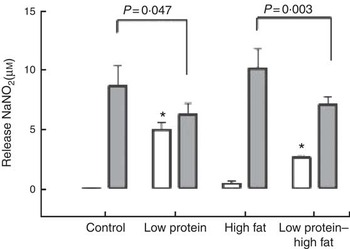
Fig. 4 Nitric oxide production (at baseline () and after stimulation with lipopolysaccharide (LPS)+interferon- γ (IFN-γ) (
)) of peritoneal macrophages of pups fed a high-fat diet from weaning to 60 d old. Male pups came from mothers fed either a low-protein diet or control diet during gestation and lactation. Values are means, with standard errors represented by vertical bars. * P<0·05 v. control, using two-way ANOVA followed by Sidak’s post hoc test.
Discussion
Previous studies have demonstrated that malnutrition occurring during early life development induces physiological and homoeostatic set points in the organism as a strategy for survival( Reference Gluckman, Hanson and Beedle 9 , Reference Fidalgo, Falcao-Tebas and Bento-Santos 19 , Reference Qasem, Yablonski and Li 26 , Reference Leandro, da Silva Ribeiro and Dos Santos 27 ). These changes are well characterised in terms of growth trajectory, alterations of food intake and metabolic disorders( Reference Fidalgo, Falcao-Tebas and Bento-Santos 19 , Reference Moita, Lustosa and Silva 28 ). Aligned with previous studies, our results demonstrated that pups submitted to maternal protein restriction during gestation and lactation showed reduced body weight, somatic growth and serum glucose, total cholesterol and leptin, and increased food intake, epididymal fat and serum TAG. Furthermore, when a HF diet was provided to LP pups, the effects on fat accumulation, food intake, TAG and total cholesterol increased, as seen in previous studies( Reference Gluckman, Hanson and Beedle 9 , Reference Vickers, Breier and Cutfield 10 , Reference Gosby, Maloney and Caterson 11 , Reference Bateson, Barker and Clutton-Brock 29 ). It can be suggested that although the organisms try to adapt to different nutritional demands, the metabolic load generated by the low lean mass and HF mass is mismatched with metabolic capacity attained during fetal life, increasing the risk of diseases in young age( Reference Wells 30 , Reference Alheiros-Lira, Araujo and Trindade 31 ).
At the end of lactation, it was observed that prolonged consumption of a LP diet resulted in low body weight and FC in LP dams, despite a similar energy intake and trend in reduced food intake. It is well established that LP intake, reduced FC and body weight gain during pregnancy/lactation are the three main factors influencing maternal and infant outcomes( Reference Alheiros-Lira, Araujo and Trindade 31 – Reference de Brito Alves, Nogueira and de Oliveira 33 ). In fact, we have demonstrated that protein restriction during gestation produces intra–uterine growth restriction and continued protein restriction during lactation counteracts catch up growth and results in long-term decreases in body weight due to a reduction in both lean and fat mass( Reference Falcao-Tebas, Bento-Santos and Fidalgo 2 , Reference de Brito Alves, Nogueira and de Oliveira 33 , Reference Pires-de-Melo, Wanderley Dos Reis and Luz 34 ). The underlying probable mechanism of the deficit in an offspring’s growth patterns can be associated to hormonal alterations, such as reduced insulin-like growth factor-1( Reference El-Khattabi, Gregoire and Remacle 35 ) concentration and growth hormone mRNA expression( Reference de Moura, Lisboa and Custodio 36 ).
At weaning, protein restricted pups were submitted to a HF diet. The LP and LP-HF pups remained lighter and smaller than their C. However, at 60 d of age, the LP-HF pups recovered their body weight, tail length and BMI. These pups also showed a two-fold increase of %BWG over their pairs fed a HF diet. A previous study verified that rats submitted to perinatal protein restriction (8 % protein) followed by a post-weaning HF diet (41 % of total energy content from fat, 42 % carbohydrates and 17 % protein) changed underwent an alteration in somatic growth indicators and increase in the %BWG( Reference Gosby, Maloney and Caterson 11 ). Our data demonstrated that the LP-HF pups showed a high FC. This represents the capacity of an animal to gain body weight as a function of the quantity of food intake (i.e. when eating the same amount of food, LP-HF animals present higher body weight gain than HF). However, the HF pups presented a significant reduction in the food intake. This reduced food intake can be attributed to the high energy density of the HF diet (19·87 v. 15·10 kJ/g (4·75 cal/g v. 3·61 kcal/g))( Reference Camargo, Batista and Ribeiro 37 ). Diets containing 30 % or more of energy derived from fat induce satiety in rodents as a result of increased energy intake and efficiency of energy storage( Reference Hill, Melanson and Wyatt 38 , Reference Hariri and Thibault 39 ). In addition, diet-induced thermogenesis is important to energetic balance regulation and may influence the food intake in HF animals( Reference Hariri and Thibault 39 ). It was interesting to observe that the reduced food intake was less pronounced in the LP-HF pups. A maternal LP diet results in reduced activation of the satiety centres and increased hypothalamic gene expression of orexigenic neuropeptides, reverberating in a higher food intake( Reference da Silva, Borba and de Almeida Lira 40 ). In offspring fed the HF diet, the expression of orexigenic neuropeptides is reduced as an adaptive mechanism to reduce the body weight gain( Reference Camargo, Batista and Ribeiro 37 , Reference Levin and Dunn-Meynell 41 ). Our finding demonstrated that perinatal protein restriction could lead to differential adaptive feeding behaviour responses to HF diet.
In the current study, measurement of serum biochemical parameters revealed that fasting produced a reduction in blood glucose, increased TAG and total cholesterol selectively in the LP offspring and thereby created a metabolic milieu that is expected for LP-HF offspring. These results are aligned with previous studies( Reference Alheiros-Lira, Araujo and Trindade 31 – Reference de Brito Alves, Nogueira and de Oliveira 33 ). In addition, our data demonstrated increased retroperitoneal and epididymal fat depots in LP and LP-HF pups, which corroborate with previous study( Reference Camargo, Batista and Ribeiro 37 ). In rats, prolonged consumption of HF diet increased adipose tissue storage and, concomitantly, blood leptin concentration( Reference Estadella, Oyama and Damaso 18 , Reference Estadella, Oyama and Bueno 42 ), whereas protein restriction decreased leptin concentration in offspring( Reference Fidalgo, Falcao-Tebas and Bento-Santos 19 , Reference Qasem, Yablonski and Li 26 ). Indeed, leptin concentration was reduced in the LP pups, but normalised in LP-HF pups probably due to the increased amount of adipose tissue.
To describe the role of macrophages in impaired host defence under maternal protein malnutrition followed by the post-weaning consumption of HF diet, we examined phagocytosis and NO production of resident peritoneal macrophages. Macrophages from the LP pups showed a higher phagocytosis rate than in the C. In contrast, previous studies have found that macrophages from undernourished animals showed reduced phagocytosis( Reference Prestes-Carneiro, Laraya and Silva 6 , Reference Morais, da Costa and Severo 43 ). Our data demonstrated that the extension of maternal protein restriction is related to the tissue inflammation that develops as the consequence of an inadequate innate immune response. In addition, this seems to be determined in a large part by the increased number of pro-inflammatory non-adipose tissue resident peritoneal macrophages. However, the mechanisms that participate in these processes are still poorly understood.
NO is a highly reactive molecule and a potent product of macrophage effector against bacteria, parasites and tumours( Reference Ferreira, Galvao and Ferraz-Pereira 7 ). Macrophages of LP and LP-HF showed increased NO production without LPS+IFN-γ stimulation. An elevated NO production has been implicated in macrophage death by apoptosis( Reference Sarih, Souvannavong and Adam 44 ) and in the pathogenesis of inflammatory diseases( Reference Borges, Vinolo and Crisma 15 ). Previous studies have shown that LP diet induces impaired production of superoxide anion and NO by resident macrophages( Reference Prestes-Carneiro, Laraya and Silva 6 , Reference Teshima, Rokutan and Takahashi 14 ). It was interesting to observe that HF diet did not blunt the phagocytosis rate of peritoneal macrophages in rats submitted to a maternal LP diet. Conversely, macrophages of LP and LP-HF pups were hyporesponsive to stimulation with LPS+IFN-γ and showed a reduced NO production. Reduced LPS+IFN-γ induced NO release is involved in reduced toxicity of macrophage and high susceptibility to bacterial infection and tumour growth( Reference Ferreira, Galvao and Ferraz-Pereira 7 ). Our data demonstrated that there exists an immunomodulation related to dietary fatty acids after the maternal LP diet-induced metabolic programming. Additional studies may consider the hypothesis that the immunomodulatory role in the potential effects of dietary unsaturated fatty acids can be applied to the amelioration of inflammatory disorders of peritoneal macrophages in young rats submitted to maternal malnutrition.
Conclusions
In this study, rats submitted to a maternal LP diet during gestation and lactation were challenged with a HF diet post weaning. As expected, the maternal LP diet altered somatic parameters of growth and development of pups. At 60 d of age, the interaction between maternal LP and post-weaning HF diet was able to recover body weight and BMI, but the pups showed double body weight gain and increased retroperitoneal and epididymal fat depots. However, food intake was reduced in pups fed a HF diet, regardless to maternal diet. It was interesting that leptin concentration was reduced in LP pups, but normalised in LP-HF pups probably due to the increased amount of adipose tissue. Phagocytic activity and NO production were increased in the peritoneal macrophage of LP pups irrespective to physiological stimulation. The post-weaning consumption of a HF diet after a period of perinatal protein restriction abolished the increased phagocytic activity of macrophages. On the other hand, the peritoneal macrophages of LP pups were hyporesponsive to LPS+IFN-γ induced NO release even after a post-weaning HF diet.
Acknowledgements
The authors would like to thank Sidney Pratt, Canadian, MAT (The Johns Hopkins University), RSAdip – TESL (Cambridge University) for the revision of the English text of this paper.
This research was supported by the Foundation to Support Science and Research in Pernambuco State – Brazil (FACEPE, APQ-0797-4.05/14), CAPES (grant no. 797/14) and the National Council for Research – Brazil (CNPq, 477915/2012-4).
The authors’ contributions are as follows: M. C. A.-L., J. C. F. and C. G. L. was the principal investigator and contributed to the study design, interpretation of the findings and data analyses and wrote the manuscript; G. C. J.-S., H. T. d.-S., S. M.-S. and W. T. F. e. S. contributed to the confection of diets, analyses of phagocytosis and NO production and carried out data analyses. All authors have read and approved the final version of the manuscript.
The authors declare that there are no conflicts of interest.