Development and optimisation of food products with appetite-suppressing properties represent a strategy to combat the increasing global prevalence of overweight and obesity( Reference Chambers, McCrickerd and Yeomans 1 , Reference Hellström 2 ). Food products affect appetite and metabolism differently depending on their content of energy and macronutrients, as well as their physiochemical attributes. Previous studies have indicated that isoenergetic intakes of macronutrients do not suppress appetite to the same magnitude; dietary fibre-rich carbohydrates and proteins have been shown to be more satiating than fats( Reference Chambers, McCrickerd and Yeomans 1 ).
Whole-grain rye food products that are naturally rich in dietary fibre, such as porridge, soft bread and crisp bread, have consistently shown appetite-suppressing effects in human studies( Reference Johansson, Lee and Risérus 3 – Reference Rosén, Östman and Shewry 14 ). Moreover, whole-grain rye foods promote tighter regulation of postprandial glucose( Reference Hartvigsen, Lærke and Overgaard 4 , Reference Hartvigsen, Gregersen and Lærke 6 , Reference Rosén, Östman and Björck 8 , Reference Rosén, Östman and Björck 10 , Reference Rosén, Silva and Andersson 11 , Reference Rosén, Östman and Shewry 14 , Reference Breen, Ryan and Gibney 15 ) and insulin concentrations( Reference Johansson, Lee and Risérus 3 , Reference Hartvigsen, Lærke and Overgaard 4 , Reference Hartvigsen, Gregersen and Lærke 6 , Reference Rosén, Östman and Björck 8 , Reference Rosén, Östman and Björck 10 , Reference Rosén, Silva and Andersson 11 , Reference Rosén, Östman and Shewry 14 , Reference Breen, Ryan and Gibney 15 ) and influence gut hormones associated with appetite, such as glucagon-like peptide 1 (GLP-1), which potentiates glucose-induced insulin secretion and slows gastric emptying( Reference Hartvigsen, Lærke and Overgaard 4 , Reference Hartvigsen, Gregersen and Lærke 6 , Reference Juntunen, Laaksonen and Autio 16 , Reference Juntunen, Niskanen and Liukkonen 17 ), leading to increased satiety( Reference Hellström 2 , Reference Näslund, Gutniak and Skogar 18 ). Appetite, metabolic and hormonal responses are believed to be affected by SCFA produced during gut fermentation of dietary fibre( Reference Hartvigsen, Lærke and Overgaard 4 , Reference Fernandes, Vogt and Wolever 19 – Reference Nilsson, Östman and Granfeldt 21 ). However, the relationship between satiety and gut fermentation is typically confounded by other aspects of dietary fibre such as volume and viscosity, and thus the role of fermentation per se is difficult to evaluate( Reference Cani, Lecourt and Dewulf 20 ).
Among macronutrients, protein is considered to be the most satiating( Reference Chambers, McCrickerd and Yeomans 1 , Reference Gilbert, Bendsen and Tremblay 22 , Reference Acheson, Blondel-Lubrano and Oguey-Araymon 23 ), although evidence is scarce on the effects of proteins from different sources, especially plant proteins, and the underlying physiochemical properties are not fully understood( Reference Gilbert, Bendsen and Tremblay 22 ). The effects of proteins on appetite are possibly mediated by diet-induced thermogenesis, secretion of gut hormones, for example, GLP-1, and stimulated secretion of insulin by absorbed amino acids( Reference Chambers, McCrickerd and Yeomans 1 , Reference Gilbert, Bendsen and Tremblay 22 ).
We hypothesised that extensive fermentation in the first part of the large intestine caused by inulin would stimulate GLP-1 secretion and promote satiety 4–8 h after ingestion. We further hypothesised that rye porridge with high protein content would lead to higher satiety compared with products with lower protein content. The primary aim of this study was therefore to investigate whether appetite-suppressing effects of whole-grain rye porridge could be enhanced by replacing part of the rye with rapidly fermented dietary fibre (inulin) and plant protein (wheat gluten). The secondary aim was to investigate the role of gut fermentation on appetite and postprandial glucose and hormonal responses up to 8 h after breakfast.
Methods
Subjects
Men and women aged 18–60 years were recruited from Uppsala, Sweden, through local advertisements. Recruitment started in March 2013, and the study was carried out until the end of August 2013 when all the enrolled subjects had completed the intervention. Screening, including measurements of anthropometrics and biomarkers in fasting blood samples, was conducted to ensure that subjects were overtly healthy. Subjects completed questionnaires regarding health, medication use, allergies, diets, tobacco use, physical activity and eating behaviour (Three-Factor Eating Questionnaire (TFEQ) in a revised version R18)( Reference Cappelleri, Bushmakin and Gerber 24 , Reference Johansson and Westerterp 25 ). Exclusion criteria were as follows: presence of diabetes, hyperglycaemia, hyperinsulinaemia, thyroid disease and metabolic issues; pregnancy, lactation or planned pregnancy; eating disorders; dieting or weight loss >10 % 3 months before screening; recent or concurrent dietary study participation; non-habitual breakfast eaters; intolerances or allergies to study foods; and heavy smokers. Only post-menopausal women or women using hormonal contraceptives were included in the study, to avoid the influence of cyclic fluctuations in hormones on eating behaviour( Reference Dye and Blundell 26 ). Before enrolment, subjects tried the test product with the highest amount of dietary fibre to prevent inclusion of subjects sensitive to foods rich in dietary fibre, with an aversion to the test product or with problems eating breakfast.
Ethics approval
This study was conducted according to the guidelines laid down in the Declaration of Helsinki, and all procedures involving human subjects were approved by the Regional Ethical Review Board, Uppsala, Sweden. Written informed consent was obtained from all subjects. The study was registered in the public trials registry ClinicalTrials.gov, ID: NCT01965210 (https://clinicaltrials.gov/ct2/show/NCT01965210).
Design
The present study was designed as a randomised, extended postprandial, single-blind, cross-over study with six breakfast meals served in random order with a wash-out period of ≥5 d in between. Subjects were not informed about the content of the breakfast meals, and were randomly assigned to a randomly generated breakfast meal sequence as they were enrolled in the study. On the morning of the study visits, subjects arrived fasted (for 12 h) to the clinic at Uppsala University Hospital, Sweden. Subjects were instructed to avoid strenuous physical activity and to exclude alcohol and dietary fibre-rich foods according to dietary guidance during the whole day before each study visit. Upon arrival, an intravenous catheter was inserted into the antecubital vein for repeated blood sampling. Capillary samples may be superior to venous antecubital venous samples for determining blood glucose concentrations and the glycaemic index of food. However, because of extensive sampling and other measurements conducted, we decided to only collect antecubital venous samples. Antecubital vein blood sampling has been widely used in several studies for measuring postprandial glucose concentrations, in designs similar to ours( Reference Maffeis, Bonadonna and Consolaro 27 – Reference Bondia-Pons, Nordlund and Mattila 29 ). Baseline measurements of appetite and breath hydrogen and methane were made (at −30 min), and blood samples were drawn (at −15 min). The prepared breakfast meal was served immediately after the second appetite rating (at 0 min). Subjects were seated together while eating and allowed to make conversation, but not regarding anything related to the study or food, and were instructed to finish the breakfast meal within 15 min. Subjects remained at the clinic and were limited to sedentary activities, for example, using a laptop or reading, and were allowed to visit the outdoor terrace area when the blood sampling schedule permitted in the late afternoon. Commercially available ready meals were served for lunch and dinner (at 240 and 480 min, respectively), as 4 h between meals was considered a realistic and appropriate time interval( Reference Blundell, de Graaf and Hulshof 30 ). Lunch was a pre-portioned standardised meal consisting of sausage Stroganoff with rice (Carolines Kök), with an average portion weight of 405 (sd 23) g (3875 kJ, protein 4 g, fat 7·1 g, available carbohydrates 15·5 g, dietary fibre 0·1 g) and one standard glass of tap water, served in the hospital restaurant. Dinner was an ad libitum meal of pasta Bolognese (Findus) and one standard glass of tap water, served in the breakfast room. Energy intake at dinner was calculated on the basis of the weight difference between food supplied and food remaining. Subjects were instructed to finish both meals within 30 min, and to eat until comfortably full at the ad libitum dinner meal. The nutrient composition of the lunch and dinner meals was based on the manufacturers’ data (online Supplementary Table S1). Subjects were not allowed to eat or drink anything that was not included in the study diet.
Test meals
The breakfast meals consisted of six isoenergetic products served as part of a complete breakfast: five whole-grain rye porridge meals and one refined wheat bread (WB) meal as reference. WB has been the common reference product in earlier appetite studies including rye, and was therefore chosen in order to facilitate comparisons( Reference Isaksson, Sundberg and Aman 13 ). The rye porridges were prepared from commercially available whole-grain rye flakes made from cut, steamed and rolled rye kernels (Lantmännen Cerealia AB). Among the five rye porridges tested, two contained 40 g of rye flakes (RP40) and 55 g of rye flakes (RP55), respectively, whereas three contained 40 g of rye flakes with 15 g of a combination of inulin (Orafti®GR inulin, purity 90 %; Beneo GmbH) and wheat gluten (Vital Wheat Gluten, purity 77 %; Arrowhead Mills Inc.). Recipes were re-calculated to compensate for impurities to provide inulin and wheat gluten in a ratio of 9:3 g (RPHI), 6:6 g (RPIG) or 3:9 g (RPHG). Inulin with an average degree of polymerisation of 10 was chosen, as it undergoes rapid gut fermentation and does not increase viscosity or bulking to the same extent as many other fermentable dietary fibres from, for example, cereals( Reference Schneeman 31 ). Sensory evaluations of isolated plant proteins from rice, potato, pea and wheat were performed. Wheat gluten was chosen as it had the most appealing flavour when added to porridge. Porridge material was stored and prepared in unlabelled opaque paper cups with lids, which concealed the content. Dry material was manually stirred thoroughly, boiling water was added, and porridge was manually stirred thoroughly and allowed to rest for 2 min while margarine (Unilever Sverige AB) was added. Porridge was again manually stirred thoroughly and allowed to rest for 2 min, and 25 g raspberry jam (Orkla Foods Sverige AB) was added on top. Porridge was served immediately after preparation. Different amounts of water were added to ensure similar viscosity of the porridges: 150 ml of water was added to the four porridges samples containing 40 g of rye flakes, whereas 200 ml of water was added to the porridge sample containing 55 g of rye flakes. The WB reference was made from 55-g commercially available refined soft WB (Pågen AB) served with margarine (Unilever Sverige AB) and 25 g raspberry jam (Orkla Foods Sverige AB). Breakfast meals also included 100 ml of milk (Arla Foods AB) and 150 ml of coffee or tea, whereas the amount of margarine was varied to make the breakfast meals isoenergetic. The ingredients of the breakfast meals are presented in Table 1.
Table 1 Ingredients, nutrient composition (g/portion) and energy (%) in the different breakfast meals tested in the study

RPHI, porridge: 40-g rye flakes inulin:wheat gluten 9:3 g; RPIG, porridge: 40-g rye flakes inulin:wheat gluten 6:6 g; RPHG, porridge: 40-g rye flakes inulin:wheat gluten 3:9 g; RP55, porridge: 55-g rye flakes; RP40, porridge: 40-g rye flakes; WB, 55-g refined wheat bread; EAA, essential amino acids; BCAA, branched-chain amino acids.
* Inulin purity 90 %.
† Wheat gluten purity 77 %.
‡ Not including content in additional food items.
§ Calculated as the sum of fructan and total dietary fibre as analysed by the Uppsala method.
|| Calculated as the sum of fructan and total extractable dietary fibre as analysed by the Uppsala method.
¶ Calculated from the sum of arabinose, xylose and galactose assuming an arabinose:extractable galactose ratio of 0·69 in arabinogalactan.
Chemical analysis
Prepared porridges and bread were freeze-dried and homogenised in an ultra-centrifugal mill (ZM-1; Retsch GmbH). Crude fat content was determined by method B in Commission Directive 98/64/EC( 32 ), and crude protein content was determined by the Kjeldahl method with a conversion factor of 6·25( 33 ) at Kungsängen Laboratory, Uppsala, Sweden. Dietary fibre (extractable and unextractable) and total glucose were determined by the Uppsala method( Reference Theander, Aman and Westerlund 34 ). Resistant starch, fructan and β-glucan contents were determined using assay kits K-RSTAR( Reference McCleary and Monaghan 35 ), K-FRUC( Reference McCleary, Murphy and Mugford 36 ) and K-BGLU( Reference McCleary and Codd 37 ), respectively (Megazyme). Total content of extractable dietary fibre was calculated as the sum of extractable dietary fibre and fructan. Cellulose content was calculated by subtracting resistant starch and β-glucan content from total glucose. Arabinogalactan content was calculated as the sum of galactose and arabinose content that is part of arabinogalactan( Reference Loosveld, Grobet and Delcour 38 ). Arabinoxylan content was calculated as the sum of xylose and galactose, after correcting for arabinose assuming an arabinose:galactose ratio of 0·69 in extractable arabinogalactan( Reference Loosveld, Grobet and Delcour 38 ). Available starch/carbohydrate content was calculated by the difference, that is, by subtracting water, protein, fat, ash and total dietary fibre from total dry weight. The composition of total amino acids was determined by the EN ISO 13903:2005 and EN ISO 13904:2005 methods as described by Johansson et al. ( Reference Johansson, Lee and Risérus 39 ) at a certified commercial testing laboratory (Eurofins AB). The nutrient composition of additional breakfast foods was based on manufacturers’ data. Energy was calculated using standard food energy conversion factors: protein and available carbohydrates 17 kJ/g, fat 37 kJ/g and dietary fibre 8 kJ/g. The nutrient composition and energy content of breakfast meals are presented in Table 1, whereas the amino acid composition of products is presented in the online Supplementary Table S2.
Appetite ratings
Subjective feelings of appetite (hunger, fullness and desire to eat) were assessed by asking the following three questions on a unipolar visual analogue scale (VAS): (1) how hungry do you feel right now? (not at all hungry/extremely hungry), (2) how full do you feel right now? (not at all full/extremely full), (3) how strong is your desire to eat right now? (not at all strong/extremely strong). The questions were presented in sequence on a hand-held Palm computer (Palm z22; Palm Inc.) using a specially designed programme that is comparable with conventional paper 100-mm VAS( Reference Whybrow, Stephen and Stubbs 40 ). Subjects indicated their answers by making a vertical line along the scale shown on the touch screen, which was translated to a value between 0 and 100. An alarm signalled each appetite rating, and it was not possible to refer to previous ratings. Subjects indicated appetite ratings on paper 100-mm VAS when the hand-held computer was not functioning properly. Appetite rating VAS were completed eighteen times during the day starting before breakfast at −30 and 0 min and continued after breakfast at +30, +60, +90, +120, +150, +180, +210, +240, +270, +300, +330, +360, +390, +420, +450 and +480 min.
Breath hydrogen and methane measurements
Excretion of hydrogen and methane via breath was measured as indicators of gut fermentation( Reference Rumessen 41 ). After calibration with a reference gas mixture, exhaled breath was collected in a special collection system (AlveoSampler; QuinTron Instrument Company Inc.), and a minimum of 20 ml of each breath sample was analysed using a breath hydrogen and methane analyser (QuinTron BreathTracker DP; QuinTron Instrument Company Inc.). Measurements were taken directly after completion of appetite ratings and were made ten times during the day starting before breakfast at −30 min and continued after breakfast at +30, +90, +150, +210, +270, +330, +390, +450 and +480 min.
Blood collection and measurements
Venous blood samples were collected by trained nurses into ice-cold vacutainer® tubes: two plasma tubes prepared with potassium EDTA and one plasma tube with lithium heparin (Sarstedt AG & Co.). Immediately after sample collection, a protease inhibitor cocktail (160 µl) was added to the EDTA tubes to prevent degradation of GLP-1( Reference Kim, Rhee and Kim 42 ). The inhibitor cocktail was prepared daily by dissolving 1 SIGMAFAST™ Protease Inhibitor tablet (catalogue no. S8820; Sigma-Aldrich Co.) in 2·2 ml H2O containing 5·5 µl 10 mm-dipeptidyl peptidase-IV inhibitor KR-62436 (catalogue no. K4264; Sigma-Aldrich) in dimethyl sulfoxide (Sigma-Aldrich). The mixture was vortexed until transparent and kept on ice. Blood samples were drawn twelve times during the day starting before breakfast at −15 min and continuing after breakfast at +15, +35, +65, +95, +125, +185, +230, +275, +305, +365 and +470 min. On each occasion, 10 ml of blood was collected (in total 120 ml) and kept on ice. Plasma was separated from erythrocytes and buffy coat by centrifugation at 4°C for 10 min at 2000 g , and aliquoted into 2·0-ml screw-cap microtubes (Sarstedt AG & Co.). Samples were initially stored at −20°C for a maximum of 1 week and then stored at −80°C until analysed. EDTA plasma samples were analysed for glucose (Architect c16000; Abbott Laboratories) and insulin (Cobas™ C8000 e602 analyser; Roche Diagnostics GmbH) at the certified laboratory of the Department of Clinical Chemistry at Uppsala University Hospital. The CV were <4 and <3 %, respectively. Total GLP-1 (7–36 and 9–36) was analysed in EDTA plasma samples using the Multi Species GLP-1 Total ELISA kit( Reference Bak, Wewer Albrechtsen and Pedersen 43 ) (EZGLP1T-36K; EMD Millipore) in order to investigate the potential impact of gut fermentation on GLP-1 after intake of RPHI, RP55 and WB. Kits with the same lot number were used in the analysis and the sensitivity limit of the kit, as provided by the manufacturer, was 1·5 pmol/l total GLP-1 (50 µl sample size). Samples from seven subjects were, however, excluded from the GLP-1 analysis because of lack of inhibitor cocktail added during sampling. The approximate assay range was 4·12–1000 pmol/l. The intra- and inter-assay CV were <10 and <15 %, respectively.
Statistical analysis
We used two ANCOVA models to evaluate differences between breakfast meals (referred to as ‘treatments’) in the following response variables: hunger, fullness, desire to eat, hydrogen, methane, glucose, insulin, GLP-1 and ad libitum food intake, using PROC MIXED in SAS (version 9.3; SAS Institute Inc.). In the first model, the effect of treatment (RPHI, RPIG, RPHG, RP55, RP40, WB), time, sex, occasion, treatment×time, treatment×sex and treatment×occasion were included as independent variables. Subject was entered as a random effect variable, and the baseline value was entered as a covariate. Interaction terms were removed from the final models if they were not statistically significant. Differences in response variables were tested for different time intervals for hunger, fullness and desire to eat: whole day (0–480 min), after breakfast (0–120 min), before lunch (0–240 min) and after lunch (270–480 min); hydrogen and methane: whole day (−30 to 480 min); and glucose, insulin and GLP-1: whole day (−15 to 470 min), after breakfast (−15 to 125 min), before lunch (−15 to 230 min) and after lunch (275 to 470 min).
In the second model, AUC over time was calculated for each subject and response variables by the trapezoidal rule, using the same independent variables (except for time and treatment×time) and covariates as in the first model. Differences in response variables were tested for different AUC time intervals for hunger, fullness, desire to eat: whole day (AUC0–480 min), before lunch (AUC0–240 min) and after lunch (AUC270–480 min); hydrogen and methane: whole day (AUC0–480 min); and glucose, insulin and GLP-1: whole day (AUC15–470 min), after breakfast (AUC15–125 min), before lunch (AUC15–230 min) and after lunch (AUC275–470 min). The within-subject correlation between hydrogen, appetite and blood glucose after lunch was investigated by regression analysis according to the method of Bland & Altman( Reference Bland and Altman 44 ). Spearman’s correlation coefficient was used to study the relationship of breath hydrogen and dietary fibre in diets. Unless otherwise indicated, data presented are model-adjusted least-squares means with standard error of the mean, and missing values were imputed before analysis. All probability (P) values presented were Bonferroni corrected to take multiple comparisons into account, and the results were considered statistically significant at P<0·05. A total of eighteen subjects is sufficient to detect a 10 % difference in appetite ratings in different conditions, as well as glucose response, between two treatments at a power of 80 % and a level of significance of P<0·05 using a paired design( Reference Brouns, Bjorck and Frayn 45 , Reference Flint, Raben and Blundell 46 ).
Results
Subject characteristics
A total of forty-two subjects were initially screened, and twenty-five were enrolled into the study. Among them, twenty-one healthy subjects (eleven men and ten women) with a mean age of 38·6 (sd 11·8) years, range 23–60 years, and BMI of 24·9 (sd 3·3) kg/m2, range 21–33 kg/m2, completed the study (Table 2); four subjects discontinued the study due to lack of time. A study process flow chart is presented in Fig. 1. All women used hormonal contraceptives, except two who were post-menopausal. One subject was prescribed antibiotics for medical reasons during one of the test occasions and was included in the data analysis, as excluding the subject from the analysis did not change the results. One subject reported smoking one to two cigarettes per day, but abstained during visit days. Three subjects were prescribed selective serotonin re-uptake inhibitors. All except one scored within the normal range of the TFEQ and were included in the data analysis. The subject scoring above the cut-off on emotional eating was included in the data analysis, as excluding the subject from the analysis did not change the results. The analysis of subjective appetite, ad libitum food intake, glucose and insulin included twenty-one subjects, the analysis of breath hydrogen and methane included seventeen subjects, and the analysis of total GLP-1 included fourteen subjects.
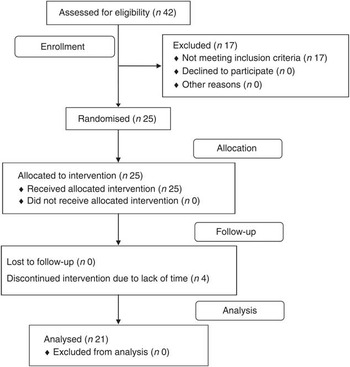
Fig. 1 Flow chart of the study process.
Table 2 Characteristics of the study subjects (Mean values and standard deviations)

Appetite ratings and food intake
In the fasted state before breakfast, no differences between hunger, fullness and desire to eat were observed between breakfast meals. Subjects reported lower hunger during the whole day (0–480 min) after consumption of the 55-g rye porridge meals (except RPHG) compared with WB and for RP55 compared with RP40 (P<0·05). Similarly, for AUC0–480 min, intake of the 55-g rye porridge meals (except RPHG) resulted in, on average, 16 % (P<0·05) lower hunger compared with WB. Hunger was 20 % (P<0·05) lower for AUC0–240 min after consumption of RP55 and RPHI compared with WB (Fig. 2).

Fig. 2 (A) Mean subjective ratings of hunger (n 21) during the whole day (0–480 min) after intake of six isoenergetic breakfast meals. Subjects reported less hunger during the whole day after intake of RPHI, RPIG and RP55 compared with WB, and RP55 compared with RP40 (P<0·05). (B) AUC for subjective hunger (n 21) before breakfast (0–120 min), before lunch (0–240 min), after lunch (270–480 min) and during the whole day (0–480 min) after intake of six isoenergetic breakfast meals. a,b Mean values with unlike letters were significantly different (P<0·05). Analysed by ANCOVA. RP40, porridge: 40-g rye flakes; RP55, porridge: 55-g rye flakes; RPHG, porridge: 40-g rye flakes inulin:wheat gluten 3:9 g; RPHI, porridge: 40-g rye flakes inulin:wheat gluten 9:3 g; RPIG, porridge: 40-g rye flakes inulin:wheat gluten 6:6 g; WB, 55-g refined wheat bread. A: , RPHI;
, RPIG;
, RPHG;
, RP55;
, RP40;
, WB. B:
, RPHI;
, RPIG;
, RPHG;
, RP55;
, RP40;
, WB.
Subjects reported higher fullness before lunch (0–240 min) and also during the whole day (0–480 min) after consumption of RPHI compared with WB (P<0·05). Fullness was on average 18 % (P<0·05) higher for AUC0–480 min and 29 % (P<0·05) higher for AUC0–240 min after intake of the 55-g rye porridge meals (except RPHG) compared with WB. Likewise, for AUC0–120 min, consumption of the 55-g rye porridge meals (except RPHI) resulted in, on average, 25 % (P<0·05) higher fullness compared with WB (Fig. 3).

Fig. 3 (A) Mean subjective ratings of fullness (n 21) during the whole day (0–480 min) after intake of six isoenergetic breakfast meals. Subjects reported higher fullness during the whole day and before lunch from intake of RPHI compared with WB (P<0·05). (B) AUC for subjective fullness (n 21) after breakfast (0–120 min), before lunch (0–240 min), after lunch (270–480 min) and during the whole day (0–480 min) after intake of six isoenergetic breakfast meals. a,b Mean values with unlike letters were significantly different (P<0·05). Analysed by ANCOVA. RP40, porridge: 40-g rye flakes; RP55, porridge: 55-g rye flakes; RPHG, porridge: 40-g rye flakes inulin:wheat gluten 3:9 g; RPHI, porridge: 40-g rye flakes inulin:wheat gluten 9:3 g; RPIG, porridge: 40-g rye flakes inulin:wheat gluten 6:6 g; WB, 55-g refined wheat bread. A: , RPHI;
, RPIG;
, RPHG;
, RP55;
, RP40;
, WB. B:
, RPHI;
, RPIG;
, RPHG;
, RP55;
, RP40;
, WB.
Subjects reported lower desire to eat before lunch (0–240 min) after consumption of RPHI compared with RP40 (P<0·05). Similarly, for AUC0–240 min, intake of RPHI resulted in 25 % (P<0·01) lower desire to eat compared with RP40 and 22 % (P<0·05) lower desire to eat compared with WB (Fig. 4).

Fig. 4 (A) Mean subjective ratings of desire to eat (n 21) during the whole day (0–480 min) after intake of isoenergetic breakfast meals. Subjects reported less desire to eat before lunch from intake of RPHI compared with RP40 (P<0·05). (B) AUC for subjective desire to eat (n 21) after breakfast (0–120 min), before lunch (0–240 min), after lunch (270–480 min) and during the whole day (0–480 min) after intake of six isoenergetic breakfast meals. a,b Mean values with unlike letters were significantly different (P<0·05). Analysed by ANCOVA. RP40, porridge: 40-g rye flakes; RP55, porridge: 55-g rye flakes; RPHG, porridge: 40-g rye flakes inulin:wheat gluten 3:9 g; RPHI, porridge: 40-g rye flakes inulin:wheat gluten 9:3 g; RPIG, porridge: 40-g rye flakes inulin:wheat gluten 6:6 g; WB, 55-g refined wheat bread. A: , RPHI;
, RPIG;
, RPHG;
, RP55;
, RP40;
, WB. B:
, RPHI;
, RPIG;
, RPHG;
, RP55;
, RP40;
, WB.
Overall, subjective ratings of hunger, fullness and desire to eat did not differ between men and women, and no differences in food intake at the ad libitum dinner 8 h after breakfast were observed between breakfast meals (online Supplementary Table S3).
Breath hydrogen and methane concentrations
The concentration of hydrogen in breath was higher during the whole day (−30 to 480 min) after consumption of the 55-g rye porridge meals compared with WB (P<0·01) and for RPHI and RPIG compared with RP40 (P<0·001). Similarly, for AUC0–480 min, intake of the 55-g rye porridge meals resulted in, on average, 3-fold (P<0·0001) higher breath hydrogen concentrations compared with WB. Intake of RPHI and RPIG displayed, on average, 46 % (P<0·05) and 102 % (P<0·0001) higher concentrations of hydrogen in breath compared with RP55 and RP40, respectively. Hydrogen concentrations were also 43 % (P<0·05), 48 % (P<0·0001) and 105 % (P<0·05) higher after consumption of RPIG compared with RPHG, RP55 and RP40, respectively (Fig. 5).

Fig. 5 (A) Mean concentrations of hydrogen in breath (n 17) during the whole day (−30 to 480 min) after intake of six isoenergetic breakfast meals. The concentration was higher during the whole day for RPHI, RPIG, RPHG and RP55 compared with WB (P<0·01), and for RPHI and RPIG compared with RP40 (P<0·001 for both comparisons). (B) AUC for hydrogen in breath (n 17) during the whole day (0–480 min) after intake of six isoenergetic breakfast meals. a,b,c,d Mean values with unlike letters were significantly different (P<0·05). Analysed by ANCOVA. RP40, porridge: 40-g rye flakes; RP55, porridge: 55-g rye flakes; RPHG, porridge: 40-g rye flakes inulin:wheat gluten 3:9 g; RPHI, porridge: 40-g rye flakes inulin:wheat gluten 9:3 g; RPIG, porridge: 40-g rye flakes inulin:wheat gluten 6:6 g; WB, 55-g refined wheat bread; ppm, parts per million. A: , RPHI;
, RPIG;
, RPHG;
, RP55;
, RP40;
, WB. B:
, RPHI;
, RPIG;
, RPHG;
, RP55;
, RP40;
, WB.
The concentration of methane excreted in breath was higher during the whole day (−30 to 480 min) after consumption of RPHI, RP55 and RP40 compared with RPHG (P<0·05) and WB (P<0·01). For AUC0–480 min, intake of RP55 resulted in 57 % (P<0·05) higher breath methane concentrations compared with WB (online Supplementary Fig. S1). Overall, the levels of hydrogen and methane excreted in breath did not differ between men and women.
Glucose
Postprandial plasma glucose concentration after breakfast (−15 to 125 min) was higher after consumption of RP55 compared with RPHI and RP40 and before lunch (−15 to 230 min) compared with WB (P<0·05) (Fig. 6). Similarly, for AUC−15 to 125 min, intake of RP55 resulted in, on average, 7 % (P<0·05) higher plasma glucose levels compared with the other rye porridge meals (except RPIG). After lunch (275–470 min), on the other hand, the postprandial blood glucose response was lower after consumption of RPHI and RP55 compared with WB (P<0·01 and P<0·05, respectively). The plasma glucose AUC275–470 min was, on average, 6 % (P<0·05) lower after intake of the 55-g rye porridge meals compared with WB (Fig. 6). Overall, there was no difference in postprandial blood glucose response between men and women.

Fig. 6 (A) Mean concentrations of plasma glucose (n 21) during the whole day (0–470 min) after intake of six isoenergetic breakfast meals. The concentration was higher from intake of RP55 compared with RPHI and RP40 after breakfast (−15 to 125 min) and before lunch (−15 to 230 min) compared with WB (P<0·05). (B) AUC for plasma glucose concentration (n 21) after breakfast (−15 to 125 min), before lunch (−15 to 230 min), after lunch (275–470 min) and during the whole day (−15 to 470 min) after intake of six isoenergetic breakfast meals. a,b Mean values with unlike letters were significantly different (P<0·05). Analysed by ANCOVA. RP40, porridge: 40-g rye flakes; RP55, porridge: 55-g rye flakes; RPHG, porridge: 40-g rye flakes inulin:wheat gluten 3:9 g; RPHI, porridge: 40-g rye flakes inulin:wheat gluten 9:3 g; RPIG, porridge: 40-g rye flakes inulin:wheat gluten 6:6 g; WB, 55-g refined wheat bread. A: , RPHI;
, RPIG;
, RPHG;
, RP55;
, RP40;
, WB. B:
, RPHI;
, RPIG;
, RPHG;
, RP55;
, RP40;
, WB.
Insulin and glucagon-like peptide-1
There were no differences in insulin or total GLP-1 concentrations between breakfast meals, or between men and women, irrespective of time interval and statistical model (online Supplementary Fig. S2–S3).
Correlations
No correlation was found between breath hydrogen (−30 to 480 min) and appetite after lunch (270–480 min). Breath hydrogen (−30 to 480 min) was positively correlated with total dietary fibre content (R 2 0·71; P<0·0001) but inversely correlated with plasma glucose after lunch (275–470 min) (R 2 −0·18; P<0·0001).
Discussion
High-fibre rye foods have consistently shown appetite-suppressing effects and reduced postprandial insulin and sometimes reduced glucose responses compared with refined wheat( Reference Johansson, Lee and Risérus 3 – Reference Isaksson, Sundberg and Aman 13 , Reference Breen, Ryan and Gibney 15 ). In the present study, we investigated whether the appetite-suppressing effects of whole-grain rye porridge could be enhanced by replacing part of the rye with the rapidly fermented dietary fibre inulin and the plant protein wheat gluten. We also explored the role of gut fermentation on appetite, postprandial glucose, insulin and total GLP-1 concentrations during 8 h after intake of the breakfast meals.
In agreement with previous studies( Reference Johansson, Lee and Risérus 3 – Reference Isaksson, Sundberg and Aman 13 ), we found that whole-grain rye porridge lowered hunger, increased fullness and lowered desire to eat compared with WB. However, no enhancement of satiety was observed after replacing part of the rye with inulin and wheat gluten. Protein-rich meals are thought to be effective in increasing satiety under isoenergetic conditions( Reference Chambers, McCrickerd and Yeomans 1 ). However, RPHG did not suppress appetite more than the other breakfast meals. In a study by Lang et al. ( Reference Lang, Bellisle and Oppert 47 ), no differences in appetite were found between proteins from various sources (wheat gluten included), although the food products tested were isoenergetic and matched for macronutrient content. In a mixed meal, the co-ingestion of carbohydrates and fats may decrease the effect of protein on appetite( Reference Lang, Bellisle and Oppert 47 ). In addition, we observed differences in branched-chain amino acids (BCAA) between the diets. These differences attributed to the addition of wheat protein (online Supplementary Table S2). Mechanisms explaining protein-dependent satiety may be related to BCAA in circulation, through effects on satiety hormone excretion, such as GLP-1. However, RPHG contained 3-fold more gluten than RPHI, causing a 2-fold difference in BCAA, but without additional effects on satiety. Thus, observed differences in appetite response are possibly more related to the dietary fibre component of the whole-grain rye porridges.
An effect of portion size on appetite was found when comparing the whole-grain rye porridge meals with each other. The larger 55-g rye porridges with or without inulin and wheat gluten increased satiety more than the small 40-g rye porridge, and the effect was strongest during the period before lunch (0–240 min). The importance of portion size was demonstrated by Isaksson( Reference Isaksson 48 ): inclusion of 55–66-g rye per portion porridge lowered appetite, whereas 45-g rye per portion bread did not, in comparison with WB. In contrast, Forsberg et al. ( Reference Forsberg, Åman and Landberg 5 ) showed less pronounced effects on appetite following intake of 80 g instead of 64-g rye crisp bread compared with WB. The larger breakfast portion may have attenuated the satiety response and affected the subsequent ad libitum food intake at lunch( Reference Forsberg, Åman and Landberg 5 ). With no difference in appetite response between the small rye porridge and the WB, it appears that more than 40 g of rye per portion of porridge is needed to significantly increase satiety.
In general, the effects on appetite in our study were weaker after consumption of a standardised lunch meal. Similarly, Isaksson et al. ( Reference Isaksson, Tillander and Andersson 7 ) did not observe any difference in appetite between 55-g whole-grain rye porridge and WB, served as part of complete breakfasts, after intake of a standardised lunch meal, although rye porridge increased satiety before lunch and elicited an increase in breath hydrogen of similar magnitude as we observed from RP55. Because of its size, the standardised lunch meal probably attenuated any differences in appetite after lunch (275–470 min), which may explain why there was no difference in subsequent ad libitum dinner intake.
Previous studies suggest that the satiating effect of dietary fibre may partly be due to increased gut fermentation and the production of SCFA( Reference Cani, Lecourt and Dewulf 20 ). We observed an early, large and dose-dependent increase in breath hydrogen in response to the amount of dietary fibre in the breakfast meals. In contrast, breath methane did not increase in a dose-dependent manner, which could be due to low presence of a methanogenic microflora( Reference Rumessen 41 ). Hartvigsen et al. ( Reference Hartvigsen, Lærke and Overgaard 4 ) observed increased breath hydrogen and SCFA concentrations, and less hunger after lunch, after intake of a semolina porridge with added rye kernels and concentrated arabinoxylans compared with a semolina porridge. Concentrated and soluble arabinoxylans are rapidly fermented in the gut, similarly to inulin( Reference Hartvigsen, Lærke and Overgaard 4 , Reference Cani, Lecourt and Dewulf 20 ), and inulin has previously shown promising satiating effects( Reference Cani, Lecourt and Dewulf 20 ). Surprisingly, we observed no enhancement of satiety on replacing part of the rye with inulin and wheat gluten in the whole-grain rye porridge, even though extensive gut fermentation occurred 4–8 h after intake of the breakfast meals.
Fermentable dietary fibre is also important in the control of postprandial glucose response, as SCFA may enter the circulation and reduce hepatic glucose production and circulating NEFA levels, thereby increasing glucose storage and insulin sensitivity( Reference Nilsson, Östman and Granfeldt 21 , Reference Russell, Baka and Björck 49 ). The early and extensive gut fermentation that occurred after consumption of the 55-g rye porridges could explain why glucose responses after lunch (275–470 min) were lower compared with WB – that is, demonstrating a second meal effect from whole-grain rye porridge. This is supported by our observation and that of others( Reference Hartvigsen, Lærke and Overgaard 4 , Reference Nilsson, Östman and Granfeldt 21 ) of a negative correlation between breath hydrogen and plasma glucose after intake of whole-grain rye. In contrast to our results, Hartvigsen et al. ( Reference Hartvigsen, Lærke and Overgaard 4 ) did not find a second meal effect on plasma glucose after consumption of semolina porridge with added rye kernels with or without concentrated arabinoxylans. A reason for the diverging results could be that the magnitude of breath hydrogen from consumption of the 55-g porridge meals with added inulin and wheat gluten in the present study was almost twice as that reported by Hartvigsen et al. ( Reference Hartvigsen, Lærke and Overgaard 4 ). The effect of gut fermentation on second meal glucose response from intake of whole-grain rye and different amounts of rapidly fermented dietary fibre warrants further investigation.
Even though there was a clear meal response in glucose concentration, we did not detect any differences in postprandial insulin response between the breakfast meals. Lower insulin levels have commonly been observed in previous studies on rye bread( Reference Hartvigsen, Gregersen and Lærke 6 , Reference Rosén, Östman and Björck 10 , Reference Rosén, Silva and Andersson 11 , Reference Juntunen, Laaksonen and Autio 16 , Reference Juntunen, Niskanen and Liukkonen 17 , Reference Leinonen, Liukkonen and Poutanen 50 ), rye flour porridge( Reference Rosén, Silva and Andersson 11 ), rye kernel porridge( Reference Hartvigsen, Lærke and Overgaard 4 ) and boiled rye kernels( Reference Rosén, Östman and Björck 10 ). The lack of difference in insulin response was accompanied by a lack of difference in the total GLP-1 concentrations between breakfast meals. Despite the whole-grain rye porridges having a disintegrated structure( Reference Vázquez-Gutiérrez, Johansson and Langton 51 ) and giving rise to extensive gut fermentation, no differences were observed in GLP-1. Previous studies on the effects of whole-grain rye foods on GLP-1 have reported mixed results( Reference Hartvigsen, Lærke and Overgaard 4 , Reference Hartvigsen, Gregersen and Lærke 6 , Reference Juntunen, Laaksonen and Autio 16 , Reference Juntunen, Niskanen and Liukkonen 17 ). GLP-1 is released in response to the amount of macronutrients ingested, mainly glucose and lipids( Reference Hellström 2 , Reference Brubaker and Anini 52 , Reference Holst 53 ). Thus, the lack of difference in GLP-1 levels could be due to the added fat( Reference Gibbons, Caudwell and Finlayson 54 ) or nutrients provided through the co-ingestion of jam and milk. Our results indicate the importance of evaluating postprandial effects on appetite and metabolism in a mixed meal context before evaluating possible implications for health.
Our study has several strengths, but also some limitations that need to be addressed. We cannot attribute any independent effects to single nutrients, as we evaluated mixed meals. Moreover, the amount of available carbohydrates varied in the breakfast meals, which could explain why RP55 showed significantly higher plasma glucose concentration after breakfast (−15 to 125 min) compared with the other breakfast meals. As we did not analyse SCFA, we cannot be certain that the extensive breath hydrogen excretion also resulted in higher SCFA production, although a link has previously been shown after intake of whole-grain rye( Reference Hartvigsen, Lærke and Overgaard 4 , Reference Ibrügger, Vigsnæs and Blennow 55 ). As the standardised lunch meal most likely obscured the effects by gut fermentation on appetite, the hypothesis that increased gut fermentation is associated with decreased appetite needs to be further addressed in extended postprandial studies. Finally, we only analysed total GLP-1 in a subset of subjects (n 14). As gut fermentation is believed to stimulate GLP-1 production, we chose to compare breakfast meals containing high (RPHI), medium (RP55) and low (WB) amounts of fermentable dietary fibre.
In conclusion, no further increase in satiety was observed when replacing part of the rye with inulin and wheat gluten, thereby increasing the content of dietary fibre and protein. Evidently, intake of whole-grain rye porridge leads to a dose-dependent increase in gut fermentation, and an attenuated plasma glucose concentration after a second meal, but without a corresponding reduction in insulin and GLP-1 response. Further studies are needed to establish causality between appetite, gut fermentation and hormonal responses.
Acknowledgements
The authors thank the subjects for participating in this study; Gunnel Fransson for chemical analysis of the food products; and Dr Pleunie Hogenkamp for valuable discussions on study set-up.
The study was supported by the BarleyFunFood research programme at the Faculty of Natural Resources and Agricultural Sciences, Swedish University of Agricultural Sciences and Lantmännen Research Foundation. The whole-grain rye porridges were provided by Lantmännen. Lantmännen had no role in the design, analysis or writing of this article.
The authors’ contributions were as follows: I. L., U. R. and R. L. designed the study; I. L. enrolled subjects, assigned subjects to interventions, conducted the study and wrote the paper; L. S. and R. L. analysed the data; L. S. revised and re-submitted the manuscript. D.-L. W. and P. M. H. provided essential materials; R. L. generated the random allocation sequence and had primary responsibility for the final content. All authors read and approved the final manuscript.
None of the authors has any personal or financial conflicts of interest.
Supplementary material
For supplementary material/s referred to in this article, please visit https://doi.org/10.1017/S0007114516004153