Highlights
EWH protects rtWAT against damage induced in the DOCA-salt hypertensive model.
EWH restores the oxidative stress imbalance caused by DOCA-salt hypertension.
EWH reduces inflammation and apoptosis in WAT of DOCA-salt hypertensive model.
Arterial hypertension (HT) is a major global health problem associated with several complications, causing 9·4 million deaths annually. In most cases, HT’s aetiology is unknown and referred to as primary or essential. This type of HT has been related to a multifactorial chronic disease linked to genetic, environmental, and dietary factors(Reference Basting and Lazartigues1). Secondary HT has a known cause, and although it affects only 5–10 % of hypertensive patients, it usually occurs with greater severity, and the treatment often does not lead to arterial blood pressure normalisation due to the high prevalence of underlying metabolic injury or established vascular endothelial damage. Moreover, the involvement of different systems in the development and maintenance of HT, including adipose tissue metabolic insult, interferes and makes therapeutic success challenging for this pathology(Reference Al Ghorani, Götzinger and Böhm2).
White adipose tissue (WAT) is an essential and complex endocrine-metabolic regulator(Reference Parra-Peralbo, Talamillo and Barrio3) that acts on homeostasis by controlling energy balance(Reference Zeng, Pirzgalska and Pereira4), insulin resistance(Reference Maliszewska and Kretowski5), immune response(Reference Michailidou, Gomez-Salazar and Alexaki6), reproductive function(Reference Mathew, Castracane and Mantzoros7), and blood pressure(Reference Hu, Garcia-Barrio and Jiang8). WAT is mainly distributed in the visceral, subcutaneous, inguinal and retroperitoneal white adipose tissue (rtWAT) regions. Dysfunctions induced in visceral WAT, as occurs in obesity, are closely related to the development of CVD such as HT(Reference Rana and Neeland9). WAT dysfunctions in HT models are related to angiotensin receptor type 1-dependent angiotensin II elevation(Reference Giacchetti, Sechi and Griffin10) and alter cardiovascular health through the activation of oxidative and inflammatory markers such as reactive oxygen species (ROS) derived from nicotinamide adenine dinucleotide phosphate oxidase (NADPH) oxidase and nuclear factor kappa B (NFκB)-dependent IL-6(Reference Mandana Pahlavani and Ramalingam11). The adipocytes also play a fundamental role in the regulation and maintenance of HT through adipokine release and aldosterone production(Reference Borghi, Sevá-Pessôa and Grassi-Kassisse12). Moreover, most functional changes of perivascular adipose tissue (PVAT) are associated with the regulation of vascular tone through its anti-contractile action, and in consequence, alterations in PVAT are also involved in the aetiology of HT(Reference Saxton, Clark and Withers13). Pathophysiological WAT alteration involves changes in the secretory profile of adipokines and may promote an oxidative and inflammatory profile with mitochondrial redox imbalance and proinflammatory factor release(Reference Zhou, Ruan and Kong14). Despite knowing about these changes, only some studies have investigated the role of WAT in pathophysiological conditions such as severe HT. Although the cardioprotective role of brown adipose tissue has already been described, the mechanisms involved in the role of WAT have not been fully understood, especially their role as a catabolic mechanism in the severe HT model(Reference Zhu, Glazier and Hinkel15).
Considering the severity of secondary HT, their complexity, and the damage caused by WAT, novel therapeutic strategies are being directed to promote protective cardiovascular and metabolic health benefits(Reference Asgary, Rastqar and Keshvari16). Functional foods constitute a class of foods that, besides their basic nutritional properties, have natural bioactive compounds. These compounds have been used as complementary strategies in the management of HT because they do not exhibit side effects and have a low cost of adherence(Reference Asgary, Rastqar and Keshvari16). Bioactive compounds from animal sources, particularly bioactive peptides, have been extensively studied. In this context, an egg white hydrolysate (EWH) produced by treatment with pepsin for 8 hours has demonstrated antioxidant, anti-inflammatory and/or antihypertensive properties in several experimental models such as Zucker obesity(Reference Requena, Miguel and Garcés-Rimón17), spontaneously hypertensive rat(Reference Lee, Jung and Jo18), metabolic syndrome(Reference Moreno-Fernández, Garcés-Rimón and González19) and deoxycorticosterone acetate (DOCA)-salt hypertension(Reference Abreu, Rodrigues Moro and Hassan Husein Kanaan20) related to the action of bioactive peptides which are included on EWH.
In line with the search for alternative dietary strategies in the management of severe HT and the importance of the role of adipose tissue in cardiometabolic regulation and the beneficial effects caused by EWH on cardiometabolic complications, this study aims to investigate whether (a) the treatment with EWH reduces the damage induced by WAT in an experimental model of severe HT, (b) these protective actions of WAT reduce HT and related complications, and (c) the underlying mechanisms involved in both situations.
Material and methods
Preparation of egg white hydrolysate
EWH was obtained by enzymatic hydrolysis of pasteurised egg white with pepsin for 8 hours, according to Garcés-Rimón et al. (2016)(Reference Garcés-Rimón, González and Uranga21). After enzymatic inactivation by increasing the pH to 7·0 with NaOH (5 N), the product was centrifuged at 2500 g for 15 min, and the supernatant was frozen and lyophilised until use. Furthermore, the following peptide sequences with antioxidant, antihypertensive and anti-inflammatory properties have already been previously identified (FRADHPFL, RADHPFL, YAEERYPIL, YRGGLEPINF, ESIINF, RDILNQ, IVF, YQIGL and SALAM)(Reference Miguel, Dávalos and Manso22). For using in treating animals, the hydrolysate was resuspended in drinking water and administered 1g/kg per day daily.
Animals and experimental models
Male Wistar rats (180–220 g) were obtained from the Central Animal Laboratory of the Federal University of Pelotas (Rio Grande do Sul, Brazil). Animals were maintained at standard conditions (constant room temperature, humidity, and 12:12 h light-dark) with water and fed ad libitum in the Federal University of Pampa vivarium. The experimental protocols were performed according to the guidelines of the National Council of Ethics with Animals (CONCEA), the National Institute of Health Guide for the Care and Use of Laboratory Animals (NIH, 1996) and the Local Institution Animal Care and Use Committee (protocol number 006/2022). All the procedures followed in this study were in accordance with the ethical standards of the Ethics Committee of the applicant’s research institution (approved by the Ethics Committee on Animal Use Experimentation of the Federal University of Pampa, Uruguaiana, Rio Grande do Sul, Brazil, protocol 006/2022) and in accordance with the guidelines for biomedical research stated by the Brazilian Societies of Experimental Biology.
Inclusion criteria
All animals exhibiting elevated blood pressure consistent with the levels specified by the DOCA-salt model were included in the study.
Exclusion criteria
The animals in the postoperative period and throughout the treatment were monitored by a veterinarian. Any animal that shows signs of suffering, such as assessment of motor activity, change in appearance, hunched posture, piloerection, ocular or nasal secretion, change in temperament, increased aggression, reluctance to change in vocalisation, chattering or grinding of teeth, increase or decrease in vocalisation, food or water consumption, abnormal weight loss, decreased excretion of urine and faeces, changes in skin colour, checking for erythema, oedema or other signs of infection at the surgical site, was removed from the study. If the animal had an infection in the surgical wound, it was treated with antibiotics as recommended by the veterinarian and would return to the study after recovery if considered recovered. Otherwise, if the infection is severe or other symptoms described above make its general condition or survival at risk or uncomfortable, the animal would be removed from the study, and the human outcome carried out according to the assessment. None of the above criteria were presented, and animals were removed from the study.
All animals (n = 32) underwent uninephrectomy before induction of HT, and after seven days of recovery, they were randomly divided into the SHAM group and DOCA-salt group. We used a randomisation table as a method. Each rat received a unique number upon allocation to different groups by the supervisor. N of eight animals per treatment group was used and divided into SHAM group, which received weekly subcutaneous injections of vehicle (mineral oil + propylene glycol 1:1) and drinking water; DOCA-salt group, which received weekly injections of DOCA (Sigma-Aldrich, Darmstadt, Germany) diluted in mineral oil + propylene glycol 1:1 subcutaneously in subsequent doses of 20 mg/kg in the 1st week, 12 mg/kg in the 2nd and 3rd week, and 6 mg/kg from the 4th to the 8th week of treatment, plus water supplemented with 1 % NaCl and 0·2 % KCl, according to Wenceslau & Rossoni et al. (2014)(Reference Wenceslau and Rossoni23). In the last four weeks of treatment, the SHAM animals were subdivided into the SHAM + EWH group, which was treated with EWH 1 g/kg/day via gavage, according to Rizzetti et al. (2017)(Reference Rizzetti, Martín and Corrales24), and the SHAM group treated only with drinking water via gavage. The DOCA animals were also subdivided into DOCA and DOCA + EWH groups (co-treated with EWH at 1 g/kg per day via gavage) (online Supplemental Fig. 1). The amount of food (in g) and liquid (in ml) ingested per animal was measured daily. To minimise potential confounders, we implemented a double-check process involving two experienced scientists in this field for each treatment step.
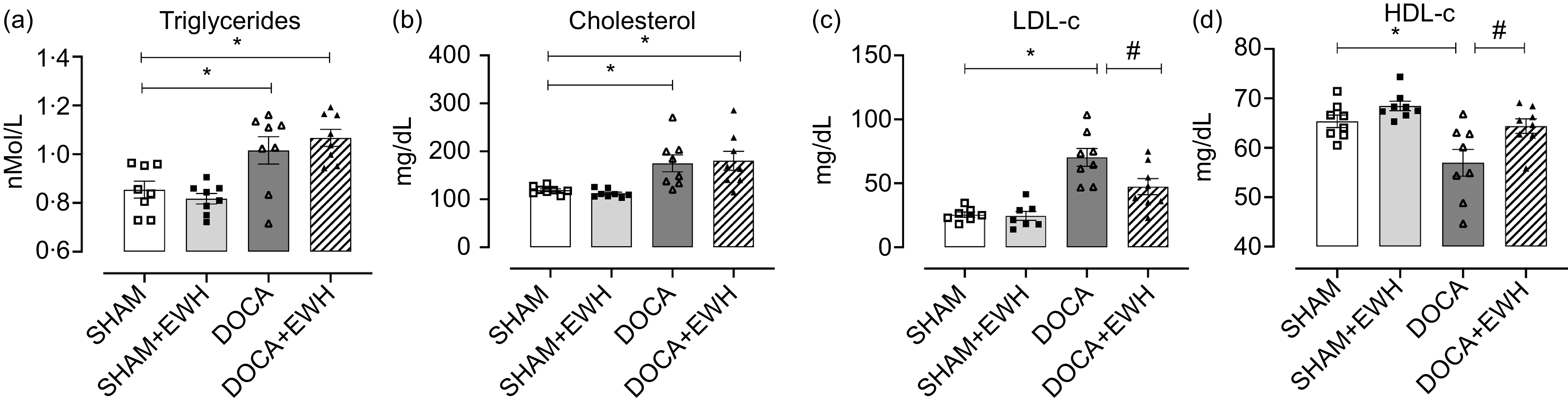
Fig. 1. Assessment of plasma lipid metabolism. Triglycerides (a), cholesterol (b), LDL-c (c), HDL-c (d). Data expressed as mean ± SEM. (n = 8). Two-way ANOVA followed by Bonferroni post-test P < 0·05 *vs SHAM; #vs DOCA.
Systolic blood pressure (SBP) was measured according to Buñag (1973)(Reference Buñag25) using a non-invasive method. The detection of the caudal arterial pulsation was performed with a tail cuff (pneumatic transducer, AD Instruments Pty Ltd., Bella Vista, NSW, Australia) in conscious and mechanically restrained animals, previously heated at 37 ºC for 10 min, for caudal artery dilation. All animals were submitted to a period of 1 week of acclimatisation before the measurements for the reliability of the pressure data. Measurements were performed weekly at the same time of the day. The results of SBP were expressed as mmHg.
At the end of the treatment, the animals were fasted for 12 hours. Before euthanasia, a blood sample from the caudal vein was collected to assess capillary blood glucose using blood glucose strips (On Call Plus II glucometer, ACON Laboratory, San Diego, CA, EUA). After euthanasia, blood was collected for glycosylated Hb determination (LabTest, Lagoa Santa, Brazil), and plasma was obtained for glucose (Bioclin, Porto Alegre, Brazil), glycosylated Hb (LabTest) and insulin determinations (Sigma-Aldrich) using commercial kits following the manufacturer’s guidelines. rtWAT was also excised and stored at –80 ºC for further biochemical determinations. Plasma lipids were evaluated using commercial colorimetric kits for measuring triglycerides (Bioclin), total cholesterol (Bioclin), HDL-c and LDL-c (LabTest). Analyses were performed according to the manufacturer’s instructions.
Biochemical analysis in retroperitoneal adipose tissue and plasma
rtWAT samples were homogenised in Tris-HCl (50 mM, pH 7·4) and centrifuged at 2400 g for 10 min at 4 ºC, and the supernatant was collected and used for further analysis. Reactive oxygen species, lipid peroxidation, total antioxidant capacity, the activity of the enzymes superoxide dismutase (SOD), catalase, glutathione peroxidase (GPx) and glutathione reductase (GR) were evaluated in rtWAT. Whole blood was centrifuged at 2500 rpm for 10 min at 4 ºC, and plasma was carefully obtained and stored at – 20 ºC. Plasma samples were diluted 1:10 with Tris-HCl (50 mM, pH 7·4) to analyse IL-6, IL-1β levels, GPx and GR activity.
Levels of reactive oxygen species were determined by 2’, 7’-dichlorofluorescein diacetate using the spectrofluorimetric method, according to Loetchutinat et al. (2005)(Reference Loetchutinat, Kothan and Dechsupa26). Data were expressed in fluorescence units.
Lipid peroxidation was measured through malondialdehyde levels using a colorimetric method according to Ohkawa et al. (1979)(Reference Ohkawa, Ohishi and Yagi27), and the thiobarbituric acid reactive substance assay data were expressed in absolute nanomoles of malondialdehyde.
Total antioxidant capacity was measured using ferric reducing antioxidant power, according to Benzie and Strain (1996)(Reference Benzie and Strain28). A standard dose-response curve of Trolox (50–1000 μM water-soluble vitamin E analogue) was prepared, and the ferric reducing antioxidant power assay was compared with Trolox equivalents expressed in mM of Trolox equivalents.
The activity of the enzyme SOD was analysed by spectrophotometry, as described by Misra and Fridovich (1972)(Reference Misra and Fridovich29). The ability of this enzyme to inhibit the autoxidation of adrenaline to adrenochrome was measured by the colour reaction at 480 nm and expressed in Units (U) of SOD activity.
The catalase enzyme activity was analysed by spectrophotometry, as described by Aebi (1984)(Reference Aebi30). Enzyme activity was expressed in catalase units (U).
GR levels were measured according to Armstrong et al. (1998)(Reference Browne and Armstrong31). Sample fluorescence was expressed in nMol GSH.
GPx activity was performed by spectrophotometry, according to Wendel (1981)(Reference Wendel32), and enzymatic activity was expressed in nMol GSH min–1.
IL-1β (Sigma-Aldrich) and IL-6 (Sigma-Aldrich) were measured using commercial kits in rtWAT samples following the manufacturer’s instructions.
Histological analysis
rtWAT was sectioned and fixed in 10 % formaldehyde for 24 h, then embedded in paraffin. Sections of 5 μm were made using a microtome and stained with haematoxylin and eosin. The images were obtained with a Zeiss Axioskop 2 microscope equipped with the image analysis software package LAS X (Leica, Munich, Germany). The analysis was performed on four random fields per section of adipose tissue under a 10X objective. Three slides per animal were quantified, with at least 4 regions per slide. The Adiposoft plug-in run in ImageJ Fiji version 2.1.0/1.53c was used to quantify the adipocyte size.
Immunohistochemistry and immunofluorescence
Immunohistochemistry was performed on rtWAT using antibodies anti-CD163 (1:750, Bio-Rad Laboratories, MCA342GA, Watford, UK), anti-TNFα (anti-TNFα, 1:150, ABclonal A11534, Barcelona, Spain) and anti-uncoupling protein-1 (UCP) (1:400, ABclonal A5857). Analyses were performed using 5 µm sections of tissue fixed in 10 % formaldehyde and embedded in paraffin. Antigenic retrieval was performed with 10 mM citrate buffer for 30 min at 95 ºC. The sections were incubated for 10 min in 3 % (vol/vol) hydrogen peroxide to inhibit endogenous peroxidase activity and blocked with horse serum for 20 min. After blocking, they were permeabilised with PBS with 0·05 % Tween 20 (Calbiochem, Darmstadt, Germany) for 30 minutes at room temperature. Primary antibodies were diluted in PBS containing 1 % BSA and incubated at 4°C overnight. The ImmPRESS® HRP Universal Peroxidase Kit (Vector Laboratories Inc., Burlingame, CA, USA; Horse Anti-Rabbit IgG Plus Polymer Kit or Horse Anti-Mouse IgG IgG Polymer Kit PLUS) was used as a secondary polymer. Horseradish peroxidase 3, 3′ diaminobenzidine tetrahydrochloride colorimetric substrate (Vector Laboratories Inc., Burlingame, CA, USA) was used for visualisation. Sections were counterstained with haematoxylin, dehydrated and mounted. The slides were studied under a Zeiss Axioskop 2 microscope equipped with the image analysis software package LAS X (Leica). Quantification of staining of the number of reactive cells was performed using the ImageJ Fiji software 2.1.0/1.53c.
For immunofluorescent analyses, rtWAT was prepared and analysed according to Jimenez-Altayó et al. (2005) with modifications for the adipose tissue(Reference Jiménez-Altayó, Briones and Giraldo33). The primary antibodies used were NOX-1 (1:400; Cat. 2 108 601, Sigma-Aldrich), NFκB (1:400; Cat. 4 502 615, Sigma-Aldrich) and caspase-3 (1:400; Cat. SAB5700914, Sigma-Aldrich). Alexa 488-conjugated goat anti-mouse immunoglobulin G (IgG) was diluted 1:500 (Cat. No. A11001, Invitrogen Life Technologies). DAPI (1:1000; Cat. MBD0015, Sigma-Aldrich) was used to stain the nuclei. In the preparation of negative control sections, we omitted the primary antibody. Images were acquired using an EVOS ® Floid ® Cell Imaging Station (Life Technologies, Carlsbad, CA, USA). For quantification, sections with the same capture parameters were analysed. Mean fluorescence densities (histogram) using ImageJ were calculated. Data were expressed as fluorescence intensity.
Statistical analysis
Data are expressed as mean ± SEM. We applied the ROUT method to identify and remove outliers, followed by a Shapiro-Wilk test to check the normality of the population data. We used the statistical program GPOWER to determine the number of animals used. We considered error type 1, 5 % (P = 0·05); CI = 95 %, power = 80 %; direction of effect, two tailed; statistical tests: ANOVA; expected attrition or death of animals = 20 %. Results were analysed using a two-way ANOVA followed by Bonferroni post-test (GraphPad Prism 8.0.1 software, San Diego, CA, USA). Differences were considered statistically significant with P values < 0·05.
Results
Table 1 depicts the effect of EWH on SBP, body weight gain, feed and water intake, and glycaemic parameters. DOCA-salt hypertension, as expected, elevated the SBP compared with SHAM group, and EWH supplementation significantly reduced these SBP levels compared with DOCA-salt animals. DOCA and DOCA + EWH animals showed a lower increase in weekly weight gain, accompanied by a decrease in caloric intake and an increase in water intake compared with SHAM group, and no differences were observed in both DOCA groups in these parameters.
Table 1. Body weight gain, systolic blood pressure, feed and water intake, and assessment of glucose metabolism parameters
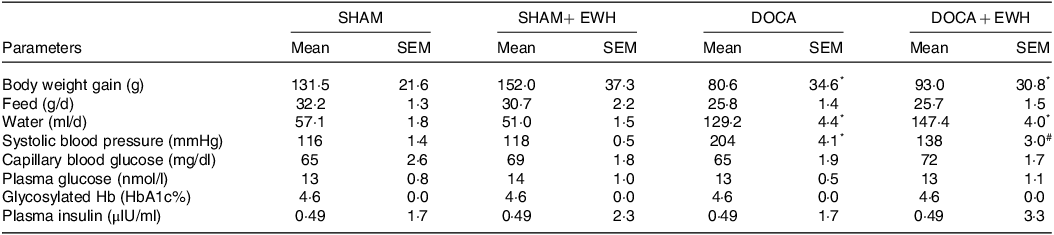
Data expressed as mean ± SEM. Two-way ANOVA followed by Bonferroni post-test.
P < 0·05 *vs SHAM group and # vs DOCA group.
EWH: egg white hydrolysate; DOCA: deoxycorticosterone acetate
Regarding metabolic parameters, all glycaemic metabolism parameters (plasma glucose, plasma insulin, blood glycosylated Hb and capillary blood glucose) were similar in the SHAM and DOCA groups with or without EWH treatment (Table 1). Lipid metabolism is shown in Fig. 1. The DOCA-salt hypertensive rats exhibited an increase in plasma triglycerides and total cholesterol compared with the SHAM group. After EWH supplementation in hypertensive animals, these parameters remained elevated, and no differences were observed when compared with the values of the DOCA group (Fig. 1(a) and (b)). However, the increase in LDL-c levels observed in the DOCA-salt HT group was effectively reduced after EWH supplementation. Moreover, the significant reduction observed in HDL-c levels in the DOCA group was recovered after EWH treatment in hypertensive animals, and this recovery reached the HDL-c SHAM levels (Fig. 1(c) and (d)).
Considering the importance of the fundamental role of balancing the redox system in treating and reducing the effects of HT on tissues, Fig. 2 shows a significant increase in lipid peroxidation levels in rtWAT in DOCA hypertensive rats that were not altered by EWH treatment (Fig. 2(a)). However, changes were not observed at ROS levels between the DOCA and SHAM groups (Fig. 2(b)). Total antioxidant status was increased in DOCA-salt compared with the SHAM group, and it was also maintained at elevated levels in the DOCA + EWH group (Fig. 2(c)). SOD activity was reduced by DOCA-salt hypertension compared with SHAM group, and EWH supplementation restored this critical antioxidant defence enzyme to control levels (Fig. 2(d)). In the same way, catalase activity was significantly increased in the DOCA-salt group, and EWH reduced this increase to control levels (Fig. 2(e)). We also evaluate the GPx and GR activity in rtWAT (Fig. 2(f) and (g)) and plasma (Fig. 2(h) and (i)). The GPx activity was increased in DOCA-salt rats in both rtWAT and plasma compared with the SHAM group, but EWH supplementation only maintained elevated GPx levels in rtWAT, and these high levels were reduced significantly in plasma compared with the DOCA group (Fig. 2(f) and (h)). The GR activity in rtWAT was similar in all groups (Fig. 2(g)). However, DOCA showed a significant increase in GR activity in plasma, and EWH supplementation restored GR activity in DOCA animals to SHAM levels (Fig. 2(i)).
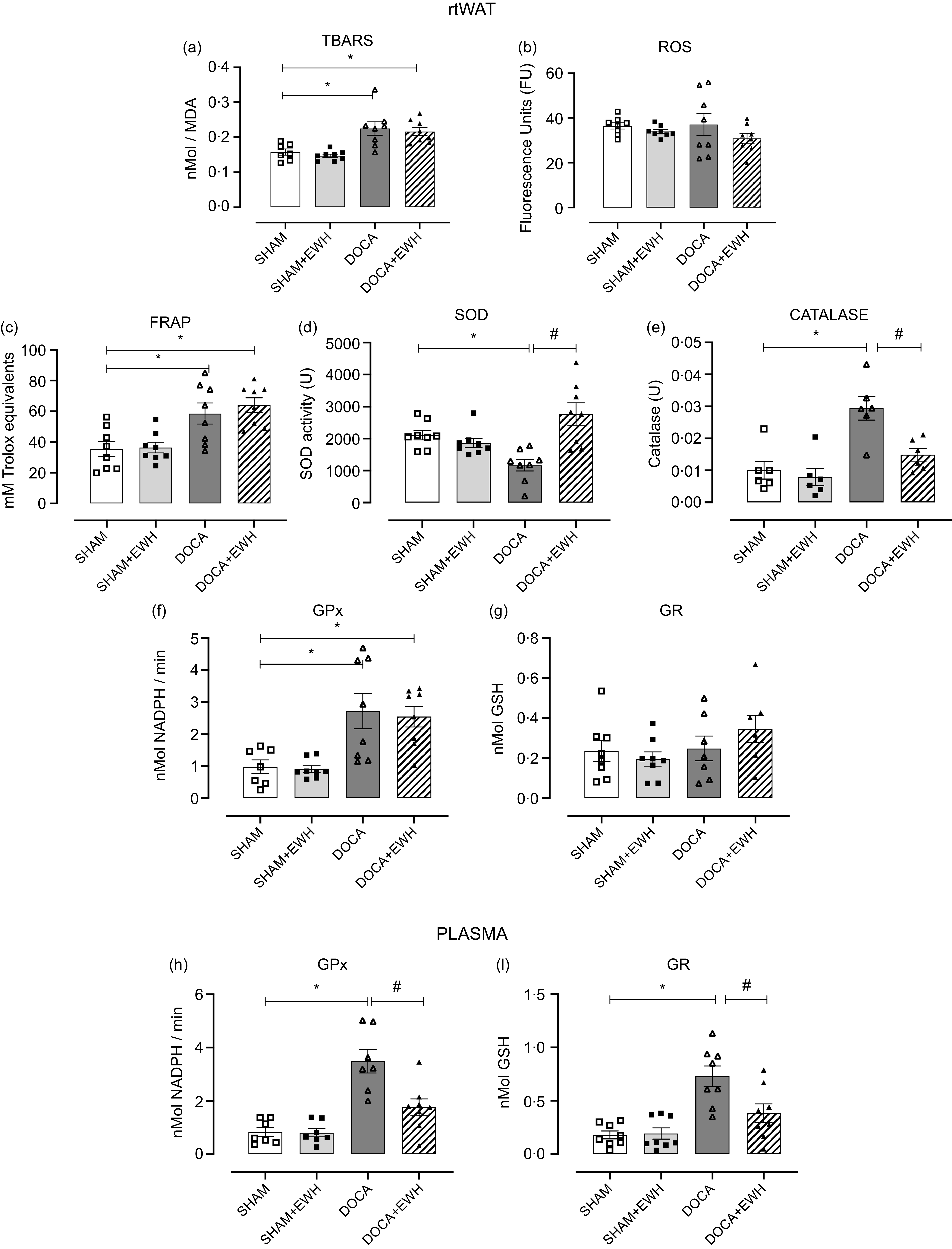
Fig. 2. Analysis of redox equilibrium in rtWAT and plasma in SHAM, SHAM + EWH, DOCA and DOCA + EWH groups. Lipid peroxidation levels – TBARS (a), reactive oxygen species – ROS (b), total antioxidant capacity – FRAP (c), the antioxidant enzymes superoxide dismutase (SOD) and catalase (D–E) were measured in rtWAT. GPx and GR neutralising enzymes were analysed in rtWAT (f–g) and in plasma (h–i). Data expressed as mean ± SEM. (n = 8). Two-way ANOVA followed by Bonferroni post-test P < 0·05 *vs SHAM; #vs DOCA. EWH: egg white hydrolysate; DOCA: deoxycorticosterone acetate
To corroborate the findings in the assessment of oxidative stress in biochemical analyses, we performed immunofluorescence with labelling for the subunit of NADPH oxidase, NOX-1, in rtWAT (Fig. 3(a–d) and online Supplemental Fig. 3). DOCA hypertensive animals showed a significant increase in fluorescence intensity of NOX-1 (Fig. 3(c)), and EWH administration reduced this important source of reactive species in HT animals to control levels (Fig. 3(d)).

Fig. 3. Effects of EWH co-treatment on NOX-1 expression in rtWAT of DOCA-salt hypertensive rats. Immunofluorescence representative images (the images correspond to the merge of the marking of the colours by the DAPI in blue and NOX-1 in green) and histogram of the groups SHAM, SHAM + EWH, DOCA and DOCA + EWH groups. Data expressed as mean ± SEM. (n = 8). Two-way ANOVA followed by Bonferroni post-test P < 0·05 *vs SHAM; # vs DOCA. EWH: egg white hydrolysate; DOCA: deoxycorticosterone acetate
After assessing oxidative stress and considering that the mechanism of chronic injury in HT promotes an active inflammatory process, the potential anti-inflammatory action of EWH by measuring proinflammatory IL-6 and IL-1β in rtWAT was analysed. Moreover, regarding adipocyte functional changes, which have been related to specific endocrine and cardiometabolic profiles, the morphological and metabolic characteristics of adipose tissue were evaluated. Compared with the SHAM group, IL-6 and 1β levels increased significantly in the DOCA group, and EWH administration showed a significant decrease of both cytokines in rtWAT (Fig. 4(a) and (b)). Alterations in the morphometry of adipocytes were not observed, and no differences in the size of adipocytes were found between groups (adipocyte area (µm2): SHAM: 1·4 ± 40·7; SHAM + EWH: 1·6 ± 241·4; DOCA: 1·7 ± 124·2 and DOCA + EWH: 1·6 ± 189·2; online Supplemental Fig. 2). However, when the infiltration of CD163+ macrophages and nuclear transcription factor (NFκB) were evaluated in rtWAT, significant alterations in the function of this tissue were observed (Fig. 5(a–d)). DOCA-salt HT promoted an increase in the number of macrophages in rtWAT, and administration of EWH was able to reverse this initial inflammatory response (Fig. 5(a–d)). Furthermore, hypertensive rats presented greater fluorescence intensity when NFκB was evaluated, and EWH treatment was able to reduce this parameter to SHAM levels (Fig. 5(a–d) and online Supplemental Fig. 4).
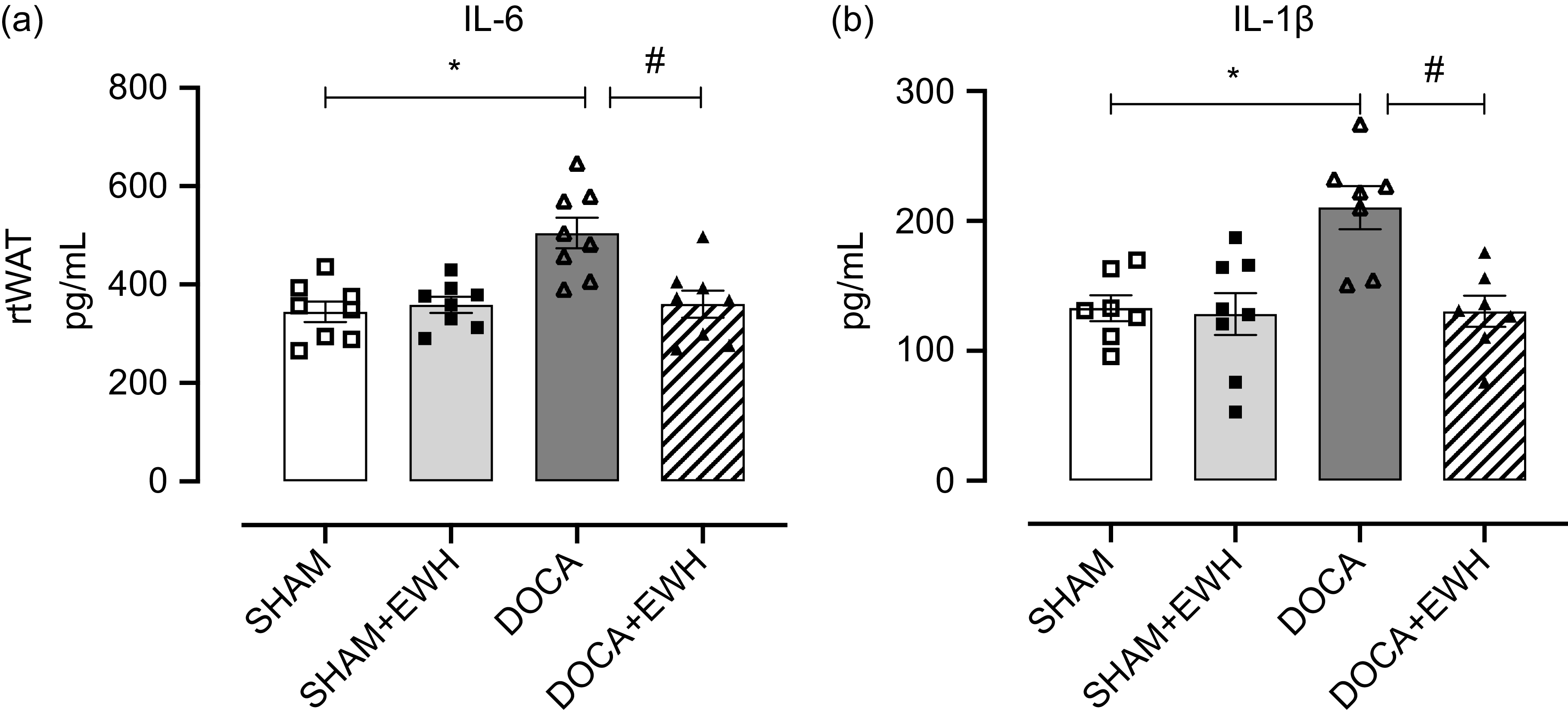
Fig. 4. Effects of EWH co-treatment on IL in rtWAT of DOCA-salt hypertensive rats. Bar graphs represent in (a) the IL-6 and in (b) IL-1B levels in rtWAT in all groups. Data expressed as mean ± SEM. (n = 8). Two-way ANOVA followed by Bonferroni post-test P < 0·05 *vs SHAM; #vs DOCA. EWH: egg white hydrolysate; DOCA: deoxycorticosterone acetate

Fig. 5. Effect of EWH co-treatment on CD163+ macrophage infiltration and NFκB expression in rtWAT of DOCA-salt hypertensive rats. Representative immunohistochemical photomicrograph images of CD163+ staining of SHAM (a), SHAM + EWH (b), DOCA (c) and DOCA + EWH (d) groups are indicated with arrows. Digital images were captured using the 10x and 20x objective, respectively. Scale bar, 50 μm. Also, representative immunofluorescence images of NFκB of groups SHAM (a), SHAM + EWH (b), DOCA (c) and DOCA + EWH (d) groups. Scale bar, 100 μm. Histogram graphs represent the number of macrophage and fluorescence of NFκB respectively in all groups. Data expressed as mean ± SEM. (n = 8). Two-way ANOVA followed by Bonferroni post-test P < 0·05 *vs SHAM; #vs DOCA. EWH: egg white hydrolysate; DOCA: deoxycorticosterone acetate
Considering the findings in oxidative and inflammatory parameters in rtWAT caused by DOCA-salt hypertension, the effect of EWH on apoptotic biomarkers was evaluated. DOCA group showed a significant increase in TNFα fluorescence intensity in rtWAT, and the supplementation with EWH reduced TNFα fluorescence intensity to SHAM levels (Fig. 6(a–d)). Likewise, for caspase-3, EWH treatment showed anti-apoptotic action in rtWAT by decreasing the fluorescence intensity of this effector caspase, which was significantly increased in this tissue in the DOCA group (Fig. 6(a–d) and online Supplemental Fig. 5).

Fig. 6. Effects of EWH co-treatment on TNFα and caspase-3 expression in rtWAT of DOCA-salt hypertensive rats. Representative immunohistochemical photomicrographs of TNFα of SHAM (a), SHAM + EWH (b), DOCA (c) and DOCA + EWH (d) in the groups are indicated with arrows. Also, representative immunofluorescence images of caspase-3 of groups SHAM (a), SHAM + EWH (b), DOCA (c) and DOCA + EWH (d) groups. Scale bar: 50 and 100 μm respectively. Histogram graphs represent the intensity of staining for TNFα and fluorescence of caspase-3 respectively in all groups. Data expressed as mean ± SEM. (n = 8). Two-way ANOVA followed by Bonferroni post-test P< 0·05 *vs SHAM; #vs DOCA. EWH: egg white hydrolysate; DOCA: deoxycorticosterone acetate
To analyse the functional alterations caused by increasing oxidative stress and the inflammatory process in DOCA-salt HT in rtWAT, the expression of the type 1 UCP-1 was also evaluated. EWH was able to reverse the increase in UCP-1 expression observed in the DOCA-salt HT group, showing an improvement in the catabolism of DOCA-salt hypertensive rats (Fig. 7(a–d)).
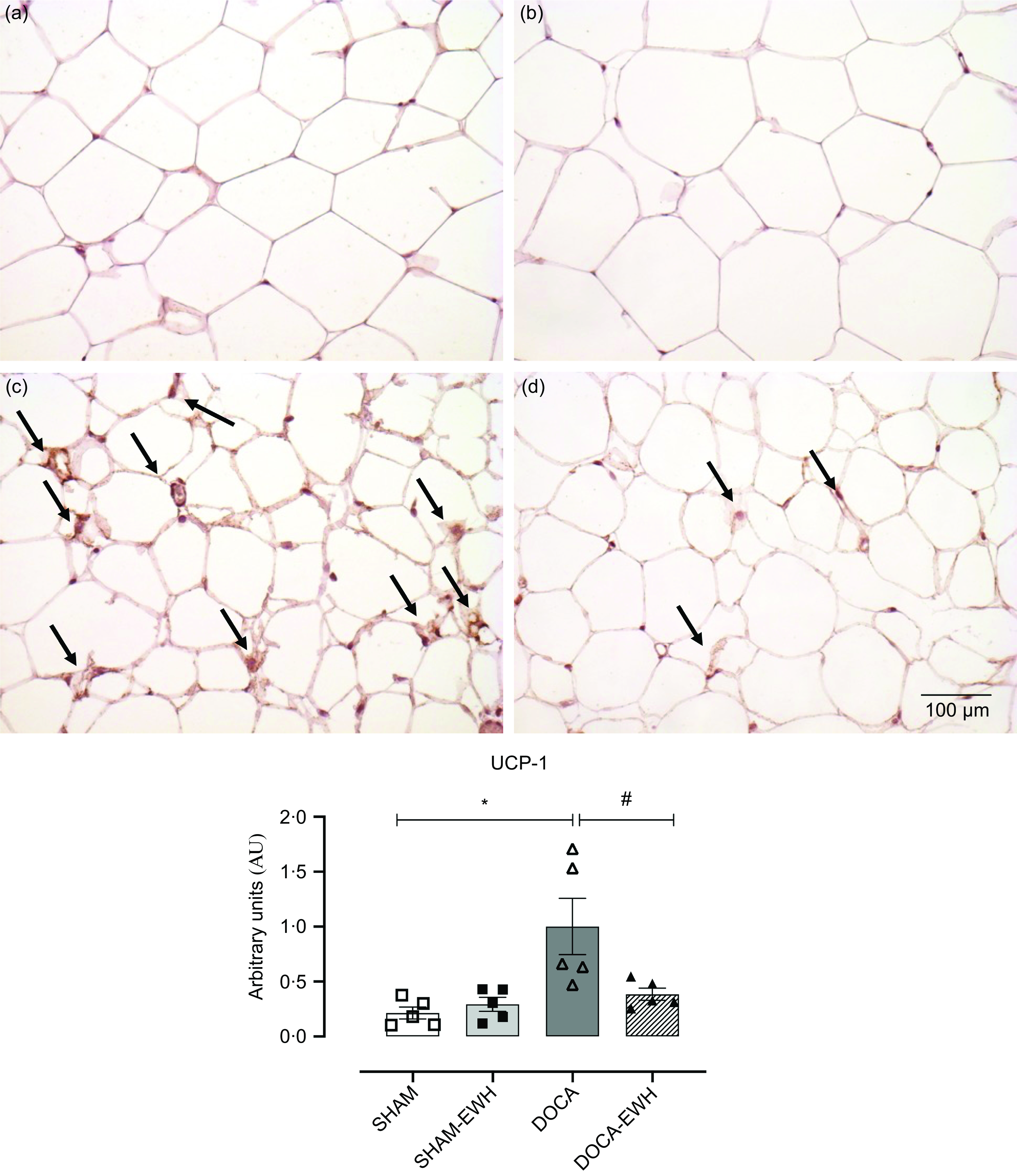
Fig. 7. Effect of EWH co-treatment on UCP-1 labelling in rtWAT of DOCA-salt hypertensive rats. Representative immunohistochemical images of UCP-1 labelling in SHAM (a), SHAM + EWH (b), DOCA (c) and DOCA + EWH (d) groups, scale bar 50 μm. Histogram graphs represent the intensity of staining for UCP-1 in all groups. Data expressed as mean ± SEM. (n = 8). Two-way ANOVA followed by Bonferroni post-test P < 0·05 *vs SHAM; #vs DOCA. EWH: egg white hydrolysate; DOCA: deoxycorticosterone acetate
Discussion
Our results show that EWH can be an effective therapeutic dietary strategy to regulate severe HT and its complications in the DOCA-salt experimental model by rtWAT functional changes. This effect may be partially mediated by decreased oxidative stress, as demonstrated by improved oxidative stress by reducing NOX-1 expression and reducing proinflammatory factors such as reduced macrophage infiltration and IL levels. Furthermore, these effects were accompanied by a decrease in pro-apoptotic factors such as caspase-3, which led to UCP-1 labelling in rtWAT, demonstrating the beneficial effects of EWH treatment in this tissue. It should be note that angiotensin-converting enzyme inhibition, antioxidant or anti-inflammatory mechanisms have been reported for EWH and their peptides. Moreover, it is known that specific amino acids and their position in the sequence are essential for bioactivity(Reference Miguel and Aleixandre34,Reference Garcia-Redondo, Roque and Miguel35) .
The antihypertensive effect produced after EWH administration in the DOCA-salt model was a 32 % reduction in SBP, corroborating a previous report using this hydrolysate(Reference Abreu, Rodrigues Moro and Hassan Husein Kanaan20). Other bioactive compounds derived from foods have also demonstrated antihypertensive effects in hypertensive experimental models, specifically in DOCA-salt hypertensive animals. Administration of flaxseed concentrates reduced diastolic and SBP in DOCA-salt rats, showing a similar pattern to the produced by captopril(Reference Sawant and Bodhankar36). Furthermore, an antihypertensive effect in DOCA-salt rats was also found using morin, a potent flavonoid, and this effect was associated with an improvement in lipid profile(Reference Prahalathan, Saravanakumar and Raja37). However, studies using alternative dietary strategies in models of secondary hypertension with an assessment of adipose tissue, especially rtWAT, have yet to be explored.
In a rat model with human primary aldosteronism, which also occurs with HT, the deleterious oxidative damage promoted by high blood pressure also affects adjacent tissues related to cardiovascular health, such as visceral adipose tissue, which is closely linked to regulating glycaemic and lipid metabolism(Reference Jadhav and Ndisang38). It has been described that WAT remodelling promotes beneficial effects on obesity and insulin resistance(Reference Kuryłowicz and Puzianowska-Kuźnicka39,Reference Fang, Suh and Reilly40) , and unlike obesity, HT does not confer an anabolic profile on WAT(Reference Das, Moon and Lee41,Reference Koenen, Hill and Cohen42) . When we observed adipocyte morphometry, there was no change in cell area or lipid droplet deposition, but adipose functionality was altered through oxidative and inflammatory factors. Our findings in the assessments of lipid metabolism demonstrate the possible catabolic action derived from DOCA-salt HT on cholesterol, showing a reduction in beneficial HDL-c and an increase in LDL-c, which are considered harmful and generate an increased risk of CHD. These findings have also been corroborated in several other studies using spontaneously hypertensive rat or DOCA-salt experimental models(Reference Prahalathan, Saravanakumar and Raja37).
These results suggest that consumption of EWH promotes hypocholesterolemic action with the restoration of circulating lipid mobilisation. Alternative strategies derived from a plant extract of Melothria maderaspatana leave also showed hypolipidemic activity in DOCA-salt rats(Reference Veeramani, Al-Numair and Chandramohan43). Similarly, a study using the same HT model revealed a reduction in HDL-c and an increase in LDL-c levels, and the flavonoid supplementation restored lipoprotein levels to an improved lipid profile(Reference Prahalathan, Saravanakumar and Raja37). Furthermore, the hypolipidemic effect induced by EWH has been previously observed in other experimental hypertensive(Reference Manso, Miguel and Even44) and obese animal models(Reference Requena, Miguel and Garcés-Rimón17,Reference Moreno-Fernández, Garcés-Rimón and González19) .
The hypocholesterolemic action provided by food protein hydrolysates has been related to the release of bioactive peptides from native proteins with anti-inflammatory and anti-atherogenic activity already(Reference Gomes, Lima and Alves45). Although it was not observed an improvement in cholesterol and triglyceride levels in DOCA-salt animals treated with EWH, the improvement in HDL and LDL levels associated with a reduction in inflammatory IL suggests a potential mechanism of action by EWH for protecting WAT. Similarly, in geriatric mice, the consumption of egg whites decreased lipid peroxidation and increased expression of anti-inflammatory IL-10(Reference Jiayu, Botta and Simtchouk46). Furthermore, an EWH obtained after thermolysin hydrolysis for 1·5 h demonstrated adipogenic differentiation capacity in pre-adipocytes, which interestingly can be attributed to the physiological effect of insulin, increasing the expression of the anti-inflammatory metabolic modulator PPARγ(Reference Jahandideh, Chakrabarti and Davidge47).
The redox imbalance observed in this model of severe HT promotes deleterious damage not only in the cardiovascular system but also in adipose tissue, especially in rtWAT, and our study demonstrates for the first time the protective action of a food protein hydrolysate in the injury caused by this condition in this tissue. The lipolytic action of WAT, described in this study, is influenced by increased oxidative action with an alteration of antioxidant enzymatic activity, favouring a catabolic state with increased lipid peroxidation at local and systemic levels of GPx. On the other hand, EWH attenuates catalase activity and restores SOD activity by reversing lipid peroxidation in rtWAT in co-treated rats. Studies with phenolic food compounds, especially epicatechin, demonstrate a reduction in the levels of malondialdehyde and aortic superoxide anion with suppression of NADPH oxidase activity and its p47phox and p22phox isoforms in DOCA-salt hypertensive rats(Reference Gómez-Guzmán, Jiménez and Sánchez48). Considering that angiotensin type 2 and/or mineralocorticoids stimulate the production of ROS by activation of the NADPH oxidase complex, we evaluated the labelling of the NOX-1 subunit by immunofluorescence technique in WAT. Our findings demonstrated that supplementation with EWH reduced the ROS levels derived from the overactivation of this enzyme complex in rtWAT tissue.
In addition to oxidative damage as the main precursor in the development of HT, with decreased NO bioavailability and increased ROS, some adipokines may also contribute to the pathogenesis of HT and cardiometabolic diseases(Reference Smekal and Vaclavik49–Reference Papathanasiou, Spyropoulos and Michael51). Our results also demonstrated an anti-inflammatory action of EWH by reducing levels of proinflammatory cytokines, IL-1β and IL-6 in rtWAT. Furthermore, in a study on adiponectin inhibition in hypertensive mice, DOCA-salt demonstrated impairment in vascular function by promoting hypertensive vascular injury and secretion of nuclear transcription factors(Reference Ruan, Ma and Ge52). The activation of prostaglandins and adipokines, mediated by the activation of TNFα and NFκB, triggers the defence system, inducing the production of phagocytic cells to reduce tissue damage caused by HT(Reference Zhang, McNerney and Riek53). EWH also reversed CD163+ macrophage infiltration and restored NFκB levels and TNFα expression in the co-treated group, promoting immune homeostatic recovery. These results suggest the meaningful participation of TNFα in the damage caused by HT and suggest that it leads to the activation of the NADPH oxidase complex, increasing ROS and generating oxidative damage. In fact, an animal model with genetic deficiency of TNFα in DOCA-salt hypertension demonstrated a significant reduction in the expression of inflammatory markers IL-1β, monocyte chemoattractant protein-1 and monocyte/macrophage marker F4/80, and a decreased expression of NADPH isoforms, gp91phox and p22phox, NADPH isoforms and prevented the reduction of eNOS in vascular tissue(Reference Cai, Hao and Liu54).
Additionally, considering that macrophages are phagocytic cells that lead to tissue homeostasis by removing apoptotic cells and releasing growth factors(Reference Zhang, McNerney and Riek53) when an apoptotic effector factor was evaluated, EWH administration effectively restored caspase-3 levels in rtWAT, protecting the tissue of apoptotic cell damage. Similarly, treatment with DOCA-salt had already demonstrated to be a mutagenic character producing DNA damage by induction of several proinflammatory genes and proteins, as demonstrated in a comet assay(Reference Schupp, Kolkhof and Queisser55). Moreover, in a recent study, the anti-apoptotic capacity of EWH was shown reducing significantly caspase-3 expression in the vascular tissue of animals exposed to high cadmium concentrations(Reference Piagette, Gomes and Husein56). Our data corroborate these previous findings; although seen in a different tissue, it still demonstrates that EWH can reverse the activation of apoptotic cascades, possibly mediated by an anti-inflammatory process.
In DOCA-salt hypertension, adipose tissue is highly active due to the catabolic process of fats, such in PVAT(Reference Son, Oh and Lee57) but also in rtWAT. As observed in this study, the increase in the UCP-1 marker suggests a modification known as ‘browning’, which indicates an increase in thermogenic activity in the WAT, also observed in PVAT. This process could be associated with increased local inflammation, with activation of IL-6, a cytokine whose production can be stimulated by NFκB and TNFα(Reference Son, Oh and Lee57). In animal models of heart failure, an increase in UCP-1 expression was observed in epididymal and inguinal. Its increase was also related to overexpression of p38-mitogen-activated protein kinases, an essential marker of oxidative stress and inflammation(Reference Valero-Muñoz, Li and Wilson58). In addition to observing an increase in UCP-1 expression in this peripheral fat (rtWAT), EWH surprisingly reversed this damage and prevented the development of this modification in this tissue.
The promoted browning effect observed of rtWAT in our study could also be related to the redox imbalance with the overactivation of NADPH oxidase and the alteration of the antioxidant enzymatic activity. This is demonstrated here as an exacerbated mitochondrial oxidative activity observed through the increase of ROS and the UCP-1 marker. This alteration of the adipose tissue, with an increase in the number of mitochondria in the unilocular adipocyte, characterises the darkening of the tissue related to modulation by adipokines. Recent evidence shows that angiotensin II could be an essential adipokine released by adipose tissue, capable of regulating adipose function and plasticity(Reference Cai, Fang and Jiang59). Furthermore, Sheng et al. (2016)(Reference Sheng, Ruan and Ma60) demonstrated that the β3-adrenergic receptor modulates lipolysis in PVAT, and its regulatory mechanism is associated with PVAT darkening and increased UCP-1 expression in DOCA-salt hypertensive rats. Besides, in WAT, the index of sympathetic activation of adipose tissue, the UCP-1 receptor, is increased under the chronic elevation of angiotensin receptor type 1-dependent angiotensin type 2, favouring the activation of adipose catabolic function(Reference de Kloet, Krause and Scott61).
Our findings suggest that the negative energy balance observed in DOCA-salt HT can be attributed to increased activation of oxidative stress and the triggering of an inflammatory process in rtWAT. However, regarding the catabolic profile of DOCA-salt HT is also related to increased plasma sodium and central activation of the renin-angiotensin system RAS(Reference Sheng, Ruan and Ma60,Reference de Kloet, Krause and Scott61) this mechanism could be also implicated in the beneficial effect produced by EWH, because this hydrolysate has demonstrated angiotensin-converting enzyme inhibitory activity in previous studies(Reference Miguel, Manso and Martín-Álvarez62). Future research will be conducted to explore the implications of this pathway.
Conclusions
In this work, we have demonstrated that the beneficial effects produced by administration of EWH in DOCA-salt HT model could be related to the improvement of the adipose function of rtWAT, through antioxidant, anti-inflammatory and anti-apoptotic mechanisms, and showing an improvement in catabolic metabolism. EWH has demonstrated a promising action as adjuvant treatment for secondary arterial hypertension with the recovery of adipose function through the restoration of the mobilisation of circulating lipids, reduction of total antioxidant capacity, reduced levels of ROS and lipid peroxidation, recovery of SOD activity and catalase activity, and also restored the activity of systemic GPx and reductase. The inflammatory and immune responses, caspase-3 and UCP-1 were also restored in rtWAT. Therefore, EWH could be used as an alternative dietary therapeutic strategy in the control of secondary HT and complications related to functional modifications of rtWAT. The improvement of adipose tissue dysfunction by the EWH, attributed to its antioxidant and anti-inflammatory properties in hypertensive conditions, could prompt novel research explorations, including the potential investigation into its role in attenuating or inhibiting the deposition of atherosclerotic plaques.
Acknowledgements
We thank Biopampa/UNIPAMPA for their assistance in caring for the mice.
This work was supported by the National Council for Scientific and Technological Development – CNPq (Edital Universal/CNPq No 44181/2014-9 and PQ/CNPq 311834/2020-5); Coordenação de Aperfeiçoamento de Pessoal de Nível Superior – Brasil (CAPES); Fundação de Amparo à Pesquisa do Rio Grande do Sul – FAPERGS/Brazil (PQG:19/2551-0001810-0); Programa Nacional de Cooperação Acadêmica; Pró-Reitoria de Pesquisa – Universidade Federal do Pampa (N. 20180615102630); FAPES/CNPq/PRONEX (N. 80598773), Foundation for Research Support of the State of São Paulo (FAPESP 2019/08026-5), and Spanish Government by the Agencia Estatal de Investigación (AEI) and Fondo Europeo de Desarrollo Regional (FEDER) (AGL2017-89213); I-COOP + 2020 (COOPA 20 453). ELA was supported by CAPES/Brazil, CRM by FAPERGS/Brazil.
C.R.M.: Investigation, Conceptualisation, Methodology, Formal analysis, Visualisation, Writing – original draft, review & editing; E.d.L.A.: Investigation, Methodology, Formal analysis, Writing – review & editing. S.H.H.K.: Investigation, Formal analysis, Writing – review & editing. J.A.U-O: Conceptualisation, Methodology, Validation, Writing – original draft, review & editing. L.V.R.: Conceptualisation, Methodology, review & editing; D.V.V.: Resources, Funding acquisition, Writing – original draft, review & editing. M.M.-C.: Conceptualisation, Methodology, Validation, Resources, Project administration, Funding acquisition, Writing – original draft, review & editing. G.A.W.: Conceptualisation, Methodology, Formal analysis, Validation, Visualisation, Data curation, Supervision, Project administration, Resources, Funding acquisition, Writing – original draft, review & editing. All authors have read and agreed to the published version of the manuscript.
The authors declare that they have no known competing financial interests or personal relationships that could have appeared to influence the work reported in this paper.
Supplementary material
For supplementary material/s referred to in this article, please visit https://doi.org/10.1017/S0007114524000552.