Lignin is a complex aromatic structure composed of phenylpropanoids bonded to cell wall carbohydrates and proteins. In ruminant nutrition, lignin has been negatively correlated with the digestibility of fibre in feedstuffs and has been used as an indicator of the potential extent of neutral-detergent fibre (NDF) digestibility (NDFD)(1). NDF describes the most nutritionally recalcitrant portion of the plant cell wall that is predominantly composed of hemicellulose and cellulose that may be digested by ruminal microbes and lignin. The two most common empirical lignin analyses applied in nutrition are Klason lignin (KL) and acid-detergent lignin (ADL). The question as to which assay is most appropriate for assessing digestibility of forage NDF, and the nutritional qualities of forages in ruminant nutrition have not been resolved.
The KL analysis was developed by wood chemist Johan Peter Klason as a measure of total lignin for an application to wood and pulps used for the production of paper(Reference Klason2). An official method (Association of Official Analytical Chemists (AOAC) Official Method 994.13) includes KL as part of an analysis for dietary fibre(Reference Theander, Aman and Westerlund3). In that method, the sample is pre-extracted with methanol, and the starch is removed through enzymatic hydrolysis and extraction. The residue is incubated in 12 m (72 %) H2SO4 at 30°C for 1 h to hydrolyse and solubilise carbohydrate. This is followed by dilution with water to 3 % acid and 1 h of autoclaving at 125°C before filtration, washing, drying and weighing, ashing and reweighing of the residue. The net loss on ashing is KL. The autoclaving step causes considerable precipitation of matter soluble in the initial treatment with H2SO4. Matter dissolved in 12 m H2SO4 and precipitated on dilution was termed as ‘acid-soluble lignin’(Reference Sullivan4). KL has been applied to forages and other animal feeds. It has been collaboratively studied on human foods(Reference Theander, Aman and Westerlund3), but no similar work has been done for forages.
ADL and NDF were developed as nutritional entities, with ADL used to estimate indigestibility of NDF. This system was designed to be applied to animal feedstuffs, particularly forages(Reference Van Soest5,Reference Van Soest6) . In the ADL method, the sample is boiled 1 h in 0·5 m H2SO4 and 2 % of cetyl trimethylammonium bromide. The filtered, washed and dried product (acid-detergent fibre (ADF)) is treated with 12 m H2SO4 for 3 h at 20°C to hydrolyse and solubilise carbohydrate. The hydrolysis is performed in fritted crucibles with repeated additions of acid that allows acid and hydrolysates to filter away during the reaction. The residue is filtered, washed and dried at 100°C and then weighed, ashed and reweighed. The net loss on ashing is ADL. Detergent extraction reduces protein content of the residue to low amounts; initial work on the ADL method exposed the problem of the inflation of lignin values related to heat damage of proteins upon drying samples at temperatures ≥50°C during the sample preparation(Reference Van Soest7). Boiling with acid detergent removed some soluble lignin-like matter(Reference Lowry, Kennedy and Conlan8). The ADL procedure allows materials dissolved in 12 m H2SO4 to be filtered away before washing the sample with water, and thus reducing the likelihood that materials soluble in the acid precipitate and become included in the ADL residue. The ADL procedure has been widely applied to forages and is used in the prediction of energy values of feeds(1) and in the Cornell Net Carbohydrate and Protein System model for the evaluation of ruminant diets(Reference Tedeschi and Fox9).
ADL gives substantially lower lignin values than KL(Reference Lowry, Kennedy and Conlan8,Reference Hatfield, Jung and Ralph10–Reference Lowry, Conlan and Schlink13) . Both empirical methods describe lignin, but for different purposes, with their differing values and compositions indicating that KL and ADL are not compositionally identical. Residues from both KL and ADL were reported as being largely free of major carbohydrate contamination and had similar patterns of pyrolytic products(Reference Hatfield, Jung and Ralph10). Upon analysis, identifiable phenolics are not detected in ADL(Reference McSweeney, Dulieu and Katayama11), although such phenolics are found in KL(Reference Raffrenato14). Substances precipitated on dilution and autoclaving that are included in KL are likely not included in ADL. One possible reason that KL values are greater than those of ADL is their inclusion of lignin and phenolic compounds not polymerised to the ADF-associated lignin(Reference Lowry, Kennedy and Conlan8); these may include the soluble lignin detected in rumen contents(Reference Gaillard and Richards15). Increasing temperatures of the 72 % H2SO4 hydrolysis, which are higher in the analysis of KL than of ADL, have been associated with increases in lignin values(Reference Ritter, Seborg and Mitchell16).
Artefactual lignin derived from protein in the plant can contribute to lignin through at least two mechanisms: the condensation of phenylpropanoid tyrosine residues(Reference McDougall, Stewart and Morrison17) and/or the formation of Maillard products during drying or heating of the sample prior to analysis(Reference Van Soest7). The Maillard reaction is pH sensitive with a maximum at slightly acidic to neutral pH but will depend on the reactants present(Reference Kato18) and is greatly reduced in strong acid. The presence of added protein variably inflates KL values, with the greatest amounts of protein causing less inflation than lower amounts; the KL values could not be adjusted to correct for the interference(Reference Norman and Jenkins19).
The lower N content of ADF has also been suggested as a partial basis for the lower lignin values of ADL, with reaction products of protein with other plant constituents being included in KL(Reference Norman and Jenkins19). Per kg of plant DM, KL contained 3·6– 6·5 times as much N as did ADL in grass stems (mean N in lignin: KL = 1·45 g N/kg plant DM and ADL = 0·28 g N/kg plant DM), and KL in lucerne stems contained 1·8–2·0 times as much N (mean N in lignin: KL = 2·39 g N/kg plant DM and ADL = 1·28 g N/kg plant DM) calculated from Hatfield et al. (Reference Hatfield, Jung and Ralph10). The presence of some N in lignin may be a consequence of linkages between lignin and cell wall proteins in the plant rather than in an analytical artifact(Reference McDougall, Stewart and Morrison17).
The difference between KL and ADL (KL – ADL = ΔL) has been ignored as a biological variable and dismissed as nothing more than error in KL or ADL. Evaluation of the relationships between the lignin methods, the difference between them and NDFD could clarify the utility of the methods for the purpose of defining nutritional characteristics of feeds. For use in ruminant nutrition, both in research and for diet formulation on farms, anaerobic in vitro fermentation of forages with mixed ruminal microbes is the most common bioassay used to assess NDFD. The use of this in vitro method allows substantial reduction in the number of animals required to determine NDFD and allows multiple forages to be compared simultaneously. The objective of this paper was to investigate ΔL and its relation to KL, ADL and in vitro NDFD estimated using mixed ruminal microbes.
Methods
Data sets
Two data sets containing information on KL and ADL (DM basis) and 48 h in vitro NDFD (as percentage of NDF; NDFD48) by mixed ruminal microbes were used in the present analysis. We found no other works published in journals that contained the needed individual sample KL, ADL and NDFD48 data. The data set from J.B. Robertson at Cornell University (Cornell data set) consists of seven C3 grass forages, seventeen C4 forages (thirteen maize plants and four maize silages) and nineteen alfalfa hays analysed for ADL and NDFD48(Reference Van Soest20) and KL(Reference Theander, Aman and Westerlund3). Ruminal inoculum for the in vitro fermentations performed by Robertson was obtained via a rumen cannula from a mature, non-lactating, ruminally cannulated Holstein cow fed a mixed, predominantly grass hay diet and provided water ad libitum. The cow was housed in a tie stall barn with other cattle, provided daily access to a pen for exercise and was maintained under protocols approved by the Cornell University Institutional Animal Care and Use Committee (protocol no. 1991-0016). The second data set (Jung data set(Reference Jung, Mertens and Payne21)) consists of fifteen C3 grasses, eight C4 forages and six alfalfa samples; values for ADL and KL are from their Table 1, and NDFD48 were determined from their figures. Ruminal inoculum used in generation of the Jung data set was obtained ‘after 12 h without feed’ from a non-lactating Holstein cow provided with alfalfa–grass hay. Seven samples were omitted from the original Jung data set: six non-alfalfa legume species were omitted because there were no equivalent samples in the Cornell data set and one C3 grass because its NDFD48 value could not be read from their figures. Within each study, ΔL, the arithmetic difference between KL and ADL as a percentage of sample DM, was calculated, and also ΔL as a percentage of KL (ΔL/KL). Arithmetic mean values with standard deviations for each forage type within data set are presented in Table 1.
Table 1. Arithmetic mean values and standard deviations for lignin, ΔL (Klason lignin (KL) – acid-detergent lignin (ADL)) and fibre digestibility in the data sets

NDFD48, in vitro 48 h neutral-detergent fibre digestibility.
Statistical methods
Data were evaluated within forage type for the effects on dependent variables of data source (Cornell, Jung), analytes and their interaction using mixed model analysis (PROC MIXED procedure, SAS version 9.4, SAS Institute Inc.) using no random variable. The respective combinations of independent with dependent variables evaluated were ADL with ΔL, KL and ΔL/KL and NDFD48 with ADL, KL, ΔL and ΔL/KL. A single forage sample was the unit of analysis. Regression analysis was performed by forage type (C3, C4 and alfalfa) within data set to provide linear regression curves using the PROC REG procedure of SAS. Correlations among variables within data sets were determined using PROC CORR of SAS. Significance was declared at P < 0·05 and tendency at 0·05 ≤ P < 0·15. Tendency is allowed to 0·15 given the relatively few values for each forage type in the data sets.
Results
The effect of data source and its interaction with independent variables was either non-significant (P > 0·15) or showed only tendencies (0·06 < P < 0·135; Table 2). The latter occurred predominantly with analyses that had NDFD48 as the dependent variable. The evaluation for C3 forages with ΔL/KL as the dependent variable and ADL as an independent variable was the sole evaluation including only chemical composition variables that had a tendency (P = 0·088) for a data source by independent variable interaction. In no evaluation with any forage type was ADL significant in the prediction of ΔL (P > 0·33) nor was ΔL significant for prediction of NDFD48 (P > 0·156; Table 2).
Table 2. P values and standard errors of the difference (SED) for evaluation of the effects of data source with different dependent and independent variables*
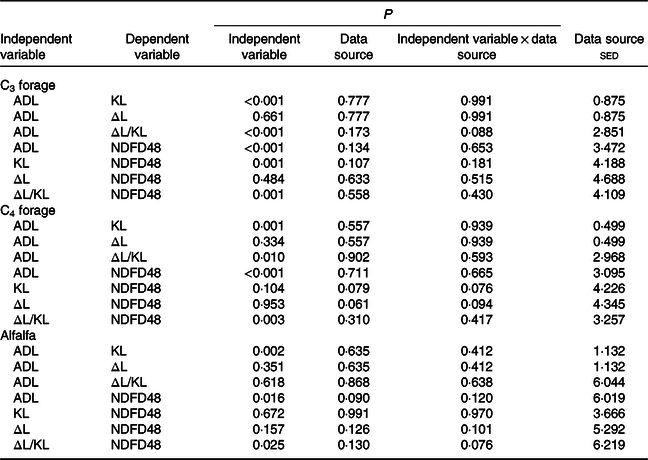
KL, Klason lignin, % of DM; ADL, acid-detergent lignin, % of DM; ΔL, KL – ADL, % of DM; NDF, neutral-detergent fibre, % of DM; NDFD48, in vitro 48 h neutral-detergent fibre digestibility, % of neutral-detergent fibre.
* Data source: Cornell or Jung(Reference Jung, Mertens and Payne21).
In the data sets, correlations of ΔL as a percentage of sample DM with NDFD48 are insignificant (P > 0·17) and vary from negative to positive (Table 3). This is in contrast to negative correlations of NDFD48 with ADL (significant or tendency for all but alfalfa in the Jung data set) and of NDFD48 with KL (Table 4). For the latter, the relationship was largely negative, more variable and non-significant for alfalfa (both data sets) and C4 forages in the Jung data set.
Table 3. Correlations of ΔL (Klason lignin (KL) – acid-detergent lignin (ADL); % of DM) on a DM basis with 48 h neutral-detergent fibre digestibility (NDFD48, % of neutral-detergent fibre), KL and ADL

Table 4. Correlations of Klason lignin (KL, % of DM), acid-detergent lignin (ADL, % of DM) and 48 h neutral-detergent fibre digestibility (NDFD48, % of neutral-detergent fibre)

Among the analytes, correlations of ΔL with ADL are low (r < 0·27) and insignificant (P > 0·30) with the exception of alfalfa (r 0·607, P < 0·01) in the Cornell data set (Table 3). Correlations of ΔL with KL are variable, positive and significant (P < 0·05) or tending to be significant (P = 0·098) for all forage types except for C3 grasses in the Cornell data set (Table 3). In contrast, KL and ADL were highly correlated with each other (r 0·605–0·896) in both data sets, with correlations significant (P < 0·01) for all but C4 and alfalfa forages (P = 0·11) in the Jung data set (Table 4). The proportion of ΔL in KL is also large and variable, averaging approximately 50 % of KL with a range of 22·1–83·3 %, and being somewhat higher in grasses than in alfalfa (Table 1). In the six C3 and one C4 samples with ΔL/KL of >70 %, ADL ranged from 1·7 to 3·4 % of DM.
The relationships of ADL, KL and ΔL are shown in Figs. 1 and 2. For all forages, KL increases with increasing ADL, with the regressions significant (P < 0·01) or tending towards significance (P = 0·11) (Fig. 1). The ΔL relationship with ADL is relatively flat (slopes from 0·047 to 0·347) and statistically insignificant (P > 0·30), with the exception of the relationship in the Cornell data set alfalfas in which ΔL rose with a slope of 0·713 relative to ADL (P < 0·01; Fig. 1(c)). The relationships of ADL with ΔL expressed as a proportion of KL (ΔL/KL) for C3 and C4 grasses show a steep decline (slopes from −3·62 to −6·50) in ΔL/KL with increasing ADL (P < 0·02; Fig. 2(a) and (b)). In the Jung data set, C4 grasses had a numerically similar negative slope (−4·40), but the relationship was NS (P = 0·32) and had a root mean squared error one-third to more than twice as large as noted for the other grasses. Legumes show no significant association of ΔL/KL with ADL (P > 0·55; Fig. 2(c)). There is a positive relationship of increasing ΔL/KL with NDFD48 for C3 and C4 forages (Fig. 3(a) and (b)), though this was non-significant (P > 0·15) for the alfalfas (Fig. 3(c)).

Fig. 1. Relationships of Klason lignin (KL) and ΔL (KL – ADL) with acid-detergent lignin (ADL) as percentages of DM for forages. (a) C3 forages; (b) C4 forages; (c) alfalfa. RMSE , root mean squared error; r, correlation. Cornell data set: , CKL;
, CΔL, closed symbols; Jung data set:
, JKL;
, JΔL, open symbols.

Fig. 2. Relationship of ΔL (KL – ADL) as a percentage of Klason lignin (KL) with acid-detergent lignin (ADL) as a percentage of DM. (a) C3 forages; (b) C4 forages; (c) alfalfa. r, Correlation. Regression lines: , Cornell data set;
, Jung data set.
, Cornell;
, Jung.

Fig. 3. Relationship between the 48-h in vitro digestibility of neutral-detergent fibre (NDFD48) with ΔL as a percentage of Klason lignin (ΔL/KL). (a) C3 forages; (b) C4 forages; (c) alfalfa. r, Correlation. Regression lines: , Cornell data set;
, Jung data set.
, Cornell;
, Jung.
Discussion
The lack of significant effects of data source on relationships among composition and digestibility measures suggests that the data were determined by comparable methods, and thus acceptable to combine in an overall evaluation. The tendencies for data source effects noted for analyses with NDFD48 as the dependent variable could be related to differences between the laboratories in the in vitro fermentation procedures used, including sources of ruminal inoculum.
The correlations of ΔL, the lignins and NDFD48 were informative for assessment of their relationships including their differing behaviour. For all forages, the strong positive correlations between ADL and KL are in contrast to their correlations to NDFD48: correlations for ADL were consistently negative, whereas those for KL were both positive and negative (Table 4). The differing relationships to NDFD indicate nutritional non-equivalence between KL and ADL.
Increases in ADL concentrations in forages are associated with increased plant maturity(Reference Bidlack and Buxton22) and decreased NDFD(Reference Smith, Goering and Gordon23). The decline in ΔL/KL with increasing ADL in the grasses suggests a conversion of ΔL into ADL and net KL as they mature. This has some support in the finding that the grass samples with the greatest proportion of KL as ΔL (>70 %) also had lower concentrations of ADL, and so likely represented more immature grasses. Unfortunately, the dates of harvest and descriptions of forage maturity stages are lacking for samples used in the present study. The impact of plant maturity needs further investigation.
The relationship of ΔL/KL with NDFD provides further insights into the relationships of KL and ADL to NDFD. Because KL is the sum of ADL and ΔL, the sum of ΔL/KL and ADL/KL is a constant 100 % with the correlation between them negative and perfect (r −1·00, P < 0·001). For example, the rise against ADL in values of KL and almost constant ΔL noted in Fig. 1(a) and (c) represents a decline in proportion of ΔL as a part of KL and an increase in the proportion of ADL. Accordingly, a correlation of positive sign for ΔL/KL such as that noted with NDFD48 (Fig. 3) will have the opposite negative value with ADL/KL. The variable relationship of NDFD48 with KL noted by Jung et al. (Reference Jung, Mertens and Payne21) can be explained on the basis that KL is a composite of ADL and ΔL which have opposite relationships with NDFD; whichever, subfraction predominates will alter the relationship of KL with NDFD48. The positive correlations of ΔL/KL with NDFD48 do not indicate any causative effect upon NDFD48 and are a result of the interaction where the proportion of ΔL declines with increasing ADL (Fig. 2), which itself is negatively correlated with NDFD48. The positive correlations of ΔL/KL with NDFD48 indicate no inhibition of ΔL on NDF digestibility, although soluble lignin likely included in ΔL is apparently itself indigestible(Reference Neilson and Richards24).
The case for the relationship of ΔL/KL with NDFD48 in legumes is unclear. This may be because the data set from Cornell was a study with varieties of the same stage of growth, which possibly minimises variation in lignification and proportions of ΔL and ADL associated with plant maturation within cultivar. The number of samples in the Jung data set is too small for any conclusions. The results in Figs. 2 and 3 show much scatter and no significance. The proportion of ΔL in KL is smaller in alfalfa than in grasses evaluated in the present study (Table 1). There is evidence that KL present in grass and legume forages differs chemically, and so may their impact on NDFD. Sodium hydroxide treatment results in reductions in KL for stem material from smooth bromegrass (Bromis inermis; C3) and maize (Zea mays; C4), but not for alfalfa(Reference Jung, Valdez and Hatfield25).
The composition of ΔL has been overlooked because it has been treated as error, and there has been little study of its composition. However, there are some constraints to its composition. Soluble low molecular weight phenolics are not included because they are not precipitable and thus not gravimetrically recovered in KL(Reference Sluiter, Hames and Ruiz26). The precipitate produced in KL upon dilution and autoclaving is added in the KL but likely absent from ADL. This is likely the major component of ΔL and appears to be a major soluble fraction.
The phenolic character of ΔL justifies its inclusion as part of KL, a lignin assay intended to describe the total lignin content of materials. A physicochemical characteristic of ΔL is its apparent solubility in several in vitro and in vivo systems. Acid-detergent extraction and the ADL analysis procedure remove an average of 50 % KL. The action of fungal or microbial enzymatic digestion in the rumen or in vitro (Reference McSweeney, Dulieu and Katayama11,Reference Lowry, Conlan and Schlink13,Reference Gaillard and Richards15,Reference Grabber, Ralph and Hatfield27) yields amounts of soluble lignin that are large proportions of KL. Gaillard & Richards(Reference Gaillard and Richards15) estimated that 50 % of KL was solubilised in rumen contents of a steer fed on Australian spear grass, which is the average amount of soluble lignin dissolved in the ADL procedure. This soluble fraction was recovered in faeces and appears to be undigested(Reference Neilson and Richards24). The rumen soluble fraction contains small amounts of protein and carbohydrate and was precipitated at pH 3 at cool temperatures(Reference Gaillard and Richards15). The soluble lignins not in ADL and detected in in vitro and in vivo systems potentially come from polymers that are not linked to the mass of ADF-associated lignin. They may be released through enzymatic or acid hydrolysis of carbohydrate or protein that link those lignins to the plant cell wall(Reference Gaillard and Richards15) or by solubilisation in detergents(Reference Lowry, Kennedy and Conlan8,Reference Lowry, Conlan and Schlink13) .
Other possible sources of ΔL of secondary importance can be mentioned. Furfurals are degradation products of carbohydrates created through the actions of heat and acid in the KL procedure(Reference Chi, Zhang and Chang28). Furfurals produce pseudo-lignin when they react with proteins(Reference Wildschut, Smit and Reith29). Earlier nutritional studies regarded the precipitate found on dilution and heating in the KL procedure as non-lignin matter(Reference Sullivan4,Reference Moon and Abou-Raya30) .
The relationships of NDFD48 with KL and ΔL as compared to that with ADL are a manifestation of the intent and complementarity of the assays. KL was designed to measure total lignin in wood products. In contrast, ADL, NDF and NDFD48 were designed as components within the detergent system of analysis to describe and evaluate the NDFD which contains the hemicellulosic and cellulosic portions of cell wall and is analogous to insoluble dietary fibre(Reference Mongeau and Brassard31). The detergent system was designed to be an internally consistent grouping of nutritionally relevant assays(Reference Van Soest20). Acid-detergent lignin was not designed to measure total lignin. Neither was NDF designed to measure total dietary fibre. Thus, using total lignin rather than just the portion present as ADL that is associated with the detergent fibres is apparently inappropriate as a predictor of NDFD.
The ΔL does not seem to have depressing effect on NDFD. These relationships are positive in the case of grasses but for legumes are indeterminate (Fig. 3). From the view of physicochemical principles, it must be asked how a soluble fraction can have negative effect on digestibility of an insoluble substance? In the case of tannins, it is abundantly clear that a complex with proteins exists; however, in the case of NDF and ΔL, there is no evidence. However, it is very possible that indigestible ΔL or soluble lignin could adversely affect the extent of total organic matter digestion, since these phenolics likely have no fermentable energy and dilute potentially digestible solubles such as sugars and fructans. This would result in an overvaluation of the energy of the detergent-solubilised organic matter. The ΔL amounts to an average of 7 % of DM in grass forages. Total neutral-detergent-soluble matter averages between 25 and 30 %. Thus, we speculate that roughly a quarter or more of grass forage neutral-detergent solubles (NDS) apparently possess no fermentable energy. This estimation is based on the presumed indigestibility of ΔL and in vivo work on the true digestibility of NDS(Reference Van Soest32,Reference Weisbjerg, Hvelplund and Søegaard33) . From an estimated 98 % true digestibility of NDS for forages and concentrates, a true indigestibility of 100 – 98 % = 2 % of NDS could be inferred(Reference Van Soest32). Further, numerical data extracted from published graphs with individual data points for grass and legume forages(Reference Van Soest32) and for diets with 40 % or less of diet DM as NDS(Reference Weisbjerg, Hvelplund and Søegaard33) gave linear regressions with slopes equal to the true digestibility of NDS of 92·4 and 98·9 %, respectively, with calculated true indigestibility values of 7·6 and 1·1 %. The scatter of the data around the regression lines in these studies speaks to variability in both the digestibility of different NDS sources and the in vivo study measurements. The impact of this potential ΔL-mediated dilution on digestibility of NDS could be negated in diet formulation through the use of measured carbohydrate and protein fraction values rather than a by-difference value which encompasses unmeasured fractions and analytical error(1). A common recommendation is to adapt ruminants and their microbiota to the diets that will be evaluated during in vivo and in vitro digestion studies. It would be of interest to discover if the acclimation of microbiota to new diets includes adaptation to the ΔL of that diet. Further study on nutritional effects of soluble lignins is needed.
Strengths and limitations
A strength of the present study was the use of a mathematical approach to parse KL for evaluation of its nutritional characteristics. The greatest limitation to interpretation of the present work is the lack of information on the composition of ΔL. That information is needed to provide a biological context for the different relationships of fibre digestibility and the empirical lignin assays. Although the samples included in the present study spanned a wide range in terms of composition, data on a greater number of samples of varied compositions, particularly of the alfalfas, would allow more definitive findings regarding the lignin relationships with NDFD48.
Conclusions
The material that has been identified as KL is not a uniform fraction in forage plants and consists of at least two components, ADL and ΔL, which have differing associations with NDFD. For grasses, the positive relationship between ΔL/KL and NDFD at 48 h indicates that ΔL has no negative effect on NDFD and indicates that the ADL component of KL represents the main negative factor affecting NDFD and forage quality. The strong negative association of ΔL/KL with ADL is suggestive of the conversion of ΔL to ADL as forages mature and KL content increases. The situation with alfalfa is less clear. ADL is more consistently related to indigestibility of NDF than is KL for application to ruminant nutrition. The heterogeneous nature of KL would also potentially recommend against its use as a marker for dietary fibre indigestibility as has been recommended for humans(Reference Theander, Aman and Westerlund3).
Acknowledgements
The present work was supported with funds from Cornell University and United States Department of Agriculture – Agricultural Research Service. No external grant funds supported the present work.
P. J. V. S. conceived and designed the research. J. B. R. conducted laboratory analyses and reviewed the manuscript. P. J. V. S., M. B. H. and M. C. B. collected data, performed statistical analysis, wrote the paper and had primary responsibility for final content. All authors, except J. B. R., read and approved the final manuscript.
The authors declare that there are no conflicts of interest.