Dyslipidaemia is one of the major determinants of the development of CVD(Reference Davidson1, Reference Cannon2). Postprandial lipaemia has emerged as a key contributor to the risk and progression of CVD. Elevated levels of non-fasting TAG are strongly associated with increased risk of myocardial infarction, ischaemic stroke and early death(Reference Nordestgaard, Langsted and Freiberg3).
Proanthocyanidins (PA), the most abundant polyphenols in human diets, have been shown to improve postprandial hypertriacylglycerolaemia in animal models(Reference Blade, Arola and Salvado4), and the ingestion of PA-rich food, such as red wine, has been shown to decrease plasma lipids in human subjects(Reference Zern, Wood and Greene5). The hypolipidaemic action of grape seed proanthocyanidin extract (GSPE) is attributable to a reduction in plasma levels of TAG-rich lipoproteins and to an improvement of the serum cholesterol profile, both in normolipidaemic(Reference Del Bas, Fernandez-Larrea and Blay6) and dyslipidaemic rats(Reference Quesada, del Bas and Pajuelo7). Additionally, the simultaneous ingestion of apple PA with fat inhibits the increase in plasma TAG levels induced by fat ingestion in mice and human subjects(Reference Sugiyama, Akazome and Shoji8).
Plasma TAG levels are the result of the balance between TAG-rich lipoprotein secretion by the intestine and liver and its uptake by the extrahepatic tissues through lipoprotein lipase (LPL). TAG-rich lipoproteins in plasma originate in either the liver (VLDL) or the intestine (chylomicrons, CM). The hypotriacylglycerolaemic effect of PA has been attributed to an inhibition of dietary lipid absorption associated with a reduction in CM secretion by enterocytes(Reference Sugiyama, Akazome and Shoji8, Reference Vidal, Hernandez-Vallejo and Pauquai9) as well as a decrease in VLDL secretion by the liver(Reference Quesada, del Bas and Pajuelo7, Reference Del Bas, Ricketts and Baiges10–Reference Baiges, Palmfeldt and Blade12) in a range of different studies. Nevertheless, the real contribution of CM and VLDL secretion and LPL activity to the hypotriacylglycerolaemic action of GSPE has never been studied. Thus, the aim of the present study was to determine the contribution of CM and VLDL in the hypotriacylglycerolaemic action of GSPE in the postprandial state and to characterise the principal mechanisms by which GSPE treatment reduces TAG-rich lipoprotein levels.
Materials and methods
Proanthocyanidin extract
The PA extract (GSPE) contained monomeric (21·3 %), dimeric (17·4 %), trimeric (16·3 %), tetrameric (13·3 %) and oligomeric (5–13 units; 31·7 %) PA.
Animals
Male Wistar rats that weighed 350 g were purchased from Charles River (Barcelona, Spain). All experiments were conducted in conformity with the Public Health Service Policy on Humane Care and Use of Laboratory Animals policy, and the studies were approved by the Animal Ethics Committee of our university. The animals were housed in animal quarters at 22°C under a 12 h light–12 h dark cycle (light from 08.00 to 20.00 hours) and were provided standard chow (Panlab, Barcelona, Spain) and water ad libitum.
Plasma lipid tolerance test and measurement of plasma lipid levels
For the lipid tolerance test, rats were deprived of food for 14 h before the experiment. Lard oil (2·5 ml/kg of body weight) with or without GSPE (250 mg/kg of body weight) was administered orally at 9 h. For blood chemical analyses, aliquots from the tail vein were collected into capillary tubes with EDTA after 1, 3, 5 and 7 h of treatment. TAG and cholesterol levels were determined using an enzymatic colorimetric kit (QCA, Barcelona, Spain). NEFA levels were determined using the Wako assay kit (Wako Chemicals GmbH, Neuss, Germany). 3-Hydroxy-butyrate was analysed using an enzymatic kit (Ben S.r.l., Milano, Italy).
Lipoprotein fractionation
Lipoprotein fractions were prepared from plasma samples by means of density gradient ultracentrifugation(Reference Schumaker and Puppione13, Reference Havel, Eder and Bragdon14). A total volume of 2 ml was put in the bottom of a 6·5 ml polyallomer ultracentrifuge tube (Beckman, Brea, CA, USA). Then, 3 ml of 1·006 g/ml density solution was added, and the sample was centrifuged in a 45·6 Kontor rotor. CM was subfractionated by a 30 min centrifugation at 16 000 rpm, and VLDL was subfractionated by a 16 h centrifugation at 37 000 rpm. After each centrifugation step, 2 ml of the solution at the top of the gradient, which contained the respective lipoprotein subclass, was aspirated and 2 ml of density solution was used to refill the tube before the next run.
The TAG content was determined using an enzymatic colorimetric kit (QCA) in both lipoprotein fractions.
VLDL-TAG secretion assay
Rats were fasted for 3 h and then fed with an oral administration of GSPE (250 mg/kg of body weight) in aqueous solution (treated group) or an oral administration of the vehicle (tap water; control group). The duration of treatment was 2 h. Rats were injected with 500 mg/kg Triton WR 1339 (Sigma-Aldrich, St Louis, MO, USA) into the tail vein to inhibit plasma VLDL-TAG hydrolysis and clearance(Reference Qu, Perdomo and Su15). Aliquots of saphenous blood were collected at 0, 10, 20, 30, 40, 50 and 60 min after the Triton WR 1339 injection. Plasma TAG levels were determined using an enzymatic colorimetric kit (QCA) and plotted as a function of time.
Lipoprotein lipase assay
Rats were deprived of food for 14 h before the experiment. Lard oil (2·5 ml/kg) with or without GSPE (250 mg/kg of body weight) was administered orally. At 1 h after the administration, rats were injected with 300 IU heparin/kg body weight intravenously, and saphenous vein blood was sampled 10 min after the heparin infusion. Heparinised plasma was prepared for the determination of LPL activity with the LPL activity kit (Roar Biochemical Inc., New York, NY, USA), as described by the manufacturer.
Carnitine palmitoyltransferase 1 assay
Rats were initially treated as described in the plasma lipid tolerance test section; later, rats were anaesthetised using ketamine/xylazine and killed by exsanguination. The liver was excised, immediately frozen in liquid N2 and stored at − 80°C until the activity assay was performed.
Mitochondria were isolated from the livers, which were homogenated at 4°C in a 1:3 ratio (w:v) of a buffer containing 250 mm-sucrose, 1 mm-EDTA and 10 mm-Tris–HCl (pH 7·4) with a Teflon/glass homogeniser and then centrifuged at 700 g for 10 min at 4°C. The supernatants were centrifuged at 12 000 g for 10 min at 4°C. The resulting pellet containing the purified mitochondria was resuspended in 500 μl of a buffer with 70 mm-sucrose, 220 mm-mannitol, 2 mm-HEPES and 1 mm-EDTA(Reference Fuster, Oliver and Sanchez16) and used to perform the carnitine palmitoyltransferase (CPT) 1a assay. Bradford reagent (Sigma-Aldrich) was used to determine the mitochondrial protein content.
CPT-1 activity was assessed as described by Bieber et al. (Reference Bieber, Abraham and Helmrath17). Assays were performed in triplicate, and 13 μl of the mitochondrial protein solution was transferred into the assay buffer (116 mm-Tris–HCl, 0·09 % Triton X-100, 1·1 mm-EDTA, 0·035 mm-palmitoyl-coenzyme A (CoA) and 0·12 mm-5,5′-dithiobis-2-nitrobenzoic acid (pH 8·0)) and incubated for 3 min at 20°C. Absorbance was then measured at 412 nm. The results are expressed in nmol CoA formed/mg protein per min.
Gene expression analysis
Rats were initially treated as described in the plasma lipid tolerance test section; later, rats were anaesthetised using ketamine/xylazine and killed by exsanguination. The first 30 cm of the intestinal mucosa, liver, leg muscle and visceral white adipose tissue was excised and immediately frozen until RNA extraction. Total RNA was isolated using an RNeasy Mini kit (Qiagen, Hilden, Germany) according to the manufacturer's instructions. Complementary DNA was synthesised from 2 μg of total RNA with the High Capacity complementary DNA Reverse Transcription Kit (Applied Biosystems, Madrid, Spain).
Changes in mRNA expression of LPL in the muscle and white adipose tissue were measured by quantitative PCR. Quantitative PCR amplification and detection were performed using a specific TaqManR Assay-On-Demand probe (Applied Biosystems; Rn00561482_m1), the TaqMan PCR Core Reagent Kit and the GeneAmpR 5700 Sequence Detection System, as recommended by the manufacturers. Quadruplicated quantifications, performed in singleplex assays, were performed for each complementary DNA. PPIA was used as the reference gene in quantitative PCR (Applied Biosystems; TaqManR Assay-On-Demand probe Rn00690933_m1).
Each complementary DNA sample of the liver and intestine (100 μl) was added to an equal volume of 2 × TaqMan Universal PCR Master Mix (Applied Biosystems). After gentle mixing and centrifugation, the mixture was then transferred into a loading port on a low-density array card (TLDA; Applied Biosystems). Each TLDA had eight separated loading ports that feed into forty-eight gene sets. Each 2 μl well contained specific user-defined probes capable of detecting a single gene. Each set of forty-eight genes also contained PPIA as a housekeeping gene. The array was centrifuged twice for 1 min, each at 1200 rpm, to distribute the samples from the loading port into each well. The card was then sealed, and PCR amplification was performed using an Applied Biosystems Prism 7900HT sequence detection system. Thermal cycling conditions were as follows: 2 min at 50°C, 10 min at 94·5°C, 30 s at 97°C and 1 min at 59·7°C for forty cycles(Reference Steg, Wang and Blanquicett18).
Statistical analysis
Results are reported as mean values with their standard errors of ten animals in the case of plasma TAG, three animals for RNA levels and five animals for other analyses. Group mean values were compared with an independent sample Student's t test, except for those of gene expression, which were compared with one-way ANOVA (P ≤ 0·05) using SPSS software (SPSS version 17.0; IBM, New York, NY, USA).
Results
Grape seed proanthocyanidin extract treatment blocked the increase in plasma total TAG by reducing VLDL-TAG or chylomicron-TAG in a time-dependent manner
In control animals, plasma TAG reached their maximum levels 3 h after the oral administration of 2·5 ml/kg of lard oil, after which it decreased (Fig. 1(a)). The administration of GSPE (250 mg/kg body weight) markedly blocked the increase in plasma TAG, with a statistically significant reduction of 22 % in the area under the curve of plasma TAG (Fig. 1(b)). Thus, GSPE treatment improved the tolerance to oral TAG. The hypotriacylglycerolaemic effect of GSPE was extremely rapid (it could be noted after 1·5 h), and it was observable until 7 h after the administration.
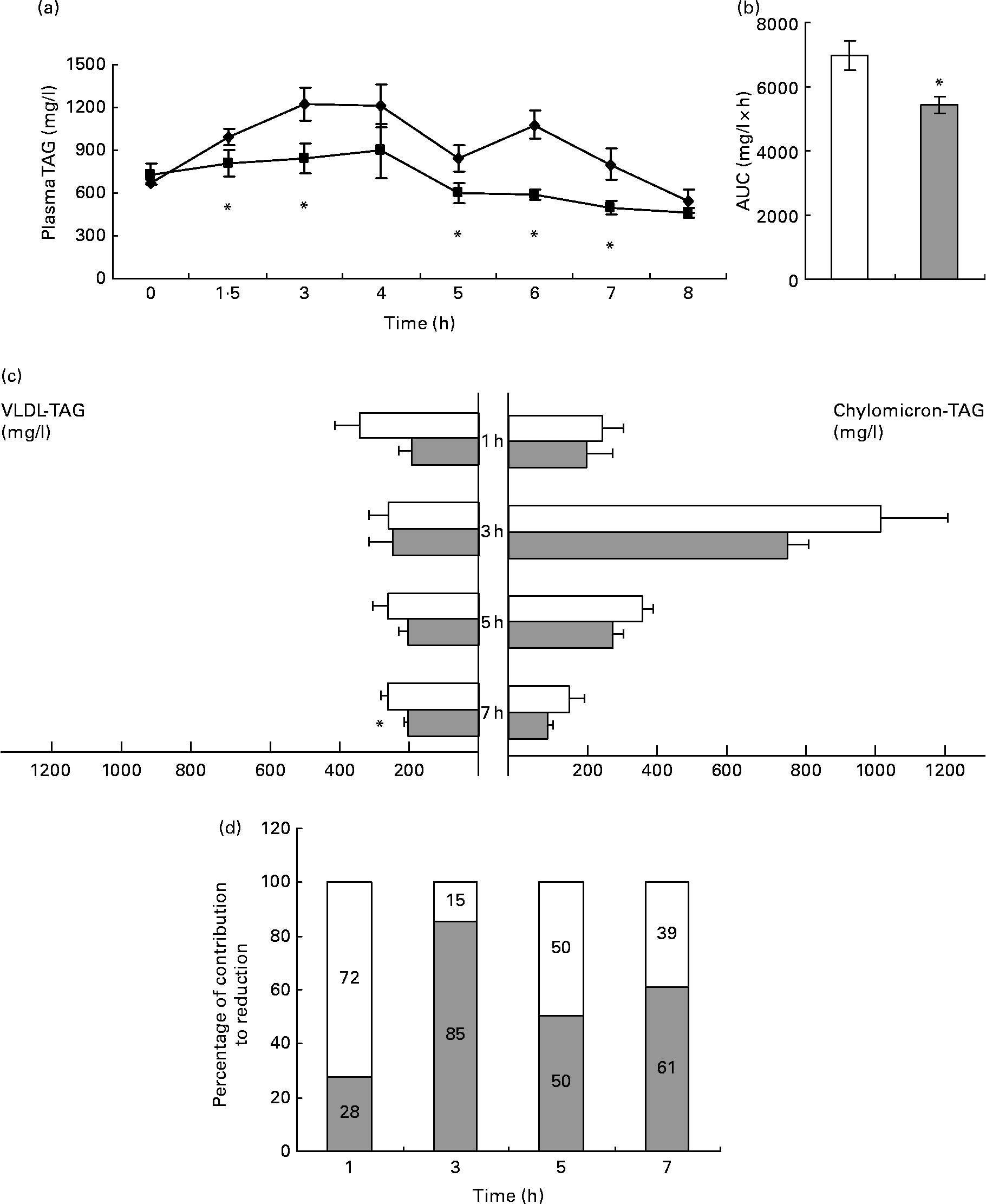
Fig. 1 TAG levels in plasma and TAG content in chylomicron (CM)-rich and VLDL-rich fractions of rats fed on lard oil with or without proanthocyanidins (grape seed proanthocyanidin extract, GSPE). Rats fasted for 14 h were administered lard oil (2·5 ml/kg) with or without GSPE (250 mg/kg) orally. (a) Plasma TAG were quantified in blood samples of the tail vein before (0 h) and after 1·5, 3, 4, 5, 6, 7 and 8 h of the administration. ◆, Lard; ■, lard+GSPE. (b) The y-axis represents the values of the area under the curve. (c) TAG content in CM-rich and VLDL-rich fractions were quantified in plasma from rats killed 1, 3, 5 and 7 h after the administration. □, Lard; , lard+GSPE. (d) Contribution to the reduction in percentage of VLDL (□)-TAG and CM (
)-TAG to plasma TAG at each time studied. Values are means with their standard errors represented by vertical or horizontal bars (n 10 for plasma TAG and of n 5 for CM and VLDL TAG). * Mean values were significantly different between the lard group and the lard+GSPE group at the same time (P < 0·05; Student's t test).
To better understand the contribution of CM and VLDL to the hypotriacylglycerolaemic effect of GSPE, we measured the TAG content of the CM-rich and VLDL-rich fractions (Fig. 1(c)). GSPE treatment slightly reduced CM-TAG at all studied time periods, showing its strongest effect after 3 h of treatment when CM-TAG levels peaked. GSPE treatment rapidly reduced VLDL-TAG levels (1 h after the administration), and the reduction became significant after 7 h. When the same values were expressed as a percentage of the total TAG reduction at each time point (Fig. 1(d)), it was obvious that both lipoproteins have a different pattern. The VLDL-rich fraction was responsible for 72 % of the TAG reduction at 1 h, whereas the CM-rich fraction was responsible for 85 % of the TAG reduction at 3 h. Altogether, these results indicate that both CM and VLDL contributed to the hypotriacylglycerolaemic action of GSPE, but their influence depended on time. The CM-rich fraction was the major contributor after 3 h of treatment, whereas the VLDL-rich fraction was the major contributor after 1 h. At 5 and 7 h after treatment, the CM-rich and VLDL-rich fractions showed a similar influence.
Plasma cholesterol levels showed almost the same pattern as plasma TAG levels in animals treated with only lard oil (Fig. 2(a)), but GSPE had no effect on plasma cholesterol content.

Fig. 2 Plasma levels of NEFA, cholesterol and 3-hydroxy-butyrate (3-OH-butyrate) of rats fed on lard oil with or without proanthocyanidins (grape seed proanthocyanidin extract, GSPE). Rats fasted for 14 h were administered lard oil (□; 2·5 ml/kg) with or without GSPE (; 250 mg/kg) orally. (a) Plasma cholesterol, (b) NEFA and (c) 3-OH-butyrate were quantified from the plasma of rats killed 1, 3, 5 and 7 h after the administration. Values are means with their standard errors represented by vertical bars (n 5). * Mean values were significantly different between the lard group and the lard+GSPE group at the same time (P < 0·05; Student's t test).
GSPE administration significantly reduced NEFA levels (Fig. 2(b)) 5 h after its administration. 3-Hydroxy-butyrate was significantly reduced 7 h after the administration of GSPE (Fig. 2(c)).
Grape seed proanthocyanidin extract treatment did not affect TAG clearance
Plasma TAG levels could be reduced by repressing TAG-rich lipoprotein secretion and/or increasing their uptake by the extrahepatic tissues. Thus, to characterise the mechanism by which GSPE treatment decreases VLDL-TAG and CM-TAG levels, we quantified TAG clearance. As GSPE affected plasma TAG levels rapidly, we measured plasma post-heparin LPL activity after 1 h of oral administration of lard oil or lard oil+GSPE. As shown in Fig. 3(a), GSPE had no effect on plasma LPL activity. Moreover, no differential expression of LPL was observed as a result of GSPE treatment in white adipose or muscle at 1 or 5 h after administration (Fig. 3(b) and (c)). Thus, these results strongly suggest that the blockage of the increase in plasma TAG levels induced by GSPE is not attributable to increased TAG clearance.

Fig. 3 Post-heparin plasma lipoprotein lipase (LPL) activity and mRNA levels of LPL in white adipose tissue and muscle of rats fed on lard oil with or without proanthocyanidins (grape seed proanthocyanidin extract, GSPE). (a) Post-heparin plasma LPL activity was quantified an hour after the administration of lard oil (2·5 ml/kg) with or without GSPE (250 mg/kg) to rats fasted for 14 h and injected 300 IU heparin/kg body weight intravenously. mRNA levels of LPL in (b) white adipose tissue and (c) muscle were determined 1 and 5 h after the administration of lard oil (□; 2·5 ml/kg) with or without GSPE (; 250 mg/kg) to rats fasted for 14 h. The basal state (■; 0 h) corresponds to mRNA values before the oral administration. The gene expression values are expressed as fold changes using PPIA expression as the endogenous control. Values are means with their standard errors represented by vertical bars (n 3 for mRNA LPL and n 5 for the LPL activity). * Mean values were significantly different between the lard group and the lard+GSPE group at the same time (P < 0·05; Student's t test).
Grape seed proanthocyanidin extract administration decreased VLDL-TAG secretion
To assess VLDL-TAG secretion, we injected the rats with Triton WR 1339, which inhibits VLDL-TAG hydrolysis and clearance. VLDL-TAG secretion was measured 2 h after GSPE administration in rats that were fasted for 5 h to avoid CM secretion (Fig. 4). GSPE treatment significantly repressed (30 %) the secretion of VLDL-TAG. As VLDL is mainly secreted by the liver, these results strongly suggest that the decrease in plasma VLDL-TAG levels induced by GSPE is due to GSPE action in the liver, which in turn represses VLDL-TAG secretion.

Fig. 4 VLDL-TAG secretion in rats treated with proanthocyanidins (grape seed proanthocyanidin extract, GSPE). VLDL-TAG secretion was evaluated in rats fasted for 5 h. Rats were administered GSPE (250 mg/kg) or water orally and injected with 500 mg/kg of Triton WR 1339 through the tail vein to inhibit plasma TAG clearance. Aliquots of saphenous blood were collected at 0, 10, 20, 30, 40, 50 and 60 min after Triton WR 1339 injection. Values are means with their standard errors represented by vertical bars (n 5). * Mean values were significantly different (P < 0·05; Student's t test).
Grape seed proanthocyanidin extract treatment modulated the expression of some lipid-related genes in the liver but not in the intestine
To determine the molecular mechanisms underlying TAG-rich lipoprotein secretion, we used a TLDA to analyse the differential expression of key genes that control TAG and cholesterol metabolism in the intestine and liver at 0 (before treatment, basal state), 1 and 5 h after lard oil or lard oil +GSPE administration. We chose the following genes that encode key proteins in lipid pathways: for cholesterol metabolism, the intestinal uptake transporter Niemann-Pick C1-like protein 1 (Npc1l1), the ATP-binding cassettes implicated in the intestinal and biliary excretion of sterols (Abcg5 and Abcg8), ATP-binding cassette sub-family A member 1 (Abca1), the enzyme controlling cholesterol synthesis 3-hydroxy-3-methylglutaryl-CoA reductase (Hmgcr) and the enzyme acetyl-CoA acetyltransferase 2 (Acat2), which catalyses the conversion of free cholesterol to cholesteryl esters; for fatty acid (FA) metabolism, the FA transporter CD36, the FA binding protein 1 (Fabp1), the enzyme controlling β-oxidation carnitine palmitoyltransferase 1a (Cpt1a), the enzyme FA synthase (Fasn), the enzyme controlling the synthesis of unsaturated FA stearoyl-CoA desaturase 1 and the enzyme acyl-CoA synthetase long-chain family member 1 (Acsl1), which catalyses the ligation of long chain FA with CoA to produce long chain acyl-CoA; for TAG synthesis, the Mg2+-dependent PA phosphatase lipin 1 (Lpin1) and the diacylglycerol O-acyltransferase homologues 1 and 2 (Dgat1 and Dgat2), which catalyse the final step of TAG synthesis; and for lipoprotein assembling and composition, microsomal TAG transfer protein (Mttp), Apoa5 and Apoc3. We also selected the nuclear receptors farnesoid X receptor (Fxr) and small heterodimer partner (Shp) and the transcription factor sterol regulatory element-binding protein (Srebp)-1c, because they govern the expression of key lipid metabolism genes in the intestine and the liver and are involved in the molecular mechanism used by GSPE in the liver(Reference Del Bas, Ricketts and Baiges10, Reference Del Bas, Ricketts and Vaque11).
In the intestine (Table 1), the ingestion of lard induced a generalised repression of genes related to lipid metabolism, excluding acetyl-CoA acetyltransferase 2, which was overexpressed. GSPE did not induce different effects from those induced by lard oil alone on the genes studied at both times, except Cpt1a, which was repressed 5 h post-administration.
Table 1 mRNA levels of genes related to lipid metabolism in the intestinal mucosa of rats fed on lard oil with or without proanthocyanidins (grape seed proanthocyanidin extract; GSPE) at 1 h and 5 h§
(Mean values with their standard errors for three rats)

* Mean value was significantly decreased from basal (P < 0·05).
† Mean value was significantly increased from basal (P < 0·05).
‡ Mean value was significantly difference (P < 0·05) from that of the lard group at 5 h (P < 0·05; ANOVA).
§ Rats fasted for 14 h were administered lard oil (2·5 ml/kg) with or without GSPE (250 mg/kg) orally and were killed at 1 h or 5 h after the administration. The basal state (0 h) corresponds to mRNA values before the oral administration. The values are expressed as fold change using PPIA expression as the endogenous control. For gene names, see the ‘Grape seed proanthocyanidin extract treatment modulated the expression of some lipid-related genes in the liver but not in the intestine’ section.
Table 2 shows the mRNA levels of genes related to lipid metabolism in the liver, except for those of Cpt1a, which have been included in Table 3. At 1 h after the administration of lard oil, the livers showed significant overexpression of lipin 1 in conjunction with significant repression of Acsl1 and Dgat1 relative to their basal levels. The livers of rats treated with GSPE presented the same expression pattern for lipin 1 and Dgat1 at the 1 h mark. On the other hand, GSPE treatment induced different effects on the expression Acsl1, Apoc3 and Hmgcr compared to those induced by lard oil treatment. After GSPE treatment, Acsl1 was not repressed, Apoc3 was repressed and Hmgcr was overexpressed relative to their basal levels.
Table 2 mRNA levels of genes related to lipid metabolism in the liver of rats fed on lard oil with or without proanthocyanidins (grape seed proanthocyanidin extract; GSPE) at 1 h and 5 h‡
(Mean values with their standard errors for three rats)

* Mean value was significantly decreased from basal (P < 0·05).
† Mean value was significantly increased from basal (P < 0·05).
‡ Rats fasted for 14 h were administered lard oil (2·5 ml/kg) with or without GSPE (250 mg/kg) orally and were killed at 1 h or 5 h after the administration. The basal state (0 h) corresponds to mRNA values before the oral administration. The values are expressed as fold change using PPIA expression as the endogenous control. For gene names, see the ‘Grape seed proanthocyanidin extract treatment modulated the expression of some lipid-related genes in the liver but not in the intestine’ section.
Table 3 Carnitine palmitoyl transferase 1 expression and activity in the liver of rats fed on lard oil with or without proanthocyanidins (GSPE)‡
(Mean values with their standard errors for five rats for Cpt1 activity and three rats for Cpt1 mRNA)

* Mean value was significantly difference (P < 0·05) from that of the lard group at 5 h (P < 0·05; Student's t test).
† Mean value was significantly decreased from basal (P < 0·05).
‡ Rats fasted for 14 h were administered lard oil (2·5 ml/kg) with or without GSPE (250 mg/kg) orally and were killed at 1 h or 5 h after the administration. The basal state (0 h) corresponds to mRNA values before the oral administration.
However, 5 h after the administration of lard oil, the livers showed significant repression of Dgat1, Acsl1, Mttp and Shp in conjunction with significant overexpression of Cd36 relative to their basal levels. The livers of rats treated with GSPE presented the same expression pattern for Dgat1, Acsl1, Mttp and Shp after 5 h. Nevertheless, GSPE treatment induced different effects from those induced by lard oil treatment on the expression of Cd36.
Grape seed proanthocyanidin extract treatment channelled fatty acid into β-oxidation in the liver immediately (1 h)
The relationship between FA oxidation and esterification in the liver has been described as a key factor in the regulation of VLDL synthesis(Reference Julius19). As enzymes related to FA esterification (Dgat1 and 2) were not differentially expressed in response to GSPE, we performed an in-depth study of the effects of lard oil with or without GSPE on FA oxidation. To the present aim, both the mRNA expression and activity of Cpt1a, which is the rate-limiting enzyme in β-oxidation, were determined at different time periods post-administration (Table 3). Lard oil administration significantly repressed the expression of Cpt1a and decreased its activity by 60 % at 1 h after its ingestion. In contrast, when lard oil was administered with GSPE, there was no repression of Cpt1a, and its activity remained high. At 5 h after the administration, the expression pattern was opposite of that observed after 1 h. Cpt1a expression had recovered in the livers of rats treated with lard oil, whereas in the livers of rats treated with lard oil +GSPE, Cpt1a expression and activity were repressed. These results suggest that GSPE treatment channelled FA into β-oxidation shortly after treatment initiation.
Discussion
Several authors have reported the hypotriacylglycerolaemic effects of PA in human subjects and animals (reviewed in Blade et al. (Reference Blade, Arola and Salvado4)). Plasma TAG levels are the result of the balance between the TAG-rich lipoprotein secretion by the intestine and the liver, and its uptake by the extrahepatic tissues through LPL. Therefore, GSPE may reduce plasma TAG levels by acting on the intestine, liver and/or peripheral tissues. However, the exact role of each organ remains unknown. For this reason, the present study intended to quantify the contribution of CM and VLDL production and LPL activity to the hypotriacylglycerolaemic action of PA in vivo. With this aim, we analysed the effects of GSPE on plasma lipids and lipoprotein kinetics during a fed state with a lipid tolerance test(Reference Cohn20). To our knowledge, this is the first study performed to measure the contribution of CM and VLDL to hypotriacylglycerolaemia induced by PA or other flavonoids.
The present results show that the oral intake of GSPE significantly blocked the increase in plasma TAG levels induced by lard oil ingestion in control animals. This blockade of plasma TAG has also been described with PA from apples(Reference Sugiyama, Akazome and Shoji8) in mice loaded with maize oil, in which the blockade was as rapid as that observed in the present experiment (1 h). In the present study, the reduction in plasma TAG levels was similar to the reductions observed in male normolipidaemic rats(Reference Del Bas, Fernandez-Larrea and Blay6) and mice(Reference Del Bas, Ricketts and Baiges10, Reference Del Bas, Ricketts and Vaque11) after performing acute GSPE treatment and in dyslipidaemic rats(Reference Quesada, del Bas and Pajuelo7) after performing chronic GSPE treatment. Thus, the improvement of plasma TAG levels is a generalised effect of PA, as it is observed in different situations and experimental approaches. Consequently, PA-rich foods may alleviate the hypertriacylglycerolaemia associated with the postprandial state and may therefore improve tolerance to dietary lipids.
Both CM-rich and VLDL-rich fractions contributed to the hypotriacylglycerolaemic action of GSPE, but their influence was time dependent. CM-rich fraction was the main contributor 3 h post-treatment, whereas VLDL-rich fraction was important 1 h post-treatment. As GSPE did not increase TAG clearance by extrahepatic tissues, the reductions of CM-TAG and VLDL-TAG levels induced by GSPE could be ascribed to repressed lipoprotein secretion. It is generally assumed that CM predominantly transports exogenously ingested TAG derived from dietary sources, whereas VLDL transports endogenously synthesised lipids(Reference Gibbons, Wiggins and Brown21). Therefore, PA repress the secretion of both synthesised, endogenous TAG and TAG absorbed from the diet.
Several authors have reported that CM and lipid absorption cause the hypolipidaemic actions of PA, but only on the basis of indirect evidence or in vitro experiments(Reference Sugiyama, Akazome and Shoji8, Reference Vidal, Hernandez-Vallejo and Pauquai9). The expression of the selected genes related to FA and TAG metabolism as well as those of the CM assembly was not modified in the intestinal mucosa at 1 or 5 h after GSPE administration. Therefore, other genes and/or molecular mechanisms could be involved in this repression, and future studies are warranted.
We did not determine the secretion of CM-TAG. However, TAG secretion associated with VLDL was repressed up to 30 % by GSPE treatment. Thus, the repression of VLDL secretion appears to be an important contributor to the hypotriacylglycerolaemic effect of PA. The liver is the primary organ that secretes VLDL(Reference Ginsberg and Fisher22, Reference Olofsson, Bostrom and Andersson23). Consequently, regarding the hypotriacylglycerolaemia induced by PA in the postprandial state, the liver is a significant target for GSPE.
The regulation of hepatic VLDL secretion mainly depends on apoB synthesis, MTTP activity and lipid availability(Reference Julius19). The present results suggest that the unavailability of lipids is the primary cause of the reduction of VLDL-TAG secretion by the liver. Shortly after GSPE administration (1 h), CPT-1a activity and expression remained high, suggesting elevated FA oxidation. A pattern similar to that of CPT-1a was observed for Acsl1 mRNA. Specific ACSL isoforms provide acyl-CoA for particular metabolic pathways(Reference Soupene and Kuypers24), and Acsl1 has been reported to have a role in mitochondrial β-oxidation in knockout models of ACSL1. The relationship between FA oxidation and esterification has been described as a key factor in the regulation of VLDL synthesis(Reference Julius19). Therefore, the increased oxidation of FA induced by GSPE treatment is a potential mechanism by which PA reduce VLDL-TAG secretion shortly after GSPE administration (1 h).
In contrast, 5 h after GSPE administration, the levels of plasma NEFA decreased considerably. As plasma NEFA is a source for TAG synthesis in the liver and has an important role in stimulating hepatic VLDL production(Reference Julius19, Reference Duez, Lamarche and Valero25), the reduction in plasma NEFA levels could be the mechanism hidden behind the repression of VLDL-TAG secretion induced by GSPE several hours after its administration. Moreover, concomitant to the decrease in plasma NEFA levels, GSPE treatment changed the expression pattern of Cd36 induced by lard alone. Hepatic Cd36 controls FA uptake by the liver, and its elevation in expression directly affects hepatic FA uptake, TAG storage and VLDL-TAG secretion(Reference Koonen, Jacobs and Febbraio26). Therefore, reduced NEFA levels in plasma, together with low levels of Cd36 expression, may result in decreased FA availability for TAG synthesis in the liver 5 h after GSPE administration, thus reducing VLDL-TAG secretion.
The described molecular mechanism by which GSPE represses hepatic TAG secretion involves the transcriptional activation of farnesoid X receptor(Reference Del Bas, Ricketts and Vaque11), overexpression of the nuclear receptor small heterodimer partner(Reference Del Bas, Ricketts and Baiges10) and repression of Srebp-1(Reference Quesada, del Bas and Pajuelo7, Reference Del Bas, Ricketts and Baiges10, Reference Del Bas, Ricketts and Vaque11). We found no significant difference in the expression of small heterodimer partner or Srebp-1 after administering lard alone or lard +GSPE. Nevertheless, mRNA levels of Srebp-1 were always lower after GSPE treatment, and small heterodimer partner mRNA levels were higher 5 h after GSPE administration. Diet and hormones, primarily insulin, regulate the transcription of Srebp-1(Reference Raghow, Yellaturu and Deng27). In the present experiment, the animals received only a TAG overload, whereas in the experiments that describe the molecular mechanism of GSPE, the animals received a mixed diet. Thus, the differences in the diets, which in the present case reduced the expression of Srebp-1 in control animals (without GSPE), minimised the differences between animals treated with GSPE and those treated with vehicle.
In conclusion, the present results show that the oral intake of GSPE significantly blocked the increase in plasma TAG levels induced by lard oil ingestion. Both CM-rich and VLDL-rich fractions contributed to the hypotriacylglycerolaemic action of GSPE, but their influence was time dependent. GSPE ingestion repressed VLDL-TAG secretion, but it did not increase TAG clearance. GSPE may block VLDL-TAG secretion because of the unavailability of FA for TAG synthesis, as a consequence of increasing FA oxidation shortly after administration and reducing plasma NEFA levels at later time periods. Overproduction of TAG-rich lipoproteins is characteristic of the dyslipidaemia in the metabolic syndrome and type 2 diabetes(Reference Adiels, Olofsson and Taskinen28, Reference Therond29). Therefore, blockage of the TAG-rich lipoprotein secretion induced by PA may explain the association between dietary intake of flavonoids and certain foods rich in flavonoids and a reduced risk of death due to CHD and CVD(Reference Mink, Scrafford and Barraj30).
Acknowledgements
The authors thank Leticia Quesada for revising the manuscript. The present study was supported by grant number AGL 2008-00387/ALI from the Spanish Dirección General de Investigación del Ministerio de Educación y Ciéncia. L. A. is a member of MITOFOOD. The authors gratefully acknowledge Mercedes Heras and Niurka Llopiz for their technical assistance. All authors participated in the conception, design and interpretation of data. H. Q., S. D, D. P. and A. F.-I. performed sample extraction and analysis. H. Q. and C. B. were involved in writing and editing the paper. All authors reviewed the final manuscript. The authors declare no conflicts of interest.