Obesity and its related metabolic disorders may prove to be the greatest threat to human lifestyle and health in the developed world this century(Reference Armitage, Poston and Taylor1). The incidence of obesity and overweight has risen markedly in Western societies and the trend is mirrored in developing nations that are transitioning to first-world economies and lifestyles. Of even more concern is the marked increase over the last decade in the prevalence of childhood obesity and concomitant increases in childhood type 2 diabetes. Metabolic disease results from a complex interaction of genetic, physiological, behavioural and environmental factors. There have been numerous hypotheses to explain the dramatic increase of obesity and metabolic disease, including increased food availability and reduced energy expenditures associated with changing work and/or leisure habits. The recent rate at which these diseases have increased suggests that environmental and behavioural influences, rather than genetic causes, are fuelling the present epidemic. A relationship has been established between the periconceptual, fetal and early-infant phases of life and the subsequent development of adult obesity and type 2 diabetes. This relationship, referred to as the ‘developmental origins of health and disease’ model, speculates that the fetus makes predictive adaptations in response to intra-uterine cues, resulting in adjustments in the homeostatic systems to aid survival and improve success in a potentially adverse postnatal environment. However, inappropriate interpretations of prenatal cues or changes to that immediate environment may result in a mismatch between prenatal predictions and postnatal reality.
By far, the largest body of evidence supporting an adverse early-life environment resulting in growth and metabolic compromise in later life has come from cohort studies of maternal famine or experimental manipulation of maternal nutritional status. Our previous studies in the rat utilising global maternal undernutrition have demonstrated that maternal nutrient restriction in pregnancy alone resulted in growth-restricted male and female offspring who, when subsequently provided with ad libitum standard laboratory nutrition, develop a classical metabolic syndrome phenotype characterised by obesity, hypertension, hyperleptinaemia and hyperinsulinaemia and early-onset puberty(Reference Vickers, Gluckman and Coveny2–Reference Sloboda, Howie and Pleasants6). While the final health consequences of nutritional programming are similar, the mechanisms underlying the development of disease vary according to the nature, duration and timing of the nutritional insult. For example, historical cohort studies have highlighted that metabolic outcomes due to maternal exposure to famine are entirely based upon the trimester of exposure(Reference Ravelli, Stein and Susser7, Reference Ravelli, van Der Meulen and Osmond8).
Many studies(Reference Petrik, Reusens and Arany9–Reference Sohi, Marchand and Revesz12) have focused on individual dietary components in order to determine if the observed changes in phenotype can be attributed to reduced levels of a particular macronutrient; where offspring of protein-restricted dams develop hypertension, impaired glucose tolerance and insulin resistance, and have impaired liver function. Although both low-protein effects(Reference Zambrano, Bautista and Deas10) and maternal undernutrition midway through gestation(Reference Desai, Babu and Ross13) have demonstrated outcomes that were dependent upon discrete critical periods of development, few studies have investigated the specific windows of development in a global maternal undernutrition model.
It is now well established that bone health and bone disorders also have their origins in utero (Reference Schlussel, Dos Santos Vaz and Kac14, Reference Cooper, Harvey and Cole15). Birth weight and postnatal growth are independent determinants of adult bone mass(Reference Schlussel, Dos Santos Vaz and Kac14, Reference Saito, Nakamura and Okuda16); and maternal nutritional history influences bone development and adult bone morphology(Reference Saito, Nakamura and Okuda16–Reference Jones, Riley and Dwyer19). Recent experimental models of maternal low protein(Reference Fetoui, Mahjoubi-Samet and Guermazi20, Reference Lanham, Roberts and Cooper21), placental insufficiency(Reference Romano, Wark and Owens22) and streptozotocin-induced diabetes(Reference Zhao and Weiler23) all demonstrate that early-life nutritional insults significantly impact on bone development in childhood and- adult bone health, although only few studies have identified that there may be critical periods of vulnerability(Reference Zambrano, Bautista and Deas10, Reference Romano, Wark and Owens22) and changes in offspring bone composition following different periods of maternal undernutrition have not previously been examined.
Therefore, the present study investigated the effect of moderate maternal global undernutrition during pregnancy and/or lactation to examine changes in body composition and bone health and postnatal metabolic sequelae in male and female offspring following well-defined periods of early-life nutrient deprivation. We hypothesised that the nature of the phenotype; offspring growth, metabolic indices and bone health, would be dependent upon the timing of the insult and that energy restriction during pregnancy and lactation would have the most detrimental effect.
Materials and methods
Female Wistar rats (120 d of age (n 24)) were time-mated using an oestrus cycle monitor (Fine Science Tools, Foster City, CA, USA). Upon confirmation of mating, four maternal dietary groups were established: (1) controls (CONT): females maintained on a standard chow diet ad libitum throughout pregnancy and lactation; (2) females fed at 50 % of CONT throughout pregnancy and then fed ad libitum throughout lactation (UNP); (3) females fed ad libitum throughout pregnancy and then fed at 50 % of CONT throughout lactation (UNL); (4) females fed at 50 % of CONT throughout pregnancy and lactation (UNPL). All pregnant dams were weighed and had food intakes measured daily throughout pregnancy. Following birth, pups were weighed, had body lengths recorded and litter size was randomly adjusted to eight pups (four males and four females) to ensure standardised nutrition until weaning. Non-assigned pups were killed by decapitation. At birth, UNP dams were fed a standard chow diet throughout lactation. Lactating dams had body weights and food intakes measured throughout the lactation period and pups were weighed every 3 d until weaning.
After weaning, dams were fasted overnight and killed by decapitation following anaesthesia with sodium pentobarbitone (60 mg/kg, intraperitoneally) and plasma samples collected for insulin and leptin analyses. At weaning (day 22), male and female offspring were housed, two per cage (two per litter/sex/maternal background) and fed the standard chow diet ad libitum until the end of the trial (day 160). At postnatal day 150, animals (ten to twelve per group) had body composition quantified by dual energy X-ray absorptiometry (DEXA) using dedicated small animal software (GE Lunar Prodigy, Waltham, MA, USA) scanning while under light isoflurane (2 %) anaesthesia. At postnatal day 160, animals were fasted overnight and killed by decapitation following anaesthesia with sodium pentobarbitone (60 mg/kg, intraperitoneally). Tissues (liver, heart, kidneys, spleen, gonads, adrenals, brain and retroperitoneal fat pad) were weighed and blood was collected into heparinised vacutainers, centrifuged and plasma supernatant stored for future analysis. All animal experiments were approved under guidelines of the Animal Ethics Committee at the University of Auckland (R402).
Plasma analyses
Fasting leptin and insulin levels in dams and offspring were analysed using commercial rat-specific ELISA (catalogue nos. 90 040 and 90 060, respectively, CrystalChem, Downers Grover, IL, USA). Fasting plasma glucose levels were measured using a glucose meter at the time of cull (Roche AccuChek, Roche Diagnostics, Auckland, New Zealand). Blood biochemistry panels were performed using an automated biochemistry analyser (Hitachi 902 Autoanalyser; Roche Diagnostics, Indianapolis, IN, USA). Plasma insulin:leptin ratios were calculated as a proxy for adipoinsular axis function(Reference De Schepper, Zhou and De Bock24). Plasma corticosterone concentrations were measured using MS as previously described(Reference Connor, Vickers and Cupido25).
Bone mineral content and bone mineral density
DEXA analysis has been validated as an accurate and precise method to measure bone mineral content (BMC) and bone mineral density (BMD) in the rodent(Reference Karahan, Kincaid and Lauten26, Reference Griffin, Kimble and Hopfer27). BMC and BMD were determined using a GE Lunar Prodigy DEXA scanner (GE Lunar Prodigy, Madison, WI, USA). Standard high-resolution analysis was performed using the rat whole-body regional high-resolution software. BMC represents an absolute value (g) of total BMC while BMD is a calculated value (g/cm2). Calibration using a standard phantom was performed daily and variability of repeated measurements for BMC and BMD was < 2 %.
Statistical analysis
Offspring data were analysed by two-way factorial ANOVA with maternal background and sex as factors. Data on lactating dams were analysed using one-way ANOVA with maternal dietary background as a factor. Post hoc comparisons were performed using the Fisher's protected least significance test. Analysis was performed using StatView statistical software (SAS Institute, Cary, NC, USA). All data are presented as means with their standard errors unless otherwise stated.
Results
Maternal weights
Maternal undernutrition significantly impaired maternal body weight gain throughout pregnancy in UNP and UNPL dams compared to CONT and UNL dams. Although from day 15 of pregnancy there was a slow increment in weight until birth, this was significantly less than that observed in CONT and UNL dams (Fig. 1; P < 0·05). There was no difference in gestation length across the maternal groups (data not shown). During lactation, maternal weights in UNL and UNPL dams were significantly reduced compared to the CONT and UNP groups (Table 1). During lactation, UNP dams fully recuperated the body weight lost through gestation by 10 d post-partum and the body weight matched that of CONT dams by the end of lactation (Table 1).

Fig. 1 Maternal body weights during pregnancy and lactation in CONT (–○–), UNP (–●–), UNL (–□–) and UNPL (–■–) animals. Values are means, with their standard errors represented by vertical bars (n 6–8). Maternal weight gain was significantly impaired in UNP and UNPL dams from day 5 of pregnancy (P < 0·05). Note: during pregnancy, symbols for CONT and UNL overlap and UNP and UNPL overlap. CONT, control; UNP, dams undernourished to 50 % of CONT during pregnancy; UNL, dams fed at 50 % of CONT throughout lactation; UNPL, dams fed at 50 % of CONT throughout pregnancy and lactation.
Table 1 Maternal characteristics at the end of pregnancy, lactation and plasma levels of insulin, leptin and insulin:leptin ratio at the end of lactation
(Mean values with their standard errors, n 6–8)

CONT, control; UNP, dams undernourished to 50 % of CONT during pregnancy; UNL, dams fed at 50 % of CONT throughout lactation; UNPL, dams fed at 50 % of CONT throughout pregnancy and lactation.
a,b,c Mean values with unlike superscript letters were significantly different (P < 0·05).
Offspring weights
Male and female birth-weights were significantly reduced in the UNP groups compared to CONT (P < 0·0001, Fig. 2). Weaning weights for males and females (Fig. 2) were CONT>UNP>UNL>UNPL, with females significantly lighter than males for all groups. Although UNP animals demonstrated catch-up growth between birth and weaning, they remained slightly but significantly lighter than CONT animals (Fig. 2). UNL offspring exhibited significant growth restriction and were significantly lighter compared to CONT and UNP animals. UNL and UNPL offspring were significantly growth restricted compared to CONT and UNP animals, and UNPL animals were significantly lighter than UNL offspring.

Fig. 2 Birth-weights and weaning weights (g) in (A) males and (B) females. Values are means, with their standard errors represented by vertical bars (n minimum 24). a,b,c,d Mean values with unlike letters were significantly different (P < 0·05). CONT, control; UNP, dams undernourished to 50 % of CONT during pregnancy; UNL, dams fed at 50 % of CONT throughout lactation; UNPL, dams fed at 50 % of CONT throughout pregnancy and lactation.
As adults (P160), body weights in males and females were not different between CONT and UNL/UNP animals (Table 2). UNPL offspring remained significantly lighter than all other groups; approximately 15 % lighter than CONT offspring. Adult body length (nose–anus) was significantly reduced in UNPL males and females compared to all other groups (Table 2).
Table 2 Dual energy X-ray absorptiometry bone mineral content (BMC), final body weights and nose–anus lengths in male and female offspring
(Mean values with their standard errors, n 10–12)
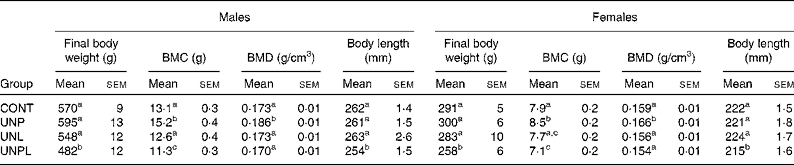
BMD, bone mineral density; CONT, control; UNP, dams undernourished to 50 % of CONT during pregnancy; UNL, dams fed at 50 % of CONT throughout lactation; UNPL, dams fed at 50 % of CONT throughout pregnancy and lactation.
a,b,c Mean values with unlike superscript letters were significantly different (P < 0·05).
Body composition
Animals underwent DEXA scanning 1 week before the completion of the trial (day 153). In males, UNP offspring had significantly increased percentage of body fat compared to all other groups (a 45 % increase over CONT, Fig. 3(A)). There were no significant differences in total body percentage fat mass between CONT and UNL or UNPL male offspring. In females, the percentage of body fat was increased in UNP offspring compared to all other groups (a 27 % increase over CONT, Fig. 3(B)). However, in contrast to males, the percentage of body fat was significantly reduced in UNL and UNPL female offspring compared to CONT offspring (25 and 24 % reductions in UNL and UNPL females compared to CONT, respectively). Overall, males had increased adiposity compared to females across all maternal dietary groups (P < 0·005 for effect of sex).

Fig. 3 Total body fat mass (%) as quantified by dual energy X-ray absorptiometry in adult (A) male and (B) female offspring. Values are means, with their standard errors represented by vertical bars (n 12–18). a,b,c Mean values with unlike letters were significantly different (P < 0·05). CONT, control; UNP, dams undernourished to 50 % of CONT during pregnancy; UNL, dams fed at 50 % of CONT throughout lactation; UNPL, dams fed at 50 % of CONT throughout pregnancy and lactation.
In males, BMC was significantly higher in UNP males compared to all other groups and was lower in UNPL males compared to the CONT, UNP and UNL groups (Table 2). In females, BMC was highest in UNP offspring and was significantly higher (P < 0·05) in CONT and UNP v. UNPL offspring and was significantly lower (P < 0·05) in UNL v. UNP offspring (Table 2). Overall, BMC was significantly higher in males compared to females for all maternal dietary groups (P < 0·005 for effect of sex). There was a highly significant positive relationship between BMC and body tissue mass (P < 0·0001 for males and females, Fig. 4), and a clear treatment effect on BMC was observed particularly in male offspring.

Fig. 4 Relationship between body tissue mass (g) as quantified by dual energy X-ray absorptiometry scan and bone mineral content (BMC) in male (R 0·87) and female (R 0·69) adult offspring. (P < 0·0001 for relationship, male v. female slopes not significant). CONT (○), control; UNP (●), dams undernourished to 50 % of CONT during pregnancy; UNL (□), dams fed at 50 % of CONT throughout lactation; UNPL (■), dams fed at 50 % of CONT throughout pregnancy and lactation.
BMD in males was slightly but significantly higher in UNP animals compared to CONT, UNL and UNPL offspring (P < 0·05, Table 2). In females, BMD was slightly but significantly increased in UNP v. CONT, UNL and UNPL offspring (P < 0·05 and P < 0·005, respectively; Table 2). BMD was significantly lower in females compared to males for all groups (P < 0·05, Table 2).
Tissue weights
Retroperitoneal fat pad weight (expressed relative to body weight) was significantly increased in UNP males compared to CONT and UNPL offspring and trending towards significance in the UNP v. UNL group (P = 0·06, Table 3). In females, retroperitoneal fat pad weight was increased in UNP animals compared to all other groups (Table 3). In contrast to males, fat pad weights were further significantly reduced in UNL and UNPL female offspring compared to CONT offspring. Relative liver weights were significantly increased in male UNP offspring compared to CONT and UNL animals but were not different between any of the female groups (Table 3). Relative spleen weights in males were significantly reduced in UNP offspring compared to all other groups. In females, spleen weights were increased in UNPL offspring compared to CONT and UNP animals (Table 3). There were no differences in adrenal weights between any of the male offspring. Adrenal weights in UNL females were increased compared to CONT and UNP animals (Table 3). Heart and kidney weights were not different between any of the male and female groups compared to all other groups (Table 3).
Table 3 Tissue weights (expressed relative to body weights (BW)) in adult male and female offspring
(Mean values with their standard errors, n 12–18)

Retro, retroperitoneal fat pad; CONT, control; UNP, dams undernourished to 50 % of CONT during pregnancy; UNL, dams fed at 50 % of CONT throughout lactation; UNPL, dams fed at 50 % of CONT throughout pregnancy and lactation.
a,b,c Mean values with unlike superscript letters were significantly different (P < 0·05).
Maternal insulin, leptin and corticosterone levels
Maternal fasting plasma insulin levels (at the end of lactation, P22) were significantly increased in UNPL dams compared to all other groups (Table 1). Maternal plasma leptin levels were significantly decreased in UNL and UNPL dams compared to CONT and UNP dams (Table 1). The maternal plasma insulin:leptin ratio (a marker of altered adipoinsular axis function(Reference De Schepper, Zhou and De Bock24)) was significantly decreased in UNP mothers compared to UNL and UNPL groups and increased in UNPL dams compared to CONT and UNP dams (Table 1).
Maternal corticosterone levels were significantly reduced in UNL and UNPL dams compared to CONT and UNP dams (Table 1).
Offspring insulin, glucose and leptin
In parallel to increased total body fat mass, fasting plasma leptin levels were significantly higher in UNP males and females compared to all other groups (Fig. 5(A) and (C). UNPL male offspring had lower plasma leptin levels than CONT male animals but were not different from UNL animals. In contrast to changes in plasma leptin levels, there was no effect of maternal dietary background on fasting plasma insulin levels across any of the male or female groups (Fig. 5(B) and (D)). Fasting plasma glucose levels were significantly increased in UNPL male offspring compared to the CONT group but were not different between other groups (CONT 5·6 (sem 0·1) mmol/l, UNP 5·7 (sem 0·4) mmol/l, UNL 5·6 (sem 0·2) mmol/l, UNPL 6·2 (sem 0·2) mmol/l). There were no differences in fasting plasma glucose levels between any of the female groups (CONT 5·7 (sem 0·2) mmol/l, UNP 5·4 (sem 0·2) mmol/l, UNL 5·4 (sem 0·2) mmol/l, UNPL 5·3 (sem 0·2) mmol/l).

Fig. 5 Fasting plasma leptin and insulin levels in adult (A and B) males and (C and D) females and (E) insulin:leptin ratios in adult male and female offspring. Values are means, with their standard errors represented by vertical bars (n 12–18). a,b,c Mean values with unlike superscript letters were significantly different (P < 0·05). CONT, control; UNP, dams undernourished to 50 % of CONT during pregnancy; UNL, dams fed at 50 % of CONT throughout lactation; UNPL, dams fed at 50 % of CONT throughout pregnancy and lactation.
Insulin:glucose ratios were significantly increased (P < 0·05) in UNPL male offspring compared to all other groups (CONT 1·8 (sem 0·2), UNP 2·6 (sem 0·3), UNL 2·5 (sem 0·2), UNPL 3·5 (sem 0·4)), indicative of reduced insulin sensitivity in UNPL offspring. Insulin:glucose ratios were not different across any of the female groups (data not shown). Plasma insulin:leptin ratios were decreased in UNP males compared to all other groups and increased in UNPL animals compared to CONT (Fig. 5(E)). In females, insulin:leptin ratios were increased in UNL and UNPL animals compared to UNP animals but were not different between the CONT and UNP groups (Fig. 5(E)).
Postnatal energy intake
Unadjusted food intake (g consumed) was not different between the treatment groups, although there was a trend (P = 0·07) towards reduced intake in UNPL offspring from weaning until postnatal day 30, at which point food intake stabilised across all groups for the remainder of the trial (data not shown). Relative energy intakes (expressed as kcal consumed/g body weight) were significantly increased in UNL and UNPL male and female offspring from weaning until approximately postnatal day 30 (average intake days 22–30: males CONT 2·05 (sem 0·08) kJ/g per d (0·49 (sem 0·02) kcal/g per d), UNP 2·17 (sem 0·12) kJ/g per d (0·52 (sem 0·03) kcal/g per d), UNL 2·42 (sem 0·16) kJ/g per d (0·58 (sem 0·04) kcal/g per d), UNPL 2·46 (sem 0·16) kJ/g per d (0·59 (sem 0·04) kcal/g per d); females: CONT 1·96 (sem 0·12) kJ/g per d (0·47 (sem 0·03) kcal/g per d), UNP 2·05 (sem 0·16) kJ/g per d (0·49 (sem 0·04) kcal/g per d), UNL 2·34 (sem 0·16) kJ/g per d (0·56 (sem 0·04) kcal/g per d), UNPL 2·30 (sem 0·16) kJ/g per d (0·55 (sem 0·04) kcal/g per d)) when daily energy intake then returned to match that of CONT and UNP offspring for the remainder of the study. There were no differences in food consumed (g) or energy intake (kcal/g) between CONT and UNP offspring at any time point measured (data not shown).
Blood biochemistry
Cholesterol/lipids
All data can be found in Table 4. Total cholesterol levels were increased in UNP and UNPL males compared to CONT offspring but were not different in UNL offspring compared to CONT males. Total plasma cholesterol levels were not altered in any of the female groups.
Table 4 Blood biochemistry data in adult male and female offspring
(Mean values with their standard errors, n 12–18)

CONT, control; UNP, dams undernourished to 50 % of CONT during pregnancy; UNL, dams fed at 50 % of CONT throughout lactation; UNPL, dams fed at 50 % of CONT throughout pregnancy and lactation; ALP, alkaline phosphatase; AST, aspartate aminotransferase; ALT, alanine transaminase.
a,b,c Mean values with unlike superscript letters were significantly different (P < 0·05).
* Cholesterol, LDL, HDL, TAG, NEFA and glycerol were represented by mmol/l.
† Lipase, ALP, AST and ALT were represented by U/l.
LDL-cholesterol levels were increased in UNP males compared to CONT but were not different in UNL and UNPL offspring compared to CONT. In females, LDL levels were higher in UNL offspring compared to UNP and UNPL offspring but there were no differences between CONT, UNP and UNPL offspring.
HDL-cholesterol levels were increased in UNP males compared to all other groups and were significantly higher in UNPL animals compared to CONT. In females, HDL levels were significantly increased in UNP and UNL offspring compared to CONT but were not different between CONT and UNPL.
LDL:HDL ratios were significantly increased in UNL males compared to CONT but were not different between any of the other groups. In females, the LDL:HDL ratio was significantly reduced in UNPL offspring compared to CONT.
NEFA levels were increased in UNP and UNPL males compared to CONT and UNL offspring. In females, NEFA were not different across any of the treatment groups. Plasma TAG levels were reduced in UNL males compared to UNP and UNPL offspring but were not different from CONT. In females, TAG were reduced in UNL offspring compared to the UNP group but were not different between other groups. Plasma lipase levels were increased in UNP males compared to CONT; and in females, the levels were increased in UNL offspring compared to all other female groups. Total free glycerol levels were increased in UNPL males compared to CONT and UNL offspring. Glycerol in females was not different across any of the treatment groups.
Plasma markers of hepatic function
All data can be found in Table 4. Alkaline phosphatase levels were increased in plasma of male UNP offspring compared to all other groups but were not different across any of the female groups. Aspartate aminotransferase levels were increased in UNP males compared to CONT and were increased in UNL offspring compared to the CONT and UNPL groups. Aspartate aminotransferase levels were not different across any of the female groups. Alanine transaminase levels were increased in UNL males compared to CONT males, and were increased in UNP females compared to all other female groups.
Discussion
Maternal undernutrition during pregnancy is well known to result in increased adiposity and related metabolic disease risk factors in offspring(Reference Vickers, Breier and Cutfield5, Reference Muhlhausler and Smith28, Reference Breier, Vickers and Ikenasio29). The present study highlights that the timing and duration of the period of undernutrition is critical to the development of the obese/metabolic phenotype and may result in changes in bone health. Extension of the period of undernutrition into lactation can partially prevent the adverse consequences associated with gestational undernutrition and, moreover, these effects are sex dependent.
In the present study, moderate maternal undernutrition restricted to pregnancy resulted in growth-restricted offspring that showed catch-up growth in weight and length paralleled by increased adiposity and increased plasma leptin levels in adulthood. Interestingly, moderate undernutrition during pregnancy alone did not have any significant effect on fasting plasma insulin or glucose levels. Compared to CONT, UNP male offspring displayed increased LDL- and HDL-cholesterol and increases in NEFA and lipase. In addition, UNP males showed increased markers of hepatic dysfunction with increased alkaline phosphatase and aspartate aminotransferase levels. In female offspring of mothers undernourished throughout pregnancy alone, the results were less marked as compared to males with only small, but significant, changes in HDL and alanine transaminase levels, with all other parameters measured being similar to those of CONT. Thus, as shown by us and others, in the presence of abundant neonatal nutrition, growth-restricted pups born to undernourished mothers demonstrate rapid catch-up growth, such that their body weights match or exceed those of CONT early in postnatal life(Reference Vickers, Breier and Cutfield5, Reference Jones, Simson and Friedman30). Thus, prevention of catch-up growth has been debated as an avenue to ameliorate the consequences of early-life growth restriction(Reference Lucas31).
Male offspring of mothers undernourished during lactation alone had total fat mass and fasting plasma insulin and leptin levels similar to those of CONT. However, there was a significant increase in LDL:HDL ratio and increases in markers of hepatic dysfunction aspartate aminotransferase and alanine transaminase. In contrast to males, UNL females had a significant reduction in total fat mass compared to both CONT and those undernourished during pregnancy alone. The reduction in fat mass was not coupled with changes in plasma insulin or leptin, although there was an increase in HDL-cholesterol and lipase. These data suggest that for females, prevention of catch-up growth in the pre-weaning period can confer lasting beneficial effects on body composition and lipid profile, independent of the level of maternal nutrition during pregnancy.
In male offspring of mothers undernourished throughout both pregnancy and lactation, total body fat mass and plasma insulin levels were similar to those of CONT although there was a small but significant reduction in plasma leptin levels. In addition, however, there were significant increases in total cholesterol (reflected by increased HDL), NEFA and glycerol in these offspring, thus still conferring a possible increased risk for cardiovascular complications in later life. Of note, there were no changes in hepatic enzymes in this group as compared to the other undernutrition groups. Similar to those females undernourished through lactation alone, female offspring of mothers undernourished throughout pregnancy and lactation had reduced total body fat mass as compared to CONT and those exposed to undernutrition during pregnancy alone. This was not reflected in altered leptin or insulin levels. As with the other plasma markers following defined windows of maternal undernutrition, females were quite distinct from males and showed a decreased LDL:HDL ratio.
Therefore, the most important result from this study is the demonstration that specific critical windows of undernutrition resulted in differential sex-specific alterations in adiposity and related blood biochemistry in adult offspring. Overall, the adverse plasma biochemistry profiles displayed by offspring of mothers undernourished through pregnancy alone were ameliorated in those offspring that continued to be undernourished into the period of lactation. Desai et al. (Reference Desai, Gayle and Babu32) showed that delayed catch-up growth in offspring with intra-uterine growth retardation was beneficial in the prevention of adult obesity, but was complicated by significant adverse effects on pancreatic function. In the present study, there were no significant changes in fasting insulin levels across the groups and only a small increase in fasting glucose levels in male UNPL offspring with no changes observed in females. This difference could relate to the timing of the nutritional challenge, with the Desai study starting the paradigm of undernutrition at mid-gestation.
With the exception of alterations in retroperitoneal fat mass, there were little marked effects of moderate maternal undernutrition on tissue weights. Retroperitoneal fat mass was increased in UNP males and females and reduced in UNL and UNPL females compared to all other groups; data which confirmed that of the DEXA data for changes in total adiposity. Maternal undernutrition has been shown to result in a reduction in kidney size and nephron number, but the lack of effect on renal mass in the present study most probably relates to a less severe level of undernutrition to that reported previously(Reference Vickers, Breier and Cutfield5, Reference Woods33, Reference Langley-Evans, Langley-Evans and Marchand34). There was a significant increase in BMC and BMD in male and female UNP offspring and this may relate to the increased adiposity and corresponding high plasma leptin levels observed in these groups(Reference Ducher, Bass and Naughton35, Reference Dennison, Syddall and Fall36). Overall, there was a highly significant relationship between BMC and body tissue weights and this agrees with previous work by others(Reference Dennison, Syddall and Fall36). Of note, these results are in disagreement with the observations of Mehta et al. (Reference Mehta, Roach and Langley-Evans37), whereby maternal low-protein exposure led to a reduction in BMC and no differences in BMD. This may relate to the different type of maternal nutritional exposures used (global undernutrition v. low-protein exposure) and the lack of programmed adiposity in the Mehta study(Reference Mehta, Roach and Langley-Evans37). Translation of our observed increases in BMC and BMD in UNP offspring to the human setting is difficult. Recent data show faster bone maturation in girls born small for gestation age (SGA) compared to CONT(Reference Ibanez, Lopez-Bermejo and Diaz38); and work by Fewtrell et al. (Reference Fewtrell, Williams and Singhal39) showed bone mass deficits only in those SGA subjects with very low birth-weight. Although altered BMC and BMD have been reported in SGA newborns(Reference Beltrand, Alison and Nicolescu40) and adolescents(Reference Leunissen, Stijnen and Boot41), respectively, data on bone mass in SGA adults are less defined. In the present study, bone data were not collected at early time points. However, since being born SGA is associated with obesity in adulthood(Reference Varvarigou42) and leptin is a known anabolic bone factor(Reference Hamrick and Ferrari43), a linkage between increased BMC and BMD in adult offspring following maternal undernutrition may not be unexpected.
Interestingly, there were marked changes in the plasma insulin:leptin ratios, useful as a marker of adipoinsular axis function(Reference De Schepper, Zhou and De Bock24). Male and female offspring undernourished during pregnancy alone had reduced insulin:leptin ratios, suggesting an impairment of leptin feedback to inhibit insulin production and adipogenesis. In contrast, male and female offspring of mothers undernourished during the period of lactation showed elevated insulin:leptin ratios, suggesting improved leptin suppression of insulin-mediated adipogenesis. This was more marked in females and thus matches the reduced adiposity seen in these groups compared to CONT and in males. However, this is a crude proxy and further molecular studies would be required to characterise specific changes in the adipoinsular feedback system.
The present study utilised a balanced experimental protocol that permitted identification of the window of exposure-dependent outcomes resulting from moderate undernutrition to pregnant and lactating rats. The data clearly show that the level of nutrition available in pregnancy and lactation plays a major role in determining offspring metabolic phenotype. The greatest effects and those most likely to be harmful to long-term function (e.g. obesity and the metabolic syndrome) occur when pups whose mothers were restricted during pregnancy received a normal – and hence abundant compared with restricted – diet during the period of lactation. Following gestational undernutrition, maintenance of the level of undernutrition into the period of lactation conferred protective effects, particularly in females, on metabolic sequalae, and further highlights the possible adverse consequences associated with catch-up growth.
Acknowledgements
The authors acknowledge the assistance of Tania Kamal for the leptin and insulin assays and Eric Thorstensen for the biochemistry profile analysis. This work was supported by the National Research Centre for Growth and Development, the Marsden Fund and the Health Research Council of New Zealand. M. H. V. designed and implemented the study with assistance from D. M. S.; G. J. H. was responsible for the experimental cohort and laboratory analysis. M. H. V. wrote the first draft of the manuscript with inputs from G. J. H. and D. M. S. The authors declare that they have no conflicts of interest.